the Creative Commons Attribution 4.0 License.
the Creative Commons Attribution 4.0 License.
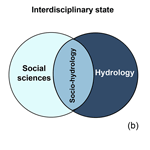
To which extent are socio-hydrology studies truly integrative? The case of natural hazards and disaster research
Franciele Maria Vanelli
Masato Kobiyama
Mariana Madruga de Brito
Given the recent developments in socio-hydrology and its potential contributions to disaster risk reduction (DRR), we conducted a systematic literature review of socio-hydrological studies aiming to identify persisting gaps and discuss tractable approaches for tackling them. A total of 44 articles that address natural hazards or disasters were reviewed in detail. Our results indicated that: (i) most of the studies addressed floods, whereas few applications were applied to droughts and compound or multi-hazard events; (ii) none of the reviewed articles investigated interactions across temporal and spatial scales; (iii) there is a wide range of understandings of what “social” means in socio-hydrology; (iv) quantitative approaches were used more often in comparison with mixed and qualitative approaches; (v) monodisciplinary studies prevailed over multi- or interdisciplinary ones; and (vi) one-third of the articles involved stakeholder participation. In summary, we observed a fragmentation in the field, with a multitude of social and physical components, methods, and data sources being used. Based on these findings, we point out potential ways of tackling the identified challenges to advance socio-hydrology, including studying multiple hazards in a joint framework and exploiting new methods for integrating results from qualitative and quantitative analyses to leverage the strengths of different fields of knowledge. Addressing these challenges will improve our understanding of human–water interactions to support DRR.
- Article
(3842 KB) -
Supplement
(748 KB) - BibTeX
- EndNote
In 2022, it will be one decade since Sivapalan et al. (2012) introduced the concept of socio-hydrology. It is also the last year of the scientific decade announced by the International Association of Hydrological Sciences (IAHS) entitled “Panta Rhei – Everything Flows” (Montanari et al., 2013). Both initiatives are associated with the growing interest in understanding the interactions between society and water. Although research on human–water interactions is not a new subject (e.g. Falkenmark, 1977, 1979), these initiatives have been fruitful in engaging researchers (Madani and Shafiee-Jood, 2020). As a result, many socio-hydrological studies have been developed in recent years, including in the areas of floods (Di Baldassarre et al., 2013; Buarque et al., 2020), droughts (Kuil et al., 2016; Medeiros and Sivapalan, 2020), groundwater (Han et al., 2017; Herrera-Franco et al., 2020), and irrigation management (Sanderson et al., 2017), among others.
Within this context, socio-hydrology is often promoted as a key approach for integrating hydrological and social sciences perspectives with the aim of providing a holistic picture of complex systems. Socio-hydrology can deal with a range of policy-relevant questions concerning natural hazards, while hydrology alone cannot address these questions as it fails to consider how anthropogenic activities affect natural hazards, and vice versa (Di Baldassarre et al., 2021). In this context, socio-hydrology can support strategies to reduce negative impacts caused by interactions between societal vulnerabilities and natural hazards (Di Baldassarre et al., 2018, 2021; Vanelli and Kobiyama, 2021). Nevertheless, although socio-hydrology can foster the integration of different knowledge types to understand coupled human–water systems, recent reviews on the topic have shown that the hydrologists' perspective still prevails (Seidl and Barthel, 2017; Xu et al., 2018). Reductionist and one-size-fits-all thinking is often used (Di Baldassarre et al., 2019). The consideration of these traditional (Hortonian) hydrology approaches departs from the Newtonian perspective of simplifying the complexity of nature to essential functions (McClain et al., 2012), whilst often assuming that quantitative approaches (positivism) are superior to qualitative ones (interpretivism) (Seidl and Barthel, 2017). Nevertheless, some valuable insights on complex human–water relations cannot be quantified or addressed solely by traditional natural sciences tools (Di Baldassarre et al., 2021; Rangecroft et al., 2021). Hence, addressing human–water interactions requires wide interdisciplinary collaboration (Seidl and Barthel, 2017; Xu et al., 2018).
The need for integrating different knowledge types is a crucial aspect of many socio-hydrology fields, especially for disaster risk reduction (DRR). Indeed, both scientific and local knowledge are required to mitigate risk and reduce the negative impacts of disasters in a comprehensive way (Rai and Khawas, 2019; Vanelli and Kobiyama, 2021). In this regard, the Sendai Framework claims that DDR should be based on understanding disaster risk in all its dimensions of vulnerability, capacity, exposure, hazard characteristics, and the environment. Hence, building disaster resilience and reducing losses requires an integrative approach and all-of-society engagement and partnership (UNDRR, 2015). Similarly, the recent report of the Intergovernmental Panel on Climate Change (Intergovernmental Panel on Climate Change, 2021) calls for multidisciplinary and transdisciplinary groups because risks can arise not only from the impacts of climate change but also from human responses to it.
Studying the complexity of natural hazards and their interactions with society thus requires us to overcome current dichotomous ways of thinking, i.e. natural sciences versus social sciences, researchers versus stakeholders, and quantitative versus qualitative. These can be addressed by integrating different disciplines (interdisciplinary), science and society (transdisciplinary), and quantitative and qualitative data and methods (mixed methods). The use of integrative approaches does not mean a “homogeneity” of the parts, but it considers that each perspective is relevant and has advantages and disadvantages. Working with the pluralism of philosophies, methodologies, backgrounds, and experiences is challenging, but it can provide new ideas, understandings, and potential solutions for complex problems (Krueger et al., 2016; Rangecroft et al., 2021; Slater and Robinson, 2020).
The main objective of this study is to investigate the current state of the art regarding socio-hydrological studies in the field of natural hazards, risks, and disasters. In particular, our research focused on evaluating the extent to which current applications are holistic in terms of considering coupled physical and social systems, as well as how they integrate different types of knowledge. To this end, a systematic literature review was conducted. The following questions guided the analysis: (i) Which disasters triggered by natural hazards are addressed in socio-hydrology studies?; (ii) How are these studies distributed across different countries and continents?; (iii) Which spatial and temporal scales are considered?; (iv) How are coupled social and physical (natural) systems illustrated or represented?; (v) Which methods are used to gather and process data?; and (vi) To which extent are these studies inter- and/or transdisciplinary? By answering these questions, we synthesized current research, identified persisting gaps, and provided possible ways forward.
To ensure objectivity, transparency, and reproducibility, the systematic review followed the ROSES reporting standards (Haddaway et al., 2018). Searches were performed on 12 February 2021 on the Web of Science (WoS) and Scopus databases. No start time constraints were used; however, only articles published until 31 December 2020 were considered. We searched for the following search terms in the title, abstract, and author's keywords: (“socio-hydrology” or “sociohydrology” or “socio-hydrological” or “sociohydrological” or “socio-hydrologic” or “sociohydrologic”). In addition, both databases were searched for terms related to hydrology in general: (“hydrology” or “hydrological” or “hydrologic”). This allowed us to normalize the data in order to correctly measure the temporal trend in the number of socio-hydrology publications. The queries were restricted to English-language and peer-reviewed articles. See Table S1 in the Supplement for more details on the search strings used.
The review process involved a set of progressive steps (Fig. 1). At first, duplicate articles (n= 189) were removed from the sample. The 231 remaining articles were screened according to the eligibility criteria shown in Table 1: first at the title and abstract level, and then on the full-text level. Given the field of expertise of the authors and that currently, there are no systematic reviews that address natural hazards, risks, and disasters, the review focused on case study applications to these fields. A total of 54 articles were retrieved for full-text analysis. Of these, five were disregarded as they were reviews, editorials, or opinion articles: Di Baldassarre et al. (2018); Borga et al. (2019); Gober and Wheater (2015); Wens et al. (2019); and Westerberg et al. (2017). Five further articles were removed from the analysis as they did not address social system components. Here, “social systems” correspond to individuals, groups, institutions, and their interactions, whereas “physical systems” refer to physical entities, processes, and their relationships. After the screening step, 44 articles were deemed relevant to the objectives of our review.
In order to answer the research questions, the articles were categorized according to the following criteria: (a) country of the study; (b) type of natural hazard investigated; (c) spatial scale of the social and physical systems; (d) temporal scale of the social and physical systems; (e) physical and social components; (f) social and physical data gathering sources; (g) social and physical data processing methods; (h) methodological approach type; and (i) inter- and transdisciplinarity. The classification followed an inductive reasoning approach and was conducted by the first author (FMV). Uncertainties were resolved through discussion between the reviewers (FMV and MMdB).
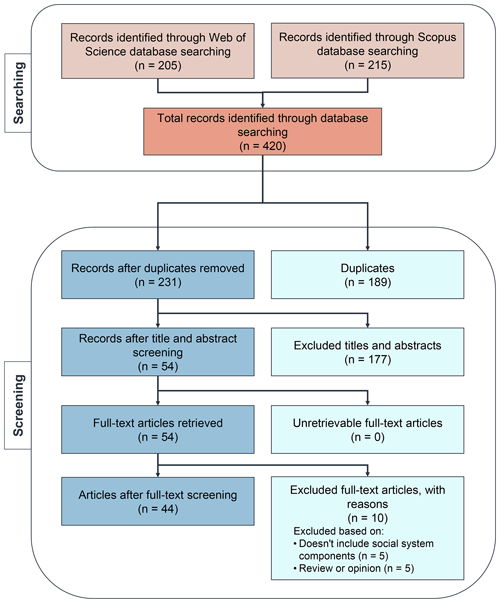
Figure 1Process used to select relevant articles adapted from the ROSES flow diagram for systematic reviews. Note that “n” indicates the number of articles in each step or item.
To classify the articles' spatial and temporal scales, we considered the scales indicated by the authors in the methods or results sections. Likewise, to classify the articles' methodological approaches, we considered the data gathering and processing techniques mentioned in the text. For instance, if an article used quantitative techniques to gather and process data, we classified it as a quantitative approach; if qualitative techniques were used, we classified it as a qualitative approach. Articles that used a mixed research design, such as qualitative techniques for data gathering (e.g. narratives or focus groups) and quantitative techniques for data processing (e.g. agent-based modelling or statistical analysis), were added to a mixed approach category. Mixed approaches are also referred to as integrative research, mixed methods research, multiple research, triangulation, and multi-strategy, among others (e.g. Di Baldassarre et al., 2021; Bryman, 2007; Creswell, 2012; Johnson and Onwuegbuzie, 2004; O'Cathain et al., 2010). In cases where the techniques used by the authors were not clear, we considered how the results were presented and analysed (qualitative or quantitative).
The interdisciplinarity of each article was classified as “monodisciplinary”, “weakly inter- or multidisciplinary”, or “inter- or multidisciplinary”. To do this, we followed previous studies (Seidl and Barthel, 2017; Xu et al., 2018) and considered the composition of the authors' disciplinary perspectives (natural or social sciences), based on their affiliations. Conversely, transdisciplinarity was classified in a binary way and was defined as studies that go beyond disciplinary boundaries and include stakeholders in the research design. To this end, we verified if stakeholders outside academia, such as citizens, decision-makers, and policymakers, were included in the study at a certain level (e.g. through filling out surveys or attending focus group discussions, among other activities).
3.1 Overview of the socio-hydrology articles
Even though some articles mentioned the term “socio-hydrology” before 2012 (e.g. Lele, 2009; Mohorjy, 1989), the first article to propose the simultaneous investigation of both social and physical components was by Sivapalan et al. (2012). To evaluate the temporal publication trends, the number of “socio-hydrology” articles published between 2012 and 2020 was normalized by the number of hydrology studies per year (Fig. 2). The results show a growing number of socio-hydrology articles in recent years, with most articles published in 2020. This growth can be explained by discussions on socio-hydrology prompted by key journals in the field. In 2015 Water Resources Research published an editorial called “Debates – Perspectives on Socio-Hydrology” (which is reflected by the first peak in article numbers), and between 2017 and 2018, a special issue titled “Socio-hydrology: Spatial And Temporal Dynamics of Coupled Human-Water Systems”. The Journal of Hydrology organized the “Virtual Special Issue on Building Socio-hydrological Resilience” between 2018 and 2019. Meanwhile, the Hydrological Sciences Journal published articles in its “Virtual Special Issue: Advancing socio-hydrology: a synthesis of coupled human–water systems across disciplines” between 2019 and 2020. It is worth mentioning that these special issues focused on socio-hydrology in general. None of them focused exclusively on disasters triggered by natural hazards.
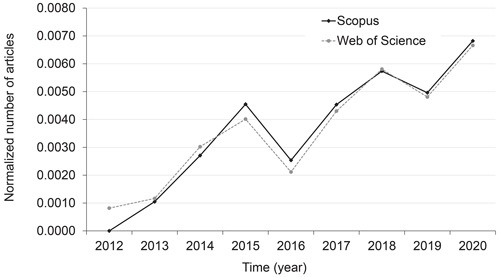
Figure 2Number of socio-hydrology articles normalized by the number of hydrology ones published between 2012 and 2020 based on data from the Web of Science (214 socio-hydrology articles and 53 175 hydrology ones) and Scopus (204 socio-hydrology articles and 50 785 hydrology ones) databases.
After the screening step, a total of 44 articles were deemed relevant and reviewed in detail. Of these, 59 % cited Sivapalan et al. (2012) when defining the concept of socio-hydrology, 41 % did not provide a definition, and none presented a new or revised concept. Hence, although researchers have used the term “socio-hydrology” more frequently in recent years, the predominance of the definition by Sivapalan et al. (2012) indicates that socio-hydrology is still a developing field.
The articles' spatial distribution (Fig. 3) shows that the studies are concentrated in just a few countries, namely Italy (n= 7) and Bangladesh (n= 6). This result is surprising because the number of studies does not correspond to the countries with the highest number of disasters between 2000 and 2019 (UNDRR and CRED, 2020). Furthermore, we did not identify many articles from the United States, Australia, and China, even though those countries generated a high frequency of studies according to a recent review on general socio-hydrology by Fischer et al. (2021).
Concerning the type of natural hazard investigated, the results show that the studies focused predominantly on floods (77.3 %). Few studies investigated droughts (11.4 %) and multi-hazards (11.4 %) (pie chart in Fig. 3). This is worrisome, as droughts cause impacts of similar magnitude to floods. Indeed, from 1900 to 2018 floods caused 4.4 million fatalities and affected 2.5 billion people. Droughts, on the other hand, killed 11.7 million people and affected 2.7 billion people (EM-Dat, 2021). Most articles did not detail the type of flood or drought investigated. Of the 34 flood articles, only 26.5 % presented details about the type of flood studied: flash flood (5.9 %); flash flood and riverine flood (2.9 %); coastal flood (5.9 %); urban flood and coastal flood (2.9 %); pluvial flood and coastal flood (2.9 %); riverine flood and coastal flood (2.9 %); and urban flood (2.9 %). None of the articles specified the type of droughts studied (i.e. meteorological drought, hydrological drought, agricultural drought). This is particularly relevant as different drought types have different implications for their management (Hagenlocher et al., 2019). Regarding multi-hazard studies, most of the articles that investigated droughts also addressed floods. Indeed, 9.1 % of the 44 articles studied floods and droughts (Albertini et al., 2020; Baeza et al., 2019; Lerner et al., 2018; Shelton et al., 2018). Moreover, Mondino et al. (2020a) analysed flood and debris flow occurring as compound hazards. Other types of natural hazards, for instance, heatwaves and earthquakes, where water is an indirect trigger and/or essential for the disaster response (Vanelli and Kobiyama, 2021), were not identified.
3.2 Trends regarding the studies' spatial scale
Different spatial scales have been used in socio-hydrological studies, with the majority (86.4 %) considering distinctive spatial scales between social and physical systems (Fig. 4). Indeed, there are clear differences between the scales used for characterizing each system (p= 0.00049, Fisher's exact test). Some studies applied more than one spatial scale for social (6.8 %) or physical (2.3 %) systems. These studies were defined as “Multiple scales” because the presence of more than one scale did not imply interactions across different scales (i.e. cross-scale). In fact, none of the reviewed articles conducted cross-scale analyses where the result of processes at one scale interacted with other processes at another scale (Soranno et al., 2014).
For the social systems, there was a preference for detailed scales. Even though there is no convention concerning which spatial scale can provide a better overview of social processes, socio-hydrology studies often need detailed information on the exposed people and communities. As such, “Individual or Household” and “Group or Community” were the most used spatial scales (36.2 %). This is similar to the findings of Moreira et al. (2021), who found that flood vulnerability studies tend to focus on the neighbourhood scale due to data availability. Studies that focus on the individual level are also popular as they enable the collection of specific behavioural information. Among the actors involved in these studies, it is important to highlight companies (Grames et al., 2019), government agents (Abebe et al., 2019), one person per household (Mondino et al., 2020a), local communities, stakeholders, and researchers (Maghsood et al., 2019). Few studies used political units as the spatial scale: “Municipal” (8.5 %), “Regional” (4.3 %), and “National” (8.5 %). This is surprising, as public policies and laws for DRR are often defined by considering political boundaries. No studies were conducted on the “Global” scale and only 4.3 % used the basic unit of hydrology, the “River basin”, to characterize social systems.
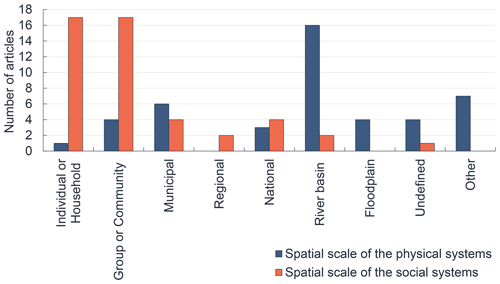
Figure 4Spatial scales used to characterize the physical and social systems in socio-hydrology studies. The total number of articles is higher than 44 as some articles used more than one scale.
For physical systems, 35.6 % of the studies used “River basin” as the spatial scale. This was expected, as the basin scale is the conventional scale used for hydrological analysis. Furthermore, as indicated previously, most of the reviewed studies dealt with floods (Fig. 3). The use of political units was infrequent: “Municipal” (13.3 %) and “National” (6.7 %). There were no studies on “Regional” or “Global” scales. Of the 44 articles, only 2.2 % used “Individual or Household” as the spatial scale for physical systems and 8.9 % used “Group or Community”. Besides this, 15.6 % of the studies relied on other spatial units: engineering structures, such as dams (Wallington and Cai, 2020) or polders (Sung et al., 2018; Yu et al., 2017), physical delimitations like groundwater (Basel et al., 2020), and floodplains (Ferdous et al., 2018, 2020; Han et al., 2020; Wang et al., 2020). One study proposed a new spatial unit: the “socio-hydrological systems boundaries”, which is defined by the outer contour of the river basins and the water supply networks (Sapountzaki and Daskalakis, 2016).
Differences were observed when comparing the scales used on different continents (Fig. 5). The traditional and common unit of hydrology, the “River basin”, was the scale most used to address physical systems in Europe, Oceania, and South America (Fig. 5a). Asian studies predominantly used “Floodplain” as the spatial scale of physical systems. For instance, Wang et al. (2020) used the “Floodplain” and defined it as the flood hazard extent with a 100-year return period. Meanwhile, Ferdous et al. (2018, 2020) and Han et al. (2020) defined the “Floodplain” extent based on previous flood events. For the social system, on all continents except Africa, most studies were conducted at the level of individuals or small groups (Fig. 5b).
3.3 Trends regarding the studies' temporal scale
The variation witnessed with regard to the spatial scales is not evident in relation to the temporal scale. Most studies (72.7 %) used the same temporal scale to address both physical and social systems (Fig. 6). For physical systems, the analyses were predominantly associated with a one-time “Extreme disaster event” (29.5 %), followed by a “Yearly” perspective (27.3 %). The “Yearly” scale predominated (50 %) for social systems and only 4.5 % of the studies investigated one-time processes by considering the “Post-disaster event” scale. Usually, the yearly temporal scale of social systems was associated with the application of data-intensive tools, such as empirical numerical modelling or agent-based modelling, resulting in a simulated sequence of data over time. Within this context, the unavailability of a time series of social data gathered in situ is a notable gap, with most studies relying on modelled data.
Some studies were classified as “Other” as they compared different temporal periods (Han et al., 2020; Nakamura and Oki, 2018) or conducted longitudinal surveys in different years (Mondino et al., 2020a). In another example, Shelton et al. (2018) considered 4 years and four rounds of analyses per year – two rounds for the dry season and two for the wet season. The temporal scale was not clearly defined in 20.5 % of the studies.
3.4 Trends regarding the social and physical components of coupled systems
To understand how coupled social and physical systems were illustrated or represented in the analysed studies, we classified the articles according to their respective components. Figure 7 shows the (a) physical and (b) social components according to the hazard investigated. Most studies (56.8 %) used more than one social component, while 25 % simplified the physical system by considering only one component. For floods, an average of 1.4 physical components and 2.4 social components was used per study, whereas for droughts the average was 1.2 for the physical components and 2.0 for the social components. Although many studies used similar components, there was a lot of variety, which is indicative of the vitality of the field. For instance, all four articles that analysed floods and droughts simultaneously applied different combinations of components (Albertini et al., 2020; Baeza et al., 2019; Lerner et al., 2018; Shelton et al., 2018). Often, the system components were not described in detail, thereby hampering the reproducibility of the results.
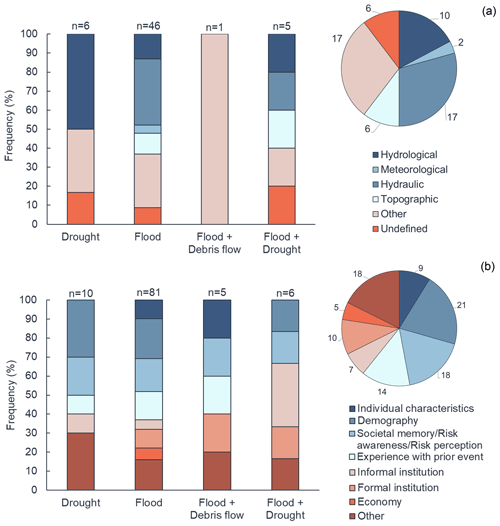
Figure 7(a) Physical components and (b) social components related to the type of natural hazard. The sum is not equal to 44 as most articles used more than one component.
For physical systems, water level, categorized here as a “Hydraulic” component, was used in 47.1 % of the studies that address floods (e.g. Di Baldassarre et al., 2017; Ciullo et al., 2017; Viglione et al., 2014). For droughts, 60 % of the studies applied a “Hydrological” component (Basel et al., 2020; Kuil et al., 2016, 2019). Several articles (38.6 %) used other physical components, such as tide level (Sung et al., 2018; Yu et al., 2017) or hazard (Ferdous et al., 2018, 2020; Leong, 2018; Mondino et al., 2020a, b). Some studies (13.6 %) did not identify the physical components in detail and were classified as “Undefined”.
Among the 54 articles that had their full text screened (Fig. 1), 5 were excluded as the social components were not considered. This indicates that the “social” in socio-hydrology has not yet been clearly defined, with divergences among authors about concepts and applications. This ambiguity in the definition of social system components was also identified by Fischer et al. (2021) in their review of socio-hydrology studies. Conversely, the physical components, such as discharge, measured in square metres per second, or water level, measured in metres, and their causal relations, are more standardized. Hence, here we will focus on the social components, aiming to provide an overview of the aspects often considered in socio-hydrological studies related to natural hazards and disasters.
Overall, the most used social component was “Demography” (47.7 %), which is used to understand people's exposure to natural hazards and the potential for population displacement. The “Informal institutions” component refers to community behaviour like local norms, rules, or attitudes, and it was used in 15.9 % of the studies. Meanwhile, “Formal institutions” (22.7 %) are based on policies, laws, or norms. “Experience with prior events” was used mainly in flood studies (27.3 %), and it was associated with the magnitude of psychological shock experienced (e.g. Di Baldassarre et al., 2013, 2017; Buarque et al., 2020; Viglione et al., 2014). In a drought study, Sapountzaki and Daskalakis (2016) investigated people's experiences with previous droughts using structured questionnaires. In a study about flood and debris flow, Mondino et al. (2020a) used “Experience”, “Risk awareness”, “Formal institution”, and “Individual characteristics” as social components.
The only social component that was applied in conjunction with all the identified types of natural hazards was “Societal memory/Risk awareness/Risk perception”. These concepts were grouped together as they are used synonymously by many authors (e.g. Michaelis et al., 2020; Sawada and Hanazaki, 2020). However, it should be highlighted that although risk awareness and risk perceptions are correlated, they are not interchangeable (Mondino et al., 2020b). Hence, even though 40.9 % of the studies used this component, the consolidation of these concepts is still lacking. Among these studies, 34.1 % calculated “Societal memory/Risk awareness/Risk perception” based on the proportion of flood damage, assuming that the individual memory is a function of disaster's exposure (e.g. Albertini et al., 2020; Di Baldassarre et al., 2013; Buarque et al., 2020).
The social component “Economy” was used by 11.4 % of the articles. For instance, Abadie et al. (2019) framed socio-hydrology as an optimization problem and included an economic valuation of costs and benefits, such as the rent on occupied land, and the costs of increasing and replacing flood defences. Sung et al. (2018) and Yu et al. (2017) considered the total annual income of a household in their socio-hydrological model. Grames et al. (2016) introduced an optimal decision framework to investigate the interaction between a society's investment in flood defence and its productive capital. In a subsequent study, Grames et al. (2019) focused on corporate decisions to invest in flood protection. In some studies, like Di Baldassarre et al. (2013), the economy was mentioned, but it was related to the growing or shrinking of human settlements in response to flooding. Such studies were therefore only included in the “Demography” category.
Many studies used “Other” social components besides the categories described above. For instance, Kuil et al. (2016) investigated the droughts that affected the Maya civilization and applied vulnerability as a social component in addition to memory and demography. Similarly, Chen et al. (2016) applied community sensitivity for studying floods in the USA, in which a higher value of sensitivity represents a greater tendency of the community to take actions favouring the environment.
3.5 Trends regarding the methods used to understand coupled social and physical systems
To investigate the most common methods used, we classified the articles according to the data gathering source and processing techniques used. It is important to highlight that we only considered the sources and methods that were explicitly mentioned by the authors. Furthermore, we attempted to adopt the same terminology used by the articles. More than 35 % of the 44 reviewed studies did not specify the sources used for gathering physical data and 25 % did not specify their social data sources (see the “Undefined” category in Fig. 8a). Of the 44 studies, 34.1 % used more than one data source for social components. For physical components this figure is 15.9 %. Although some studies used “Interviews” and “Focus groups” to collect physical data, this type of data was primarily sourced from “Gauges” and “Remote sensing”. Conversely, “Questionnaires” were the primary sources for social data gathering, followed by “Census data” and “Official documents”.
With regard to the data processing tools, empirical numerical modelling was the most used technique for both social and physical systems (Fig. 8b). This quantitative technique uses differential equations to represent the dynamic system; the resulting modelling has less detail but is intended to capture the system's holistic aspects in a general way (Sivapalan and Blöschl, 2015). An example of this socio-hydrological model is provided by Di Baldassarre et al. (2015). It is a simplification of a previous model (Di Baldassarre et al., 2013; Viglione et al., 2014) that has been applied in several studies (Di Baldassarre et al., 2017; Buarque et al., 2020; Ciullo et al., 2017; Sawada and Hanazaki, 2020) and was also modified by Abadie et al. (2019), which included the economy as a component.
“Statistical analysis” and “Agent-based modelling” were used to process social systems data in 18.2 % and 15.9 % of the studies, respectively. Few studies applied more than one technique for processing social data (Horn and Elagib, 2018; Maghsood et al., 2019; Mondino et al., 2020a; Sapountzaki and Daskalakis, 2018), and only Sawada and Hanazaki (2020) applied two techniques (empirical numerical modelling and data assimilation) for both social and physical data. Among the “Other” techniques, for instance, Leong (2018) applied the quantitative Q methodology to study the subjectivities that explain how the social memory of floods results in different vulnerability or adaptive responses.
Several data sources and techniques were used for assessing the same component. Hence, when we conducted a cluster analysis, it was not possible to identify patterns or trends in the components and methods used (see Fig. S1 in the Supplement). In one example of this diversity, Nakamura and Oki (2018) considered flood hazard and formal institutions data gathered from official documents and processed them by means of a content analysis. Koutiva et al. (2020) used similar components, but the data were obtained through “Questionnaires” and “Focus groups” and processed using an “Agent-based model”. This fragmentation and lack of guidelines about which data and methods should be used makes it difficult to compare the studies.
The classification of the data gathering sources and processing methods (Fig. 8) indicated the predominance of quantitative approaches (65.9 %) over mixed (22.7 %) and qualitative (11.4 %) ones. Although socio-hydrology was originally proposed as a quantitative field (Sivapalan et al., 2012), there are limitations to studying the human–water system solely using quantitative data and methods (Wilson et al., 2015). Our results revealed the limited integration of quantitative and qualitative data. Indeed, data analyses were often carried out separately and their findings were not combined. However mixed approaches can make a valuable contribution to a holistic understanding of interwoven social and environmental processes. Only a few of the reviewed studies used mixed approaches, such as the translation of qualitative data in agent-based models (Shelton et al., 2018) or data triangulation (Ferdous et al., 2018).
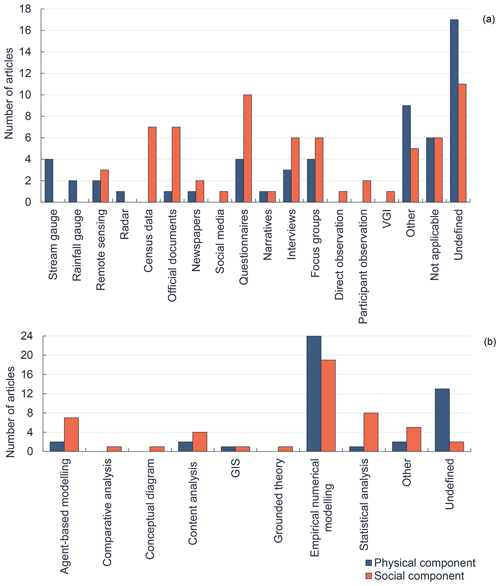
Figure 8(a) Data gathering and (b) data processing tools used for understanding the physical and social systems. The sum is not equal to 44 as most articles used more than one data source or processing technique. “Not applicable” refers to studies where data was not gathered, as they address hypothetical cases.
3.6 Trends regarding the studies' inter- and transdisciplinarity
Although disasters involve social and natural aspects, and consequently, different researchers and techniques, our review demonstrates the predominance of monodisciplinary studies (61.4 %) – mainly from natural sciences (Fig. 9a). This finding is in line with those of Seidl and Barthel (2017) and Xu et al. (2018): socio-hydrology is still dominated by hydrologists who have adopted a hegemonic attitude toward interdisciplinary collaboration with social scientists. Among the multi- or interdisciplinary studies, the working groups often involved hydrologists, physical geographers, social scientists, economists, mathematicians, and ecologists (e.g. Abadie et al., 2019; Baeza et al., 2019; Mondino et al., 2020a). It is important to highlight that these results should be interpreted with care as we considered the author's affiliation as a proxy for the study's disciplinarity. (See Sect. 5 for a discussion on this.)
Besides the infrequent use of iterative approaches among social and natural scientists, few monodisciplinary studies included stakeholder participation (33.3 %) compared with multi- or interdisciplinary studies, where stakeholder participation dominated (75 %) (Fig. 9b). This can lead to results that are not trusted – and therefore not used – by stakeholders, since they were not involved in the analysis (de Brito et al., 2017; Evers et al., 2018). In the few transdisciplinary studies we reviewed, the participation of stakeholders occurred at different levels. For instance, Mondino et al. (2020a) gathered longitudinal social data through more than 450 questionnaires in two communities. Ferdous et al. (2018) collected approximately 900 questionnaires and conducted 12 focus group discussions, each with 20 participants. Koutiva et al. (2020) used the results of questionnaires and workshops to design a model. One article (Basel et al., 2020) was even co-written by local leaders in collaboration with researchers.
In the past 5 years, there has been an upsurge in the number of socio-hydrology studies applied to natural hazards and disasters. By systematically reviewing 44 studies, we found that considerable achievements have been made. However, our results also underlined that current knowledge is limited with respect to several key areas. In this section, we summarize the challenges we have identified and propose an agenda for future research (Table 2).
The first persisting knowledge gap is related to the predominance of flood studies. Even though droughts and multi-hazard events also cause considerable damages, they have received little attention. This result can be explained by the fact that drought and multi-hazard events have complex characteristics, which make their investigation in a coupled system more challenging. For droughts, their onset, cessation, and spatial extent are notoriously problematic to determine (de Brito et al., 2020). With regard to multi-hazard events, multiple interconnections must be considered when studying them (Kappes et al., 2012). Yet, these events must be studied in the future as both droughts and compound and cascading multi-hazard events are expected to increase (AghaKouchak et al., 2018, 2020; de Brito, 2021). Besides this, all types of disasters can be considered socio-hydrological phenomena because disasters are directly and/or indirectly associated with water, which can act as the triggering agent and/or is indispensable during disaster response (Vanelli and Kobiyama, 2021). Hence, it is necessary to advance our understanding of interwoven social and environmental processes by considering the interplay between society and different types and combinations of natural hazards. To this end, new methods and data are needed to consider the dynamics between consecutive and compound hazards and society.
The second gap refers to the lack of cross-scale analyses. An investigation of the interplay across spatial scales is often advocated by socio-hydrologists (e.g. Di Baldassarre et al., 2019; Pande and Sivapalan, 2017; Sivapalan et al., 2014). This is required as the dynamic interactions between natural hazards and society share nonlinear behaviours that are driven by forces interacting across spatial and temporal scales (Adger et al., 2005; Birkmann and von Teichman, 2010; Nelson et al., 2006; Peters et al., 2004; Räsänen, 2021; Vanelli and Kobiyama, 2021). Therefore, recognizing the interactions across scales is fundamental for improving future projections, particularly in systems dominated by changing social dynamics (Srinivasan et al., 2017; York et al., 2019). Although no cross-scale studies were identified in our review, cross-scale analyses exist for applications other than natural hazards, including water resources management (York et al., 2019). We observed an overreliance on local case studies that ignore broad socio-political contexts, and vice versa. The use of static scale analysis, thus, limits our ability to understand cross-scale connections, which can in turn lead to maladaptive practices (Ford et al., 2018). In this context, the concept of “glocal” proposed by Robertson (1994) and Swyngedouw (2004) can be used to strengthen the idea of interactions and feedbacks occurring across different scales, where global connections influence the local level while local heterogeneous characteristics simultaneously influence global strategies. However, setting up such analyses is challenging due to the lack of data on human–water interactions (Brunner et al., 2021).
The third gap refers to the wide range of understandings of what `social' means in socio-hydrology. Some articles were removed during the screening stage because, even though the authors stated that they had conducted a socio-hydrological study, no social aspects were actually considered. As Basel et al. (2020) have pointed out, socio-hydrology is still developing knowledge of the variables that drives the coupled system. As such, concepts and interpretations of which social components should be considered remains contested. Such confusion makes it difficult to draw comparisons between the studies and complicates the production of cumulative insights and the identification of patterns among multiple studies (i.e. by conducting meta-analyses). Hence, there is a need for a deeper understanding of the social components and their causal relations. We suggest that scientists should be explicit about the variables they use and the reasons for doing so.
The fourth gap concerns the predominance of quantitative approaches for data gathering and processing. The use of mixed-methods research designs makes it possible to better understand the diverse social, economic, environmental, and political parts that make up natural hazards and disasters (Eriksen et al., 2011). Using different data or methods to test a hypothesis is an effective way to check its validity and reliability (Jick, 1979), because when different methods produce the same or similar results they are less likely to be artefacts (Munafò and Davey Smith, 2018). Mixed-methods approaches can enhance our confidence in the findings and be used to assess whether data agree (convergence), complement one another (complementarity), or contradict each other (O'Cathain et al., 2010). Hence, the integration of qualitative and quantitative data and methods should be used in future studies to examine socio-hydrological phenomena from multiple perspectives, as this allows us to expand or deepen our understanding of the social components in the coupled system (Di Baldassarre et al., 2021; Vanelli and Kobiyama, 2021; Wilson et al., 2015). To this end, different types of mixed research designs can be used, including simultaneous quantitative and qualitative data collection and analysis (a convergent parallel design) or the sequential collection and analysis of data (explanatory sequential design) (Creswell, 2012).
The fifth gap refers to the low frequency of inter- and transdisciplinary studies among social and natural scientists, as well as among scientists and stakeholders. Although the study of natural hazards and disasters is interdisciplinary, most of the studies we reviewed were monodisciplinary, conducted by hydrologists, and with low stakeholder participation. This raises the question for reflection: If socio-hydrology uses the same methods and perspectives as traditional hydrology, can we expect it to deliver different and new insights into complex human–water systems? Collaborative discussions and research between the social and natural sciences can significantly enhance the way research is designed and carried out, as well as produce holistic outputs (Carr et al., 2020; Rangecroft et al., 2021; Thaler, 2021). By engaging in a dialogue with key players and decision-makers, we can design models and solutions that address users' needs. Furthermore, transdisciplinary development helps to improve the sense of plural perspectives, to transform empirical knowledge into actionable knowledge, and, particularly for DRR, to enhance the credibility and deployment of results (de Brito et al., 2018). Truly transdisciplinary research requires elevating the role of stakeholders to that of co-producers (Klenk et al., 2015).
The sixth gap is linked to the lack of transparency and openness in the reviewed studies. Some articles were excluded (Fig. 1) because they did not describe the social or physical component. Furthermore, a large share of them did not provide unambiguous descriptions of the temporal and spatial scale adopted and the data gathering and processing techniques used. This restricts the reproducibility and replication of the research results and goes against FAIR guiding principles for scientific data management (Wilkinson et al., 2016). Thus, future studies should create reproducible workflows and reduce vague or incomplete reporting of the methodology used. Otherwise, science falls short of communicating results effectively. Although this gap commonly can be observed in other sciences (Nosek et al., 2015; Nüst and Pebesma, 2021), it is important to raise this discussion in the socio-hydrology community.
The last gap refers to ethical considerations about social data management (Flint et al., 2017), power dynamics, and researcher positionality in fieldwork with participants (Rangecroft et al., 2021). These topics were not mentioned in the reviewed studies. When working with human-related data, researchers must follow FAIR principles (Wilkinson et al., 2016), minimize risks to participants, obtain informed consent, and protect people's privacy (Flint et al., 2017). Privacy concerns are especially important when dealing with sensitive data about people, particularly high-resolution spatial data, consumer data, and digital trace data from social media (Zipper et al., 2019). Furthermore, there are risks for disadvantaged groups and marginalized minority populations that need to be considered (Kounadi and Leitner, 2014). Hence, socio-hydrologists need to pay more attention to the proper management of social data.
While the present study provided an inter- and transdisciplinary account of barriers in socio-hydrological research applied to natural hazards, risks, and disasters, some caveats should be considered when interpreting the obtained results. First, although we used a comprehensive set of keywords, we may have missed relevant articles during the screening process (Fig. 1). For instance, van Emmerik et al. (2014), Garcia et al. (2016), and Gonzales and Ajami (2017) address aspects related to natural hazards and awareness, which are relevant for understanding socio-hydrological phenomena. This is a common problem with any systematic review, as researchers risk missing important references given the language, search terms, and inclusion criteria used (Ivanova et al., 2020). Meanwhile, despite their drawbacks, systematic review procedures provide an in-depth and more detailed overview of the studies addressed than other techniques like systematic mapping or bibliometric analyses.
Second, we focused only on articles that mention socio-hydrology in the title, abstract, and/or author's keywords. However, studies that deal with understanding human and water interactions without mentioning socio-hydrology could contribute to a deep understanding of how these interactions are considered in disaster and risk research. In future studies, besides socio-hydrological studies related to natural hazards, risks, and disasters, human–water dynamics studies can be reviewed aiming to analyse and compare the methods used. It can be interesting to compare how human–water interactions are addressed through different lenses (e.g. nexus approach, socio-ecological system, complexity theory).
Third, there is a bias in the classification of the articles into monodisciplinary and interdisciplinary. We considered the author's affiliation as a proxy for their discipline. However, nowadays, an increasing number of researchers work in interdisciplinary projects whose affiliation department does not reflect their expertise. Hence, although the present results can be a sufficient indicator of the current disciplinarity scenario, the studies' interdisciplinarity should be investigated when possible. This could be done by, for instance, analysing the publications record of each author.
Nevertheless, despite these potential limitations, the present study is the first to present a systematic review of socio-hydrological studies applied to natural hazards, risks, and disasters. The sample of included articles provides sufficient information to stimulate discussion aiming to address challenges in this field of knowledge.
This article has provided an overview of the state of the art of socio-hydrology studies in the field of natural hazards and disaster research. The aim was to scrutinize the field's maturity in relation to different aspects. Although considerable achievements have been made during this first decade of socio-hydrology development, our systematic review revealed and reconfirmed many persisting gaps, especially regarding the degree to which current approaches are actually holistic.
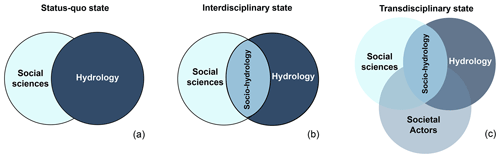
Figure 10Conceptual model of socio-hydrology: (a) status-quo state where social sciences and hydrology approaches “overlap”; (b) interdisciplinary state where social scientists and hydrologists interact; and (c) transdisciplinary state where in addition to the interaction of different disciplines, new understandings are produced by interactions with society.
We conclude that the scholarly debate on what constitutes “social” in socio-hydrology research is timely and urgent. Our results showed that hydrology has often overlapped with social sciences with no deep exchange between them and a predominance of hydrology perspectives (Fig. 10a). Notwithstanding philosophical, methodological, and communication differences, some studies did indeed apply techniques and methods from both natural and social sciences (Fig. 10b). This demonstrates that they can be used complementarily to provide a more holistic perspective on complex problems. However, the study of coupled human–water systems still has a long way to go in terms of integrating several disciplines and stakeholders. Within this context, we recommend that socio-hydrology should consider social and natural sciences knowledge equally while simultaneously involving stakeholders in order to produce new understandings (Fig. 10c). An emphasis on linking research to the practical realities of stakeholders is essential to enhance the impact of socio-hydrological studies and gain the stakeholders' trust.
Based on the identified challenges, we highlighted specific research needs that will play an important role in extending socio-hydrology in the coming years and ensuring that it can holistically address natural hazards, risks, and disasters. We expect this discussion to encourage socio-hydrologists to investigate different types of disasters using more integrative approaches that better combine the natural and social sciences, and by exploring mixed approaches, involving stakeholders, and broadening the use of cross-scale analyses. The consideration of the identified research gaps can help to strengthen socio-hydrology research and enhance its relevance to scientists, practitioners, and decision-makers to better support DRR.
The data from reviewed articles are tabulated and presented in the Supplement.
The supplement related to this article is available online at: https://doi.org/10.5194/hess-26-2301-2022-supplement.
FMV contributed to the conceptualization, data curation, investigation, methodology, and writing (original draft preparation, and review and editing). MK contributed to the project administration, supervision, and writing (review and editing). MMdB contributed to the conceptualization, methodology, supervision, and writing (review and editing).
The contact author has declared that neither they nor their co-authors have any competing interests.
Publisher's note: Copernicus Publications remains neutral with regard to jurisdictional claims in published maps and institutional affiliations.
This article is part of the special issue “Contributions of transdisciplinary approaches to hydrology and water resources management”. It is not associated with a conference.
The first author thanks the Brazilian National Council for Scientific and Technological Development (CNPq) for a research scholarship. The authors thank the referees for their relevant contributions.
This research has been supported by the Conselho Nacional de Desenvolvimento Científico e Tecnológico (grant no. 141384/2019-0).
The article processing charges for this open-access publication were covered by the Helmholtz Centre for Environmental Research – UFZ.
This paper was edited by Thomas Thaler and reviewed by Maurizio Mazzoleni and one anonymous referee.
Abadie, L. M., Markandya, A., and Neumann, M. B.: Accounting for economic factors in socio-hydrology: Optimization under uncertainty and climate change, Water-Sui., 11, 1–17, https://doi.org/10.3390/w11102073, 2019.
Abebe, Y. A., Ghorbani, A., Nikolic, I., Vojinovic, Z., and Sanchez, A.: Flood risk management in Sint Maarten – A coupled agent-based and flood modelling method, J. Environ. Manage., 248, 109317, https://doi.org/10.1016/j.jenvman.2019.109317, 2019.
Adger, W. N., Arnell, N. W., and Tompkins, E. L.: Successful adaptation to climate change across scales, Global Environ. Chang., 15, 77–86, https://doi.org/10.1016/j.gloenvcha.2004.12.005, 2005.
AghaKouchak, A., Huning, L. S., Chiang, F., Sadegh, M., Vahedifard, F., Mazdiyasni, O., Moftakhari, H., and Mallakpour, I.: How do natural hazards cascade to cause disasters?, Nature, 561, 458–460, https://doi.org/10.1038/d41586-018-06783-6, 2018.
AghaKouchak, A., Chiang, F., Huning, L. S., Love, C. A., Mallakpour, I., Mazdiyasni, O., Moftakhari, H., Papalexiou, S. M., Ragno, E., and Sadegh, M.: Climate Extremes and Compound Hazards in a Warming World, Annu. Rev. Earth Pl. Sc., 48, 519–548, https://doi.org/10.1146/annurev-earth-071719-055228, 2020.
Albertini, C., Mazzoleni, M., Totaro, V., Iacobellis, V., and Di Baldassarre, G.: Socio-Hydrological Modelling: The Influence of Reservoir Management and Societal Responses on Flood Impacts, Water, 12, 1384, https://doi.org/10.3390/w12051384, 2020.
Baeza, A., Bojorquez-Tapia, L. A., Janssen, M. A., and Eakin, H.: Operationalizing the feedback between institutional decision-making, socio-political infrastructure, and environmental risk in urban vulnerability analysis, J. Environ. Manage., 241, 407–417, https://doi.org/10.1016/j.jenvman.2019.03.138, 2019.
Di Baldassarre, G., Viglione, A., Carr, G., Kuil, L., Salinas, J. L., and Blöschl, G.: Socio-hydrology: conceptualising human-flood interactions, Hydrol. Earth Syst. Sci., 17, 3295–3303, https://doi.org/10.5194/hess-17-3295-2013, 2013.
Di Baldassarre, G., Viglione, A., Carr, G., Kuil, L., Yan, K., Brandimarte, L., and Blöschl, G.: Debates – Perspectives on socio-hydrology: Capturing feedbacks between physical and social processes, Water Resour. Res., 51, 4770–4781, https://doi.org/10.1002/2014WR016416, 2015.
Di Baldassarre, G., Saccà, S., Aronica, G. T., Grimaldi, S., Ciullo, A., and Crisci, M.: Human-flood interactions in Rome over the past 150 years, Adv. Geosci., 44, 9–13, https://doi.org/10.5194/adgeo-44-9-2017, 2017.
Di Baldassarre, G., Nohrstedt, D., Mård, J., Burchardt, S., Albin, C., Bondesson, S., Breinl, K., Deegan, F. M., Fuentes, D., Lopez, M. G., Granberg, M., Nyberg, L., Nyman, M. R., Rhodes, E., Troll, V., Young, S., Walch, C., and Parker, C. F.: An Integrative Research Framework to Unravel the Interplay of Natural Hazards and Vulnerabilities, Earth's Future, 6, 305–310, https://doi.org/10.1002/2017EF000764, 2018.
Di Baldassarre, G., Sivapalan, M., Rusca, M., Cudennec, C., Garcia, M., Kreibich, H., Konar, M., Mondino, E., Mård, J., Pande, S., Sanderson, M. R., Tian, F., Viglione, A., Wei, J., Wei, Y., Yu, D. J., Srinivasan, V., and Blöschl, G.: Sociohydrology: Scientific Challenges in Addressing the Sustainable Development Goals, Water Resour. Res., 55, 6327–6355, https://doi.org/10.1029/2018WR023901, 2019.
Di Baldassarre, G., Cloke, H., Lindersson, S., Mazzoleni, M., Mondino, E., Mård, J., Odongo, V., Raffetti, E., Ridolfi, E., Rusca, M., Savelli, E., and Tootoonchi, F.: Integrating Multiple Research Methods to Unravel the Complexity of Human-Water Systems, AGU Adv., 2, 1–6, https://doi.org/10.1029/2021av000473, 2021.
Basel, B., Hernández Quiroz, N., Velasco Herrera, R., Santiago Alonso, C., and Hoogesteger, J.: Bee mietii rak rkabni nis (The people know how to seed water): A Zapotec experience in adapting to water scarcity and drought, Clim. Dev., 13, 792–806, https://doi.org/10.1080/17565529.2020.1855100, 2020.
Birkmann, J. and von Teichman, K.: Integrating disaster risk reduction and climate change adaptation: Key challenges-scales, knowledge, and norms, Sustain. Sci., 5, 171–184, https://doi.org/10.1007/s11625-010-0108-y, 2010.
Borga, M., Comiti, F., Ruin, I., and Marra, F.: Forensic analysis of flash flood response, WIREs Water, 6, 1–9, https://doi.org/10.1002/wat2.1338, 2019.
de Brito, M. M.: Compound and cascading drought impacts do not happen by chance: A proposal to quantify their relationships, Sci. Total Environ., 778, 146236, https://doi.org/10.1016/j.scitotenv.2021.146236, 2021.
de Brito, M. M., Evers, M., and Höllermann, B.: Prioritization of flood vulnerability, coping capacity and exposure indicators through the Delphi technique: A case study in Taquari-Antas basin, Brazil, Int. J. Disast. Risk Re., 24, 119–128, https://doi.org/10.1016/j.ijdrr.2017.05.027, 2017.
de Brito, M. M., Evers, M., and Almoradie, A. D. S.: Participatory flood vulnerability assessment: a multi-criteria approach, Hydrol. Earth Syst. Sci., 22, 373–390, https://doi.org/10.5194/hess-22-373-2018, 2018.
de Brito, M. M., Kuhlicke, C., and Marx, A.: Near-real-time drought impact assessment: A text mining approach on the 2018/19 drought in Germany, Environ. Res. Lett., 15, 1040a9, https://doi.org/10.1088/1748-9326/aba4ca, 2020.
Brunner, M. I., Slater, L., Tallaksen, L. M., and Clark, M.: Challenges in modeling and predicting floods and droughts: A review, WIREs Water, 8, e1520, https://doi.org/10.1002/wat2.1520, 2021.
Bryman, A.: Barriers to Integrating Quantitative and Qualitative Research, J. Mix. Methods Res., 1, 8–22, https://doi.org/10.1177/2345678906290531, 2007.
Buarque, A. C. S., Bhattacharya-Mis, N., Fava, M. C., de Souza, F. A. A., and Mendiondo, E. M.: Using historical source data to understand urban flood risk: a socio-hydrological modelling application at Gregório Creek, Brazil, Hydrolog. Sci. J., 65, 1075–1083, https://doi.org/10.1080/02626667.2020.1740705, 2020.
Carr, G., Barendrecht, M. H., Debevec, L., Kuil, L., and Blöschl, G.: People and water: Understanding integrated systems needs integrated approaches, J. Water Supply Res. T., 69, 819–832, https://doi.org/10.2166/aqua.2020.055, 2020.
Chen, X., Wang, D., Tian, F., and Sivapalan, M.: From channelization to restoration: Sociohydrologic modeling with changing community preferences in the Kissimmee River Basin, Florida, Water Resour. Res., 52, 1227–1244, https://doi.org/10.1002/2015WR018194, 2016.
Ciullo, A., Viglione, A., Castellarin, A., Crisci, M., and Di Baldassarre, G.: Socio-hydrological modelling of flood-risk dynamics: comparing the resilience of green and technological systems, Hydrolog. Sci. J., 62, 880–891, https://doi.org/10.1080/02626667.2016.1273527, 2017.
Creswell, J.: Educational research: planning, conducting and evaluating quantitative and qualitative research, 4th ed., edited by: Pearson, Boston, ISBN-10 0-13-136739-0, ISBN-13 978-0-13-136739-5, 2012.
EM-Dat: The Emergency Events Database, https://public.emdat.be/, last access: November 2021.
Eriksen, C., Gill, N., and Bradstock, R.: Trial by fire: Natural hazards, mixed-methods and cultural research, Aust. Geogr., 42, 19–40, https://doi.org/10.1080/00049182.2011.546317, 2011.
Evers, M., Almoradie, A., and de Brito, M. M.: Enhancing Flood Resilience Through Collaborative Modelling and Multi-criteria Decision Analysis (MCDA), in: Urban Disaster Resilience and Security, The Urban Book Series, edited by: Fekete, A. and Fiedrich, F., Springer, Cham, 221–236, https://doi.org/10.1007/978-3-319-68606-6_14, 2018.
Falkenmark, M.: Water and Mankind: A Complex System of Mutual Interaction, Ambio, 6, 3–9, 1977.
Falkenmark, M.: Main Problems of Water Use and Transfer of Technology, GeoJournal, 3, 435–443, 1979.
Ferdous, M. R., Wesselink, A., Brandimarte, L., Slager, K., Zwarteveen, M., and Di Baldassarre, G.: Socio-hydrological spaces in the Jamuna River floodplain in Bangladesh, Hydrol. Earth Syst. Sci., 22, 5159–5173, https://doi.org/10.5194/hess-22-5159-2018, 2018.
Ferdous, M. R., Di Baldassarre, G., Brandimarte, L., and Wesselink, A.: The interplay between structural flood protection, population density, and flood mortality along the Jamuna River, Bangladesh, Reg. Environ. Chang., 20, 1–9, https://doi.org/10.1007/s10113-020-01600-1, 2020.
Fischer, A., Miller, J. A., Nottingham, E., Wiederstein, T., Krueger, L. J., Perez-Quesada, G., Hutchinson, S. L., and Sanderson, M. R.: A Systematic Review of Spatial-Temporal Scale Issues in Sociohydrology, Front. Water, 3, 1–19, https://doi.org/10.3389/frwa.2021.730169, 2021.
Flint, C. G., Jones, A. S., and Horsburgh, J. S.: Data Management Dimensions of Social Water Science: The iUTAH Experience, J. Am. Water Resour. Assoc., 53, 988–996, https://doi.org/10.1111/1752-1688.12568, 2017.
Ford, J. D., Pearce, T., McDowell, G., Berrang-Ford, L., Sayles, J. S., and Belfer, E.: Vulnerability and its discontents: the past, present, and future of climate change vulnerability research, Clim. Change, 151, 189–203, https://doi.org/10.1007/s10584-018-2304-1, 2018.
Garcia, M., Portney, K., and Islam, S.: A question driven socio-hydrological modeling process, Hydrol. Earth Syst. Sci., 20, 73–92, https://doi.org/10.5194/hess-20-73-2016, 2016.
Gober, P. and Wheater, H. S.: Debates – Perspectives on socio-hydrology: Modeling flood risk as a public policy problem, Water Resour. Res., 51, 4782–4788, https://doi.org/10.1002/2015WR016945, 2015.
Gonzales, P. and Ajami, N.: Social and Structural Patterns of Drought-Related Water Conservation and Rebound, Water Resour. Res., 53, 10619–10634, https://doi.org/10.1002/2017WR021852, 2017.
Gotham, K. F. and Campanella, R.: Coupled vulnerability and resilience: The dynamics of cross-scale interactions in post-Katrina new Orleans, Ecol. Soc., 16, 25, https://doi.org/10.5751/ES-04292-160312, 2011.
Grames, J., Prskawetz, A., Grass, D., Viglione, A., and Blöschl, G.: Modeling the interaction between flooding events and economic growth, Ecol. Econ., 129, 193–209, https://doi.org/10.1016/j.ecolecon.2016.06.014, 2016.
Grames, J., Grass, D., Kort, P. M., and Prskawetz, A.: Optimal investment and location decisions of a firm in a flood risk area using impulse control theory, Cent. Eur. J. Oper. Res., 27, 1051–1077, https://doi.org/10.1007/s10100-018-0532-0, 2019.
Haddaway, N. R., Macura, B., Whaley, P., and Pullin, A. S.: ROSES Reporting standards for Systematic Evidence Syntheses: Pro forma, flow-diagram and descriptive summary of the plan and conduct of environmental systematic reviews and systematic maps, Environ. Evid., 7, 7, https://doi.org/10.1186/s13750-018-0121-7, 2018.
Hagenlocher, M., Meza, I., Anderson, C. C., Min, A., Renaud, F. G., Walz, Y., Siebert, S., and Sebesvari, Z.: Drought vulnerability and risk assessments: State of the art, persistent gaps, and research agenda, Environ. Res. Lett., 14, 083002, https://doi.org/10.1088/1748-9326/ab225d, 2019.
Han, S., Tian, F., Liu, Y., and Duan, X.: Socio-hydrological perspectives of the co-evolution of humans and groundwater in Cangzhou, North China Plain, Hydrol. Earth Syst. Sci., 21, 3619–3633, https://doi.org/10.5194/hess-21-3619-2017, 2017.
Han, Y., Huang, Q., He, C., Fang, Y., Wen, J., Gao, J., and Du, S.: The growth mode of built-up land in floodplains and its impacts on flood vulnerability, Sci. Total Environ., 700, 134462, https://doi.org/10.1016/j.scitotenv.2019.134462, 2020.
Herrera-Franco, G., Carrión-Mero, P., Aguilar-Aguilar, M., Morante-Carballo, F., Jaya-Montalvo, M., and Morillo-Balsera, M. C.: Groundwater resilience assessment in a communal coastal aquifer system. The case of manglaralto in Santa Elena, Ecuador, Sustain., 12, 8290, https://doi.org/10.3390/su12198290, 2020.
Horn, F. and Elagib, N. A.: Building socio-hydrological resilient cities against flash floods: Key challenges and a practical plan for arid regions, J. Hydrol., 564, 125–132, https://doi.org/10.1016/j.jhydrol.2018.07.001, 2018.
Intergovernmental Panel on Climate Change: Assessment Report 6 Climate Change 2021: The Physical Science Basis, Contribution of Working Group I to the Sixth Assessment Report of the Intergovernmental Panel on Climate Change, edited by: Masson-Delmotte, V., Zhai, P., Pirani, A., Connors, S. L., Péan, C., Berger, S., Caud, N., Chen, Y., Goldfarb, L., Gomis, M. I., Huang, M., Leitzell, K., Lonnoy, E., Matthews, J. B. R., Maycock, T. K., Waterfield, T., Yelekçi, O., Yu, R., and Zhou, B., Cambridge University Press, https://www.ipcc.ch/report/ar6/wg1/, last access: December 2021.
Ivanova, D., Barrett, J., Wiedenhofer, D., Macura, B., Callaghan, M., and Creutzig, F.: Quantifying the potential for climate change mitigation of consumption options, Environ. Res. Lett., 15, 093001, https://doi.org/10.1088/1748-9326/ab8589, 2020.
Jick, T. D.: Mixing Qualitative and Quantitative Methods: Triangulation in Action, Adm. Sci. Q., 24, 602, https://doi.org/10.2307/2392366, 1979.
Johnson, R. B. and Onwuegbuzie, A. J.: Mixed Methods Research: A Research Paradigm Whose Time Has Come, Educ. Res., 33, 14–26, https://doi.org/10.3102/0013189X033007014, 2004.
Kappes, M. S., Keiler, M., von Elverfeldt, K., and Glade, T.: Challenges of analyzing multi-hazard risk: A review, Nat. Hazards, 64, 1925–1958, https://doi.org/10.1007/s11069-012-0294-2, 2012.
Klenk, N. L., Meehan, K., Pinel, S. L., Mendez, F., Lima, P. T., and Kammen, D. M.: Stakeholders in climate science: Beyond lip service?, Science, 350, 743–744, https://doi.org/10.1126/science.aab1495, 2015.
Kounadi, O. and Leitner, M.: Why does geoprivacy matter? The scientific publication of confidential data presented on maps, J. Empir. Res. Hum. Res., 9, 34–45, https://doi.org/10.1177/1556264614544103, 2014.
Koutiva, I., Lykou, A., Pantazis, C., and Makropoulos, C.: Investigating decision mechanisms of statutory stakeholders in flood risk strategy formation: A computational experiments approach, Water-Sui., 12, 2716, https://doi.org/10.3390/w12102716, 2020.
Krueger, T., Maynard, C., Carr, G., Bruns, A., Mueller, E. N., and Lane, S.: A transdisciplinary account of water research, WIREs Water, 3, 369–389, https://doi.org/10.1002/wat2.1132, 2016.
Kuil, L., Carr, G., Viglione, A., Prskawetz, A., and Blöschl, G.: Conceptualizing socio-hydrological drought processes: The case of the Maya collapse, Water Resour. Res., 52, 6222–6242, https://doi.org/10.1111/j.1752-1688.1969.tb04897.x, 2016.
Kuil, L., Carr, G., Prskawetz, A., Salinas, J. L., Viglione, A., and Blöschl, G.: Learning from the Ancient Maya: Exploring the Impact of Drought on Population Dynamics, Ecol. Econ., 157, 1–16, https://doi.org/10.1016/j.ecolecon.2018.10.018, 2019.
Lele, S.: Watershed services of tropical forests: from hydrology to economic valuation to integrated analysis, Curr. Opin. Sust., 1, 148–155, https://doi.org/10.1016/j.cosust.2009.10.007, 2009.
Leong, C.: The Role of Narratives in Sociohydrological Models of Flood Behaviors, Water Resour. Res., 54, 3100–3121, https://doi.org/10.1002/2017WR022036, 2018.
Lerner, A. M., Eakin, H. C., Tellman, E., Bausch, J. C., and Hernández Aguilar, B.: Governing the gaps in water governance and land-use planning in a megacity: The example of hydrological risk in Mexico City, Cities, 83, 61–70, https://doi.org/10.1016/j.cities.2018.06.009, 2018.
Madani, K. and Shafiee-Jood, M.: Socio-hydrology: A new understanding to unite or a new science to divide?, Water, 12, 1–26, https://doi.org/10.3390/w12071941, 2020.
Maghsood, F. F., Moradi, H., Berndtsson, R., Panahi, M., Daneshi, A., Hashemi, H., and Bavani, A. R. M.: Social acceptability of flood management strategies under climate change using contingent valuation method (CVM), Sustain., 11, 5053, https://doi.org/10.3390/su11185053, 2019.
McClain, M. E., Chícharo, L., Fohrer, N., Gaviño Novillo, M., Windhorst, W., and Zalewski, M.: Training hydrologists to be ecohydrologists and play a leading role in environmental problem solving, Hydrol. Earth Syst. Sci., 16, 1685–1696, https://doi.org/10.5194/hess-16-1685-2012, 2012.
Medeiros, P. and Sivapalan, M.: From hard-path to soft-path solutions: slow–fast dynamics of human adaptation to droughts in a water scarce environment, Hydrolog. Sci. J., 65, 1803–1814, https://doi.org/10.1080/02626667.2020.1770258, 2020.
Michaelis, T., Brandimarte, L., and Mazzoleni, M.: Capturing flood-risk dynamics with a coupled agent-based and hydraulic modelling framework, Hydrol. Sci. J., 65, 1458–1473, https://doi.org/10.1080/02626667.2020.1750617, 2020.
Mohorjy, A. M.: Multidisciplinary Planning and Managing of Water Reuse, JAWRA J. Am. Water Resour. Assoc., 25, 433–442, https://doi.org/10.1111/j.1752-1688.1989.tb03080.x, 1989.
Mondino, E., Scolobig, A., Borga, M., Albrecht, F., Mård, J., Weyrich, P., and Di Baldassarre, G.: Exploring changes in hydrogeological risk awareness and preparedness over time: a case study in northeastern Italy, Hydrolog. Sci. J., 65, 1049–1059, https://doi.org/10.1080/02626667.2020.1729361, 2020a.
Mondino, E., Scolobig, A., Borga, M., and Di Baldassarre, G.: The role of experience and different sources of knowledge in shaping flood risk awareness, Water-Sui., 12, 2130, https://doi.org/10.3390/W12082130, 2020b.
Montanari, A., Young, G., Savenije, H. H. G., Hughes, D., Wagener, T., Ren, L. L., Koutsoyiannis, D., Cudennec, C., Toth, E., Grimaldi, S., Bloeschl, G., Sivapalan, M., Beven, K., Gupta, H., Hipsey, M., Schaefli, B., Arheimer, B., Boegh, E., Schymanski, S. J., Di Baldassarre, G., Yu, B., Hubert, P., Huang, Y., Schumann, A., Post, D. A., Srinivasan, V., Harman, C., Thompson, S., Rogger, M., Viglione, A., McMillan, H., Characklis, G., Pang, Z., and Belyaev, V.: “Panta Rhei-Everything Flows”: Change in hydrology and society-The IAHS Scientific Decade 2013-2022, Hydrolog. Sci. J., 58, 1256–1275, https://doi.org/10.1080/02626667.2013.809088, 2013.
Moreira, L. L., de Brito, M. M., and Kobiyama, M.: Review article: A systematic review and future prospects of flood vulnerability indices, Nat. Hazards Earth Syst. Sci., 21, 1513–1530, https://doi.org/10.5194/nhess-21-1513-2021, 2021.
Munafò, M. R. and Davey Smith, G.: Robust research needs many lines of evidence, Nature, 553, 399–401, https://doi.org/10.1038/d41586-018-01023-3, 2018.
Nakamura, S. and Oki, T.: Paradigm Shifts on Flood Risk Management in Japan: Detecting Triggers of Design Flood Revisions in the Modern Era, Water Resour. Res., 54, 5504–5515, https://doi.org/10.1029/2017WR022509, 2018.
Nelson, G. C., Bennett, E., Berhe, A. A., Cassman, K., DeFries, R., Dietz, T., Dobermann, A., Dobson, A., Janetos, A., Levy, M., Marco, D., Nakicenovic, N., O'Neill, B., Norgaard, R., Petschel-Held, G., Ojima, D., Pingali, P., Watson, R., and Zurek, M.: Anthropogenic drivers of ecosystem change: An overview, Ecol. Soc., 11, 29, https://doi.org/10.5751/ES-01826-110229, 2006.
Nosek, B. A., Alter, G., Banks, G. C., Borsboom, D., Bowman, S. D., Breckler, S. J., Buck, S., Chambers, C. D., Chin, G., Christensen, G., Contestabile, M., Dafoe, A., Eich, E., Freese, J., Glennerster, R., Goroff, D., Green, D. P., Hesse, B., Humphreys, M., Ishiyama, J., Karlan, D., Kraut, A., Lupia, A., Mabry, P., Madon, T. A., Malhotra, N., Mayo-Wilson, E., McNutt, M., Miguel, E., Paluck, E. L., Simonsohn, U., Soderberg, C., Spellman, B. A., Turitto, J., VandenBos, G., Vazire, S., Wagenmakers, E. J., Wilson, R., and Yarkoni, T.: Promoting an open research culture, Science, 348, 1422–1425, https://doi.org/10.1126/science.aab2374, 2015.
Nüst, D. and Pebesma, E.: Practical Reproducibility in Geography and Geosciences, Ann. Am. Assoc. Geogr., 111, 1300–1310, https://doi.org/10.1080/24694452.2020.1806028, 2021.
O'Cathain, A., Murphy, E., and Nicholl, J.: Three techniques for integrating data in mixed methods studies, BMJ, 341, 1147–1150, https://doi.org/10.1136/bmj.c4587, 2010.
Pande, S. and Sivapalan, M.: Progress in socio-hydrology: a meta-analysis of challenges and opportunities, Wiley Interdiscip. Rev. Water, 4, e1193, https://doi.org/10.1002/wat2.1193, 2017.
Peters, D. P. C., Pielke, R. a, Bestelmeyer, B. T., Allen, C. D., Munson-mcgee, S., and Havstad, K. M.: Cross-scale interactions, nonlinearities, and forecasting catastrophic events, P. Natl. Acad. Sci. USA, 101, 15130–15135, 2004.
Rai, P. and Khawas, V.: Traditional knowledge system in disaster risk reduction: Exploration, acknowledgement and proposition, Journal of Disaster Risk Studies, 11, a484, https://doi.org/10.4102/jamba.v11i1.484, 2019.
Rangecroft, S., Rohse, M., Banks, E. W., Day, R., Di Baldassarre, G., Frommen, T., Hayashi, Y., Höllermann, B., Lebek, K., Mondino, E., Rusca, M., Wens, M., and Van Loon, A. F.: Guiding principles for hydrologists conducting interdisciplinary research and fieldwork with participants, Hydrol. Sci. J., 66, 214–225, https://doi.org/10.1080/02626667.2020.1852241, 2021.
Räsänen, A.: Cross-scale interactions in flood risk management: A case study from Rovaniemi, Finland, Int. J. Disaster Risk Re., 57, 102185, https://doi.org/10.1016/j.ijdrr.2021.102185, 2021.
Robertson, R.: Globalisation or glocalisation?, J. Int. Commun., 1, 33–52, https://doi.org/10.1080/13216597.2012.709925, 1994.
Sanderson, M. R., Bergtold, J. S., Heier Stamm, J. L., Caldas, M. M., and Ramsey, S. M.: Bringing the “social” into sociohydrology: Conservation policy support in the Central Great Plains of Kansas, USA, Water Resour. Res., 53, 6725–6743, https://doi.org/10.1002/2017WR020659, 2017.
Sapountzaki, K. and Daskalakis, I.: Transboundary resilience: the case of social-hydrological systems facing water scarcity or drought, J. Risk Res., 19, 829–846, https://doi.org/10.1080/13669877.2015.1057202, 2016.
Sapountzaki, K. and Daskalakis, I.: Expansionary Adaptive Transformations of Socio-Hydrological Systems (SHSs): The Case of Drought in Messara Plain, Crete, Greece, Environ. Manage., 61, 819–833, https://doi.org/10.1007/s00267-018-1012-y, 2018.
Sawada, Y. and Hanazaki, R.: Socio-hydrological data assimilation: analyzing human–flood interactions by model–data integration, Hydrol. Earth Syst. Sci., 24, 4777–4791, https://doi.org/10.5194/hess-24-4777-2020, 2020.
Seidl, R. and Barthel, R.: Linking scientific disciplines: Hydrology and social sciences, J. Hydrol., 550, 441–452, https://doi.org/10.1016/j.jhydrol.2017.05.008, 2017.
Shelton, R. E., Baeza, A., Janssen, M. A., and Eakin, H.: Managing household socio-hydrological risk in Mexico city: A game to communicate and validate computational modeling with stakeholders, J. Environ. Manage., 227, 200–208, https://doi.org/10.1016/j.jenvman.2018.08.094, 2018.
Sivapalan, M. and Blöschl, G.: Time scale interactions and the coevolution of humans and water, Water Resour. Res., 51, 6988–7022, https://doi.org/10.1002/2015WR017896, 2015.
Sivapalan, M., Savenije, H. H. G., and Blöschl, G.: Socio-hydrology: A new science of people and water, Hydrol. Process., 26, 1270–1276, https://doi.org/10.1002/hyp.8426, 2012.
Sivapalan, M., Konar, M., Srinivasan, V., Chhatre, A., Wutich, A., Scott, C. A., and Wescoat, J. L.: Socio-hydrology: Use-inspired water sustainability science for the Anthropocene, Earth’s Future, 2, 225–230, https://doi.org/10.1002/2013EF000164, 2014.
Slater, K. and Robinson, J.: Social learning and transdisciplinary co-production: A social practice approach, Sustain., 12, 1–17, https://doi.org/10.3390/su12187511, 2020.
Soranno, P. A., Cheruvelil, K. S., Bissell, E. G., Bremigan, M. T., Downing, J. A., Fergus, C. E., Filstrup, C. T., Henry, E. N., Lottig, N. R., Stanley, E. H., Stow, C. A., Tan, P. N., Wagner, T., and Webster, K. E.: Cross-scale interactions: Quantifying multi-scaled cause-effect relationships in macrosystems, Front. Ecol. Environ., 12, 65–73, https://doi.org/10.1890/120366, 2014.
Srinivasan, V., Sanderson, M., Garcia, M., Konar, M., Blöschl, G., and Sivapalan, M.: Prediction in a socio-hydrological world, Hydrolog. Sci. J., 62, 338–345, https://doi.org/10.1080/02626667.2016.1253844, 2017.
Sung, K., Jeong, H., Sangwan, N., and Yu, D. J.: Effects of Flood Control Strategies on Flood Resilience Under Sociohydrological Disturbances, Water Resour. Res., 54, 2661–2680, https://doi.org/10.1002/2017WR021440, 2018.
Swyngedouw, E.: Globalisation or “glocalisation”? Networks, territories and rescaling, Camb. Rev. Int. Aff., 17, 25–48, https://doi.org/10.1080/0955757042000203632, 2004.
Thaler, T.: Social justice in socio-hydrology – how we can integrate the two different perspectives, Hydrolog. Sci. J., 66, 1503–1512, https://doi.org/10.1080/02626667.2021.1950916, 2021.
United Nations for Disaster Risk Reduction (UNDRR): Sendai Framework for Disaster Risk Reduction 2015–2030, Geneva, Switzerland, https://www.preventionweb.net/files/43291_sendaiframeworkfordrren.pdf (last access: November 2021), 2015.
United Nations for Disaster Risk Reduction (UNDRR) and Centre for Research on the Epidemiology of Disasters (CRED): Human Cost of Disasters. An overview of last 20 years 2000–2019, UNDRR, Geneva, 29 pp., https://www.preventionweb.net/files/74124_humancostofdisasters20002019reportu.pdf (last access: November 2021), 2020.
Vanelli, F. M. and Kobiyama, M.: How can socio-hydrology contribute to natural disaster risk reduction?, Hydrolog. Sci. J., 66, 1758–1766, https://doi.org/10.1080/02626667.2021.1967356, 2021.
van Emmerik, T. H. M., Li, Z., Sivapalan, M., Pande, S., Kandasamy, J., Savenije, H. H. G., Chanan, A., and Vigneswaran, S.: Socio-hydrologic modeling to understand and mediate the competition for water between agriculture development and environmental health: Murrumbidgee River basin, Australia, Hydrol. Earth Syst. Sci., 18, 4239–4259, https://doi.org/10.5194/hess-18-4239-2014, 2014.
Viglione, A., Di Baldassarre, G., Brandimarte, L., Kuil, L., Carr, G., Salinas, J. L., Scolobig, A., and Blöschl, G.: Insights from socio-hydrology modelling on dealing with flood risk – Roles of collective memory, risk-taking attitude and trust, J. Hydrol., 518, 71–82, https://doi.org/10.1016/j.jhydrol.2014.01.018, 2014.
Wallington, K. and Cai, X.: Feedback Between Reservoir Operation and Floodplain Development: Implications for Reservoir Benefits and Beneficiaries, Water Resour. Res., 56, 1–20, https://doi.org/10.1029/2019WR026610, 2020.
Wang, G., Hu, Z., Liu, Y., Zhang, G., Liu, J., Lyu, Y., Gu, Y., Huang, X., Zhang, Q., Tong, Z., Hong, C., and Liu, L.: Impact of expansion pattern of built-up land in floodplains on flood vulnerability: A case study in the North China plain area, Remote Sens., 12, 1–29, https://doi.org/10.3390/rs12193172, 2020.
Wens, M., Johnson, J. M., Zagaria, C., and Veldkamp, T. I. E.: Integrating human behavior dynamics into drought risk assessment – A sociohydrologic, agent-based approach, Wiley Interdiscip. Rev. Water, 6, e1345, https://doi.org/10.1002/wat2.1345, 2019.
Westerberg, I. K., Di Baldassarre, G., Beven, K. J., Coxon, G., and Krueger, T.: Perceptual models of uncertainty for socio-hydrological systems: a flood risk change example, Hydrolog. Sci. J., 62, 1705–1713, https://doi.org/10.1080/02626667.2017.1356926, 2017.
Wilkinson, M. D., Dumontier, M., Aalbersberg, Ij. J., Appleton, G., Axton, M., Baak, A., Blomberg, N., Boiten, J. W., da Silva Santos, L. B., Bourne, P. E., Bouwman, J., Brookes, A. J., Clark, T., Crosas, M., Dillo, I., Dumon, O., Edmunds, S., Evelo, C. T., Finkers, R., Gonzalez-Beltran, A., Gray, A. J. G., Groth, P., Goble, C., Grethe, J. S., Heringa, J., t Hoen, P. A. C., Hooft, R., Kuhn, T., Kok, R., Kok, J., Lusher, S. J., Martone, M. E., Mons, A., Packer, A. L., Persson, B., Rocca-Serra, P., Roos, M., van Schaik, R., Sansone, S. A., Schultes, E., Sengstag, T., Slater, T., Strawn, G., Swertz, M. A., Thompson, M., Van Der Lei, J., Van Mulligen, E., Velterop, J., Waagmeester, A., Wittenburg, P., Wolstencroft, K., Zhao, J., and Mons, B.: The FAIR Guiding Principles for scientific data management and stewardship, Sci. Data, 3, 1–9, https://doi.org/10.1038/sdata.2016.18, 2016.
Wilson, N. J., Todd Walter, M., and Waterhouse, J.: Indigenous knowledge of hydrologic change in the Yukon river basin: A case study of Ruby, Alaska, Arctic, 68, 93–106, https://doi.org/10.14430/arctic4459, 2015.
Xu, L., Gober, P., Wheater, H. S., and Kajikawa, Y.: Reframing socio-hydrological research to include a social science perspective, J. Hydrol., 563, 76–83, https://doi.org/10.1016/j.jhydrol.2018.05.061, 2018.
York, A. M., Sullivan, A., and Bausch, J. C.: Cross-scale interactions of socio-hydrological subsystems: Examining the frontier of common pool resource governance in Arizona, Environ. Res. Lett., 14, 125019, https://doi.org/10.1088/1748-9326/ab51be, 2019.
Yu, D. J., Sangwan, N., Sung, K., Chen, X., and Merwade, V.: Incorporating institutions and collective action into a sociohydrological model of flood resilience, Water Resour. Res., 53, 1336–1353, https://doi.org/10.1002/2016WR019746, 2017.
Zipper, S. C., Stack Whitney, K., Deines, J. M., Befus, K. M., Bhatia, U., Albers, S. J., Beecher, J., Brelsford, C., Garcia, M., Gleeson, T., O'Donnell, F., Resnik, D., and Schlager, E.: Balancing Open Science and Data Privacy in the Water Sciences, Water Resour. Res., 55, 5202–5211, https://doi.org/10.1029/2019WR025080, 2019.
socialmeans in socio-hydrology, and monodisciplinary studies prevail. We expect to encourage socio-hydrologists to investigate different disasters using a more integrative approach that combines natural and social sciences tools by involving stakeholders and broadening the use of mixed methods.