the Creative Commons Attribution 4.0 License.
the Creative Commons Attribution 4.0 License.
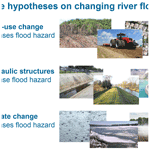
Three hypotheses on changing river flood hazards
Günter Blöschl
There is serious concern that the hazard, or probability, of river floods is increasing over time. Starting from narratives that are sometimes discussed in public, the article addresses three hypotheses. The first suggests that land-use changes, such as deforestation, urbanisation and soil compaction by agriculture, increase flood hazards. This review finds that land-use effects on floods are particularly pronounced in small catchments since soil permeability plays an important role in infiltration at this scale. For regional floods, and the most extreme events, land use is usually not the most important control, since areas of soil saturation play a greater role in runoff generation, which are less dependent on soil permeability. The second hypothesis suggests that hydraulic interventions and structures, such as river training, levees and dams, increase flood hazards. This review finds that hydraulic structures have the greatest impact on events of medium magnitude, associated with return periods of tens to hundreds of years, and that their effects are usually local. Long-term interactions between humans and floods must be taken into account when predicting future flood hazards. The third hypothesis suggests that climate change increases flood hazard. This review finds that, in small catchments of a few hectares, flood hazards may increase due to convective storms. In large catchments, where regional floods occur, changes are not necessarily directly related to precipitation, nor are they directly related to rising air temperatures, but are determined by the seasonal interplay of soil moisture, snow and extreme precipitation via runoff generation. Increases and decreases in flood hazards have been observed worldwide. It is concluded that significant progress has been made in recent years in understanding the role of land use, hydraulic structures and climate in changing river flood hazards. It is crucial to consider all three factors of change in flood risk management and communicate them to the general public in a nuanced way.
Floods are a threat to humans. Floods involve unusually high water levels that inundate the landscape in diverse settings, be it in urban areas, in mountains, at the coast or along rivers. Inundations along rivers in particular, can cause major damage to infrastructure and surprise citizens when water levels rise rapidly. The action of flowing water, sometimes combined with sediment and debris, adds to the damage. River floods are therefore one of the most costly natural hazards, both in financial terms and in loss of life (World Economic Forum, 2022). In the 20th century, river floods have caused a direct global average annual loss of USD 104 billion (UNDRR, 2015) and claimed about seven million lives (Doocy et al., 2013). It is therefore understandable that societies have always strived to reduce the likelihood of being flooded with the use of flood management instruments.
The instruments fall into two categories (Blöschl, 2017). The first comprises non-engineering (or non-structural) instruments such as evacuation (to minimise loss of life), regional planning (to ensure flood-prone areas are not developed or existing buildings are relocated to less risky areas) and flood insurance (to offset the negative economic impact of flooding). The second comprises engineering (or structural) instruments such as land-use change (e.g. afforestation to increase infiltration), flood storage (to hold back some of the flood water upstream of the point of interest), levees and mobile walls (to prevent floodplain flooding), local flood proofing (to reduce damage to buildings) and dredging (i.e. deepening the channel to increase its flood conveyance).
Both types of instruments require accurate estimates of the flood hazards, i.e. the probability that a location will be flooded in a year, or the probability that the peak runoff of a given magnitude will be exceeded in a year (The peak runoff represents the maximum volume per unit time flowing through a cross section of a stream during a flood event, measured in m s−1). There is a very good reason why accurate estimates of the flood hazards are so important. All the instruments can be implemented to different extents. Levees can be higher or lower, flood storage reservoirs can be bigger or smaller, and insurance coverage can be higher or lower. In general, the instruments are most cost effective (i.e. the reduction in the expected damage is much greater than the cost of the instruments) if the protection level matches the magnitude of the hazard, i.e. the higher the hazard, the higher the protection level. In order to minimise the resource use (e.g. financial resources, land) with the greatest possible benefit, the flood hazard must therefore be precisely known.
Hydrologists have developed a plethora of methods for estimating flood hazards that can be grouped into two types: (i) empirical approaches based on flood observations, and (ii) process-based approaches based on the drivers that control the flood magnitudes and their probabilities (Rosbjerg et al., 2013; François et al., 2019; Blöschl, 2022). In both instances, a stationarity assumption has traditionally been made, i.e. the assumption that the future will “in some ways” be similar to the past (Montanari and Koutsoyiannis, 2014). Alas, what else can we do but base our predictions of the future on what we have experienced in the past? Yet, the similarity “in some ways” does not preclude accounting for known changes (François et al., 2019). For example, the empirical approaches can involve non-stationary statistical models (Šraj et al., 2016) and the process approaches can use scenarios of a future, changed climate (Merz et al., 2014; Vorogushyn et al., 2018). The challenge then is to understand exactly which changes in the flood hazard can be expected at a particular location or in a particular context. Figure 1 illustrates how a change in the flood hazard would be reflected in observed time series of flood peak runoff and the associated frequency plots. A common indicator of the flood hazard is the 100-year flood (or equivalently, a flood with a return period of 100 years), which is the peak runoff that is exceeded with a probability of 1 % in any 1 year at a particular location along a stream.
There is growing concern that the changes in flood hazards could be substantial, not least prompted by the numerous devastating floods that have occurred in Europe and around the world in recent years, such as the flood in South Africa (April 2022), the flood in Germany with more than 200 fatalities and an event in Henan, China with more than 300 fatalities in the same month (July 2021); the flood in Bangladesh where the monsoon season was one of the worst on record (July 2020); and the floods in central Europe and in Northern India (June 2013), the latter with more than 5000 fatalities (Merz et al., 2021). This concern is an important topic in the public debate on natural disasters, both because of the threatening aspect of the recent floods, and because of the enormous dimension of resources and political decisions involved (McGrath, 2021; Matczak and Hegger, 2020). It is therefore not surprising that there is a tendency for the debate to adopt oversimplified narratives to explain the causes of disastrous floods (Pielke Jr., 2007; Merz et al., 2015). As early as 1929, the yearbook for hydrology in northern Germany, under the impression of the devastating Rhine floods of 1925 and 1926, stated: “The general public has been surprised by the major floods of the last 10 years and it is understandable that, in their search for the causes of this unusual phenomenon, they felt they had to blame the various water management measures. Almost the same concerns were expressed earlier when, after a long period of quiet, unexpectedly large floods occurred.” (LGH, 1929, p. 5).
Previous review articles (Merz et al., 2012; Hall et al., 2014; Blöschl et al., 2015) have suggested that river flood hazards may change for three main process reasons: land-use change, hydraulic structures and climate change. These are also the main factors to which increases of the flood hazard are attributed in the media and the public discussion with significant policy implications (Gavin et al., 2011). Starting from these narratives, this article therefore addresses three hypotheses (Fig. 2):
-
Land-use change increases the flood hazard.
-
Hydraulic structures increase the flood hazard.
-
Climate change increases the flood hazard.
For each of these hypotheses, I will examine experimental and modelling evidence and discuss to what extent, and under what conditions, the hypotheses are tenable. The article will therefore review recent advances in understanding how and why river floods, and their probabilities, change over time. The review is intended to guide future research and ultimately improve the efficiency and robustness of flood management instruments.
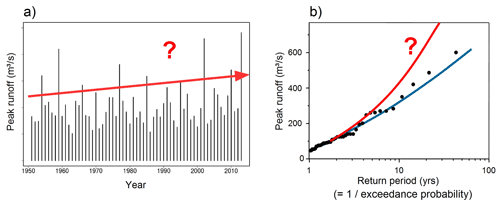
Figure 1(a) A hypothetical river flood time series. Each bar is the maximum annual peak runoff, and the red arrow indicates an increase in hazard. (b) Flood frequency plot. Each point is the maximum annual peak runoff plotted against its exceedance probability (expressed as its inverse, the return period). The fitted blue line reflects the past situation and the red line a potential increase in river flood hazards. “Hazard” is defined as the exceedance probability of a river flood level that potentially causes damage.
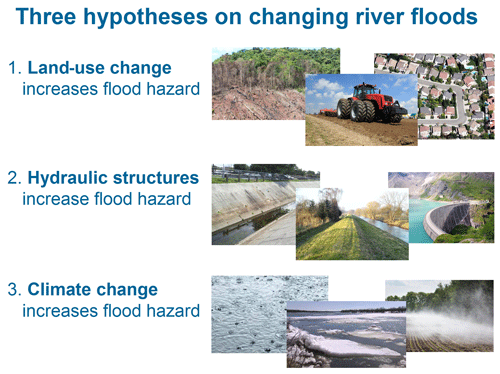
Figure 2Starting from narratives sometimes discussed by the general public, the article addresses three hypotheses: land-use changes, such as deforestation, urbanisation and soil compaction by agriculture, increase flood hazards; hydraulic structures, such as river training, levees and dams, increase flood hazards; climate change increases flood hazards through modified precipitation, snowmelt and evaporation.
Land-use change has a potentially strong impact on flooding as humans have greatly altered natural landscapes (Rogger et al., 2017). The use of heavy machinery on agricultural land tends to cause soil compaction which reduces soil infiltration, leading to increased surface runoff (Keller et al., 2019). Similarly, urbanisation tends to reduce soil infiltration, and sewage systems may shorten flow paths and thus increase flood peaks (Miller and Hutchins, 2017). Deforestation, such as clear-cutting in forest plantations, may alter soil structure and in turn reduce infiltration (Jones and Grant, 1996; Beschta et al., 2000).
Locally, at the plot scale, these processes are fairly well understood since they are amenable to experimentation. Figure 3 shows an example of where different land plots are irrigated with a known intensity of water volume per area, and the surface runoff from the plots is measured. During the experiments, there was almost no runoff from the forest plots (because all the irrigated water infiltrated), while on the grassland plots, between 30 % and 70 % of the irrigated water ran off the surface (Fig. 3b). The difference is mainly due to the higher permeability of the forest soils related to macropores, which are thin channels, e.g. created by tree roots, which enhance vertical preferential flow and thus infiltration (Gao et al., 2018). In addition, trees can absorb more water from the soil, thereby reducing soil moisture and increasing soil storage capacity (Fig. 3a) (Brown et al., 2005).
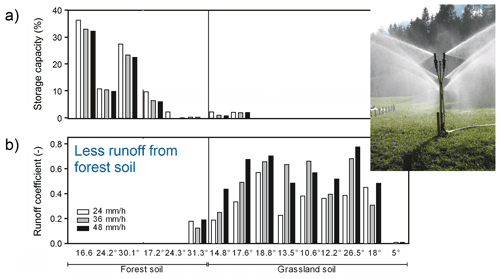
Figure 3Effect of land use on flood generation from irrigation experiments (see inset photo) in the Bernese Oberland in Switzerland. (a) Storage capacity of the soil (defined as the difference between total porosity and maximum soil moisture measured during infiltration). Each group of three bars gives the results for an experimental site. The grey shades represent different irrigation intensities. (b) Runoff coefficient (defined as the proportion of irrigation water that runs off the surface and does not infiltrate). The forest soils produce much less surface runoff than the grassland soils. Photo: Gerhard Markart (Alaoui et al., 2018).
At the catchment scale, the impacts of land-use change on floods are much less well understood due to the interaction of a number of processes involved in runoff generation (Hess et al., 2010; Rogger et al., 2017; Blöschl, 2022) and due to the inability to measure the exact spatial distribution of the relevant variables in a catchment (Blöschl et al., 2016). Paired catchment studies, where runoff from two nearby catchments (one forested and the other deforested) are compared, typically show a greater land-use effect on the seasonal water balance than on floods (Brown et al., 2005). Therefore, the usual method for assessing land-use change impacts at the catchment scale on floods is to resort to rainfall–runoff modelling (e.g. Kohnová et al., 2019). In these models, the split of rainfall into infiltration and runoff is quantified as a function of soil characteristics (such as its permeability), soil moisture and rainfall characteristics (Beven, 2011). The less permeable the soil and the higher the soil moisture before the event, the higher the runoff. In addition, the models account for evaporation from the soil and the vegetation, which reduce soil moisture.
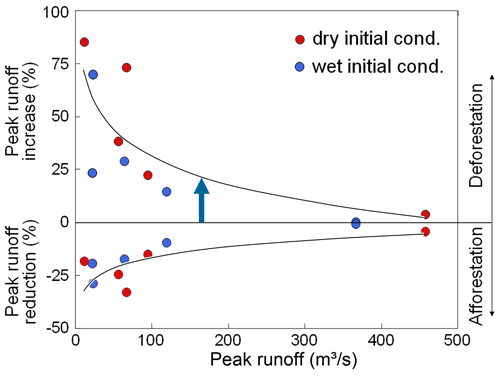
Figure 4Effect of land-use change on flood peak runoff for the 622 km2 Kamp catchment at Zwettl in Austria, using a distributed rainfall–runoff model. Upper part of the figure: increase in peak runoff of a scenario of complete deforestation relative to the observed baseline case of 47 % forested area, plotted against the peak runoff of the baseline case. Lower part of the figure: same but for afforestation (increase in forested area from 47 % to 86 %). Each point represents one event, and the initial soil moisture is shown in colour. Deforestation reduces infiltration and thus increases surface runoff. The effect decreases as the event magnitude increases. Modified from Salazar et al. (2012).
An example of such a simulation study in a 622 km2 catchment is shown in Fig. 4. The simulations show that deforestation increases surface runoff, which is mainly due to the reduced infiltration and partly to higher soil moisture. The effect is slightly larger if the soils are dry at the beginning of the event. On the other hand, afforestation decreases surface runoff but its impact depends on the event magnitude. While, for the smallest events, the peak runoff reduction is about 75 %, it is close to zero for the largest events simulated. This is because the soil becomes saturated during the largest events, so the soil structure no longer matters. This decrease of land-use change effects with event magnitude is echoed by numerous plot-scale experiments and catchment-scale simulation studies, and thus seems to be quite universal (Rogger et al., 2017). As an aside, the effect of land-use change on landslides is quite different, since the weakening of soil strength associated with deforestation may increase landslide risk for small and large rainstorms alike (Glade, 2003). As a consequence, the risk of combined landslide–flood events and debris flow events resulting from extreme rainstorms may very well be exacerbated by deforestation (Lorente et al., 2002).
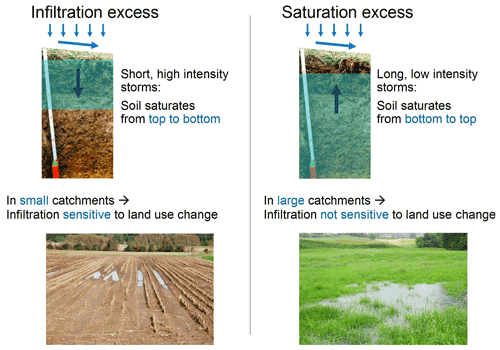
Figure 5Two runoff generation mechanisms and their impact on the sensitivity of flood runoff to land-use change. Left: The infiltration excess runoff generation mechanism often occurs in small catchments because the most critical storms tend to be short and of high intensity. In this mechanism, the soil saturates from the top to the bottom, and surface runoff is generated if the rainfall intensity exceeds the infiltration capacity. Right: The saturation excess runoff generation mechanism often occurs in large catchments because the most critical storms tend to be long and of lower intensity. In this mechanism, the soil saturates from the bottom to the top, and surface runoff is generated once the entire soil column is filled. Photos: Erwin Murer, Erwin Zehe.
One may think a catchment consists of many small plots, similar to a mosaic, hence it seems that at first glance, catchments should behave similarly to a collection of small plots, regardless of the scale of the catchment. However, this is not the case because there are very important scale effects in flood generation (Blöschl and Sivapalan, 1995; Blöschl, 2022). The main scale effect is as follows. In small catchments of a few hectares, the flood response to a rainstorm tends to be rapid, on the order of 10 min. This is the time it takes for the raindrops, or the water they push out of the soil, to reach the catchment outlet, and this time is short because of the short flow distances of a few hundred metres. Now, catchments act as filters in the sense that the largest floods are generated by storms with durations that are similar to the catchment response time, everything else (including the rainfall probability) being equal (Viglione and Blöschl, 2009). This principle is used in flood design by the Rational Method (Mulvany, 1851). In small catchments, therefore, the short storms produce the largest floods and these are storms with high-rainfall intensities, often because of their convective origin (thunderstorms). During short, high-intensity storms, the dominant runoff generation mechanism is infiltration excess (Fig. 5, left), i.e. a mechanism in which the soil starts becoming saturated from the top, and the wetting front moves down. Surface runoff is generated if the rainfall intensity (which is high) exceeds the infiltration capacity. Since the infiltration capacity is essentially determined by the soil permeability, the surface runoff produced depends heavily on the land use. In small catchments, flood runoff can therefore be expected to be quite sensitive to land-use change.
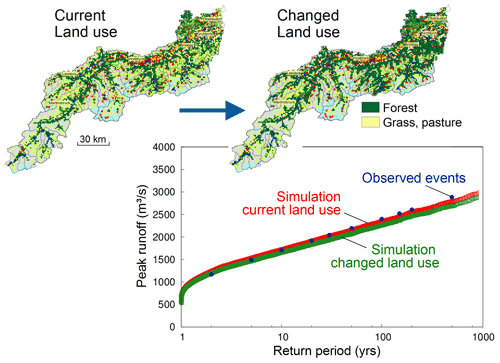
Figure 6Effects of afforestation on floods for the 9700 km2 Inn catchment at Oberaudorf in Austria using a distributed rainfall–runoff model. Considering that the catchment area is large, the relevant runoff generation mechanism is saturation excess and thus the effect of land-use change (an additional 100 000 ha of forest) is small. The flood peak runoff associated with a return period of 100 years is reduced by only 4 % (Blöschl et al., 2018).
In larger catchments, from a few to thousands of square kilometres, the situation is different. The flood response to a rainstorm tends to take longer, on the order of hours or days, because of the longer flow distances (Gaál et al., 2012), and thus longer duration, lower-intensity storms tend to be the most critical. This notion is fully in line with general experience, as the biggest floods in large river basins, such as the Danube, are never caused by local thunderstorms, but rather by regional, persistent rainfall over days (Blöschl et al., 2013). During long, lower-intensity storms, the dominant runoff generation mechanism is saturation excess (Fig. 5, right), i.e. a mechanism in which the soil starts becoming saturated from the bottom as, initially, the low-rainfall intensities can be infiltrated easily. The wetting front then moves upward from an impermeable layer in the ground. Surface runoff occurs as soon as the local groundwater table reaches the surface, i.e. surface saturation is reached. Since the depth to the impermeable layer is rarely controlled by land use, the surface runoff produced depends very little on land use. In large catchments, flood runoff can therefore be expected not to be sensitive to land-use change.
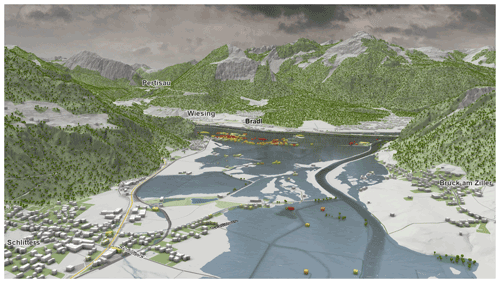
Figure 7Flood inundation modelling at hyper-resolution in Austria using a 2D hydrodynamic model at 2 m resolution nationwide. The flood-hazard zones are associated with a return period of 100 years of the peak runoff. Published by the Ministry of Agriculture, Regions and Tourism as part of the HORA flood risk zoning project on https://www.hora.gv.at (last access: 1 June 2022). (Blöschl et al., 2022).
This reasoning is based on the simulations for a 9700 km2 catchment in Fig. 6. In this study, stochastic rainfall simulations were combined with a distributed rainfall–runoff model to generate long series of peak runoff, which were analysed statistically. Two scenarios are compared, the current situation and afforestation of all the grassland below 2000 m a.s.l., which is about 11 % of the area. The reduction in the 100-year flood is only 4 % because saturation excess is the main runoff generation mechanism for the events relevant at this scale. Unlike Fig. 4, at this catchment scale, there is little dependence of the flood reduction on the return period, which is usually related to greater spatial extents of flood-producing rainstorms with increasing return period.
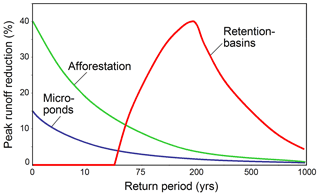
Figure 8Effect of retention basins, micro-ponds and afforestation on reducing flood peak runoff (schematic). Retention basins typically have little impact on small floods as they pass through the basins without alteration, little impact on the largest floods as the retention basins tend to fill up early in the event, and most impact on medium floods. The effect tends to be largest for small catchments that produce short flood events with small runoff volumes (Salazar et al., 2012).
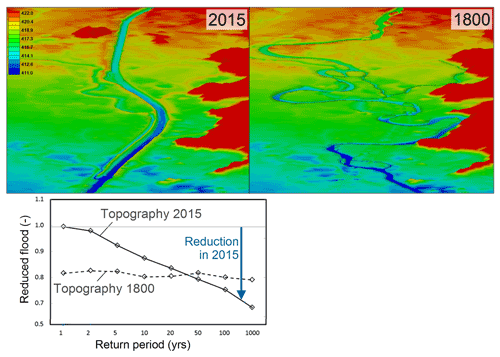
Figure 9Effect of river training on peak runoff for a 70 km reach of the Bavarian Danube between Neu-Ulm and Donauwörth using a 2D hydrodynamic model. Top left: topography of the Danube and its flood plain in 2005, reflecting the straight channel due to river training. Top right: topography in 1800, reflecting the meandering morphology of the stream. Colours are elevation (m). Bottom: Flood peak attenuation within the 70 km reach in terms of the downstream flood peak scaled by the upstream flood peak. The loss of storage volume in the flood plain between 2015 and 1800 mainly concerns small events. For example, for a return period of 1 year, the 1800 downstream peak is 82 % of the upstream peak, while the 2015 downstream peak is identical with the upstream peak. For large events, the river training has little effect on peak runoff (Skublics et al., 2016).
The effect that the works of hydraulic structures and engineering has on increasing flood hazards is fairly well understood. This is because, in some instances, their effect can simply be estimated by mass balance. In other instances, the hydrodynamic equations of moment balance need to be additionally used, for which accurate parameter-estimation methods and efficient numerical schemes exist (Horváth et al., 2020; Buttinger-Kreuzhuber et al., 2019). These techniques, along with recent advances in computing power, have enabled hyper-resolution modelling. For example, in the HORA (Hochwasserrisikozonierung Austria) project, flood-hazard zones have been estimated by running a 2D hydrodynamic model at 2 m resolution for all of Austria (Fig. 7). The main inputs to these models are the flood frequencies, at all locations along the stream network, as shown in Figs. 1b and 6.
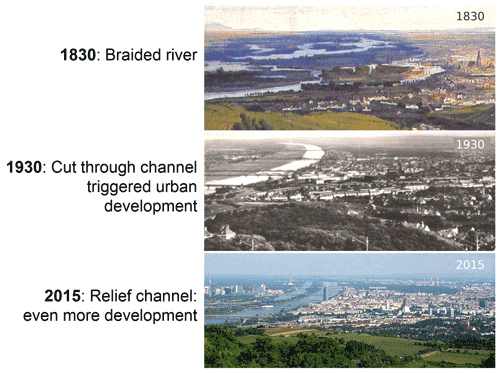
Figure 10Long-term human–flood interactions illustrated for the Danube at Vienna, Austria. In 1830, the river near Vienna was braided. As a consequence of devastating floods, a cut-through channel was constructed in 1870, as seen in the photo of 1930. The enhanced protection triggered urban development on the flood plain, which required an additional relief channel constructed in 1970, which eventually led to further urban development. The attempt of increasing protection has thus increased rather than reduced the damage potential. These unintended consequences are studied in socio-hydrology (Barendrecht et al., 2017).
Dams and the associated reservoirs on a stream are usually constructed for flood control, hydropower generation and/or water supply. These dams create online storage, i.e. during an event, part of the flood water is held back in the reservoir with the aim of reducing the peak runoff downstream of the structure. Because of this, dams rarely increase and usually decrease the downstream flood hazard. The magnitude of the reduction depends on the storage volume that can be leveraged during the flood relative to the volume of the flood wave (Wang et al., 2017; Volpi et al., 2018; Gao et al., 2019). For this reason, flood-retention basins are most effective in small catchments where the most relevant flood events tend to be short, as mentioned above, and thus the volumes are small. As the catchment scale increases, events tend to be longer and retention basins, or polders, become less and less effective. The event magnitude also plays an essential role (Fig. 8). Retention basins are usually designed in a way to bypass small floods of return periods of a few years, as these are not relevant for flood mitigation. On the other hand, very large floods will fill up the reservoir early in the event (Vorogushyn et al., 2012). At the time of the peak, the reservoir is full, so the available storage volume is zero, and the flood wave passes through the reservoir with little reduction in the flood peak runoff. The greatest reduction is thus achieved for floods with medium return periods, typically around 100 years. Furthermore, the greatest reductions are achieved immediately downstream of the dam, and the effects diminish rather quickly further downstream (Volpi et al., 2018).
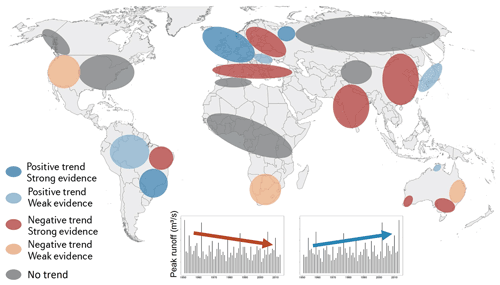
Figure 11Observed flood trends in the period of approximately 1960–2010 based on the analysis of runoff data. Blue colours indicate an increasing trend, reddish colours show a decreasing trend in mean flood peak runoff. The patterns are based on a literature meta-analysis. Globally, there are both increasing and decreasing trends of flood hazards. Merz et al. (2021).
River training, or channel straightening, by artificial cutoffs has been a widely used method, particularly in the 19th and first half of the 20th century, for reducing the frequency of overbank flooding by enlarging channel capacity (Blazejewski et al., 1995). Regarding the impact on peak runoff, two situations need to be considered separately, i.e. the local reach where the channel is straightened, and the downstream reaches. Locally, the increase in channel capacity and sometimes channel incision resulting from enhanced erosion of the river bed usually translate into less frequent floodplain inundation but little change in peak runoff (Wyzga, 1996; Erskine, 1992). Downstream, on the other hand, flood peak runoff and therefore flood hazards may increase because of the loss of flood plain storage. Analogous to the retention basin case, the magnitude of the increase depends on the storage volume that can no longer be leveraged relative to the volume of the flood wave. Conversely, allowing flood plains to inundate after river restoration works (e.g. removing levees, unstraightening rivers) will only help in proportion to the storage volume leveraged, i.e. , where , ΔVfp and Vflood are the relative peak runoff reduction, the storage volume leveraged and the runoff volume of the flood event, respectively. This means that large floodplain areas will need to be reactivated for tangible effects.
The event magnitude also plays an essential role in flood-hazard increases from loss of flood plain storage, as illustrated in Fig. 9 for a Danube reach in Bavaria. For small floods with return periods of a few years, in the historic situation of the year 1800, the flood plain gets flooded and the storage dampens the flood peak runoff. In the modern situation of 2015, the channel capacity is higher, the flood plain is no longer flooded, so the flood peak runoff is higher. For large floods with return periods of hundreds of years, however, the situation reverses. In the historic situation the flood plain is filled early during the event, so little retention takes place at the time of peak. In the modern situation, the flood plain is filled later, and significant storage takes place at the time of the peak, which reduces the peak runoff as compared to the historic situation. While river training thus tends to increase the downstream flood hazard associated with small events, it may actually reduce it for large events.
From a long-term perspective, the interactions between human decisions on building hydraulic structures and changing flood hazards are very relevant. Let us consider the case of the Danube at Vienna (Blöschl, 2014). Up to the mid-19th century, the Danube was a braided river as illustrated at the top of Fig. 10. Due to repeated floods (such as those in 1830 and 1862), the government decided to construct a cut-through channel in 1870 to increase the channel capacity and thus reduce the flood hazard in the former floodplains. The increased sense of security triggered significant urban development in the decades that followed, as illustrated in the photo from 1930. When a major flood hit the city in 1954 the damage was therefore immense, which made the government build a relief channel in the 1970s. The latter triggered even more urban development. This phenomenon of human–flood interactions is known as the levee effect (Burton et al., 1968; Viglione et al., 2014), where the attempt of increasing protection increases rather than decreases the damage potential and the flood risk. Such unintended consequences are studied in socio-hydrology (Sivapalan et al., 2012; Sivapalan and Blöschl, 2015; Barendrecht et al., 2019) with the aim of understanding changes in flood hazards more holistically.
Climate change may increase river flood hazards through altering precipitation, snowmelt and evaporation. Future changes are usually assessed on the basis of a model chain in which scenarios of projected socioeconomic global change are used to drive climate models, which produce projections of precipitation, temperature and other variables. These are used as inputs to rainfall–runoff models (Hall et al., 2014) to simulate long series of runoff. The change is then evaluated by comparing the simulated flood runoff peaks of the scenarios with simulations representing the current situation. Such simulations have been performed globally, regionally and locally (see, e.g. Do et al., 2020; Swain et al., 2020). Typically, the smaller the scale, the more detailed information on flood generation processes can be included. The meta-analysis of Merz et al. (2021) about recent regional studies around the world indicates increases in the 100-year flood peak runoff during the 21st century for much of sub-Saharan Africa, eastern and southern Asia, north-western Europe, northern Russia and some regions in South and North America. Decreases are projected for eastern Europe, south-western Russia and northern Africa.
The reliability of such projections naturally depends on the extent to which the flood-generating processes in the atmosphere, on the land and in the subsurface are captured (Blöschl, 2022). It is therefore of interest to study the climate-induced changes of the past and understand their process controls. Figure 11, again a meta-analysis, shows observed flood trends around the globe in the past 6 decades for medium-sized and large catchments. Increases have been observed in north-western Europe, parts of Japan, Amazonia and southern Brazil, while decreases have been observed in the Mediterranean, India, China, Australia, South Africa and north-eastern Brazil.
When analysing trends, the particular study period is often of crucial importance, as flood peak runoff rarely changes exactly linearly over time (Lun et al., 2020). More commonly, there are decades when floods occur more often and with larger magnitudes than usual. In these long-term fluctuations, oceans may play an important role through climate modes such as El Nino (Liu and Zhang, 2017). This is because the residence times of water and thus the memory of the oceans are on the order of decades or more. The residence times of atmospheric water, on the other hand, are only a few days, and those in catchments are months to years (Szolgayova et al, 2014; Zhang et al., 2022), so they cannot easily explain decadal or centennial flood-hazard variability. Figure 12 shows the flood-rich periods in Europe during the last 5 centuries. These periods were identified from more than 100 high-resolution historical flood series based on documentary evidence, such as chronicles, annals and legal records, covering all major regions of Europe. The most severe flood-rich period was 1760–1800 and it covered most of Europe, followed by 1840–1870 (western and southern Europe) and the recent period 1990–2016, which covered western and central Europe. The recent period, perhaps still ongoing, is thus among the most flood-rich periods in the last 500 years.
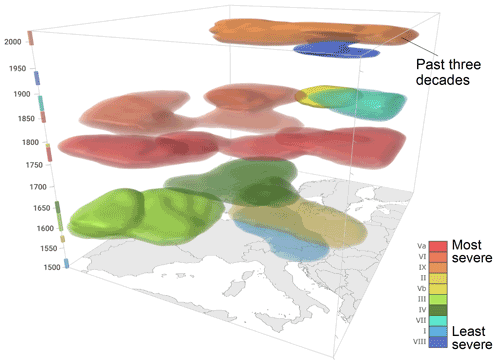
Figure 12Flood-rich periods in the last 500 years in Europe defined as periods and regions in which floods are much larger and more frequent than usual. The flood-rich periods were identified from 103 high-resolution historical flood series based on documentary evidence. The past 3 decades were among the most flood-rich periods (Blöschl et al., 2020).
For the last 6 decades, there are more detailed flood observations available in Europe which are analysed in Fig. 13 in terms of the trends in flood peak runoff for medium-sized and large catchments. There are very clear patterns of change. In north-western Europe, the median flood peak has increased by more than 5 % per decade for stations with significant changes. In the east and south there are decreases of similar magnitudes. The median flood (corresponding to a return period of 2 years) as shown Fig. 13, can be estimated relatively robustly from data, but from a flood management perspective, the hazard associated with more extreme events (such as the 100-year flood) is more relevant. Bertola et al. (2020) therefore analysed the same data set as a function of the return period. They found that, in small catchments in north-western Europe, the 100-year flood increases more than the 2-year flood, in southern Europe the 100-year flood decreases less than the median flood, and in eastern Europe the decreases depend little on the return period.
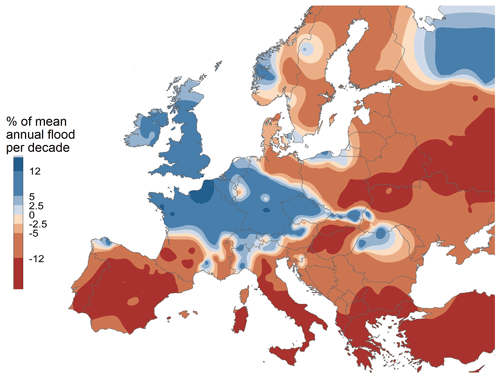
Figure 13Observed flood trends in the period 1960–2010 based on the analysis of runoff data. Blue colours indicate an increasing trend and reddish colours a decreasing trend in the median flood peak runoff. Only stations with significant trends (significance level α=0.1) were used in the spatial interpolation (664 stations) (Blöschl et al., 2019b).
There is of course an extensive literature on the role of climate change in atmospheric processes that give rise to changes in heavy precipitation (see, e.g. Field et al., 2012; Masson-Delmotte et al., 2021). In the context of flood-hazard changes, we need to again treat small and large catchments separately, similar to the effect of land use and hydraulic structures. In small catchments of a few hectares, short duration, high-intensity storms (usually of convective origin) are most relevant, and there is evidence for the intensities to increase with air temperature (Fowler et al., 2021). The rate of increase found by empirical studies often corresponds to the increasing water-holding capacity of the atmosphere (i.e. the Clausius–Clapeyron rate of ∼7 % K−1). In some regions, the rate is greater, probably because of enhanced feedbacks in convective clouds (Molnar et al., 2015; Lochbihler et al., 2017; Fowler et al., 2021). A general assessment is however difficult, because long series of high-resolution (e.g. hourly) precipitation are rather rare and not very accurate, and because summer–winter differences in heavy precipitation are sometimes interpreted to imply decadal correlations. Nevertheless, there are reasons to believe that climate change may indeed increase the flood hazard in small catchments. For large return periods, the increase may be of a similar magnitude to that of precipitation since the rainfall–runoff relationship tends to become linear, while for smaller return periods, the relative increase may be larger (Viglione et al., 2009; Breinl et al., 2021).
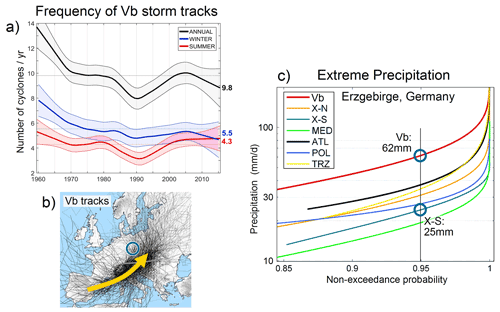
Figure 14(a) Frequency of Vb storm tracks in the period 1960–2020. (b) Vb tracks (according to Van Bebber, 1891) follow a path across the western Mediterranean into central Europe and are responsible for some of the largest floods on record, such as the August 2002 and June 2013 floods. (c) Vb storms produce much larger heavy precipitation than other track types, as illustrated by the frequency distribution of daily precipitation in the Erzgebirge region, Germany. X–S are storm types that approach from the southeast. Cyclone-track analysis based on a combination of sea level and 700 hPa pressure from JRA-55 reanalysis data (Hofstätter et al. 2016, 2018; Hofstätter and Blöschl, 2019).
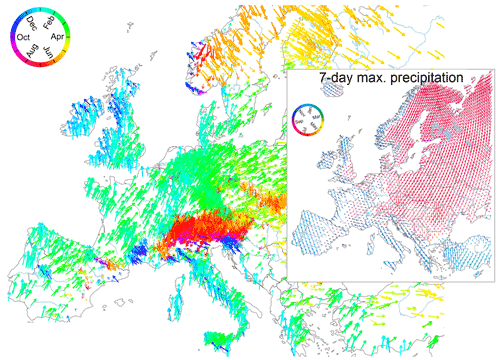
Figure 15Average timing of floods within the year to understand changes in flood hazards. Blue arrows pointing upwards indicate winter floods, red arrows show downward summer floods. Based on data from 4062 stations, 1960–2010. Inset image shows a similar plot, but for 7 d maximum annual precipitation based on the E-Obs data set. The flood seasonality differs significantly from that of precipitation, which is because of the seasonal interplay of soil moisture, snow and extreme precipitation (Blöschl et al., 2017).
In larger catchments, from a few to thousands of square kilometres, the situation is different in that convective precipitation is rarely important, but instead longer-duration, lower-intensity storms are important, so the database is better as there are many more long, high-quality records of daily precipitation than of higher-resolution precipitation (e.g. Contractor et al., 2021). In order to shed light on the driving processes, Blöschl et al. (2020) correlated European flood occurrence in the last 500 years with air temperatures and found that most of the flood-rich periods shown in Fig. 12 were around 0.3 ∘C colder than the intervals in between. This finding is in line with a tendency in summer for higher cyclonic precipitation to be associated with lower temperatures because of the enhanced cloud cover and lower solar radiation (Gagen et al., 2016), while the opposite may be true in winter (Hurrell and Van Loon, 1997). In contrast, the flood-rich period of the last 3 decades was about 1.4 ∘C warmer than usual. While, sometimes, the higher water-holding capacity of a warmer atmosphere is brought forward as a main reason of increasing floods, the different temperatures of the recent and past flood-rich periods suggest otherwise, pointing to a more important role of dynamic climate-circulation processes in modifying flood hazards in medium-sized and large catchments. Similarly, the presence of both increasing and decreasing flood peaks in Europe (Fig. 13) indicates processes quite different from an increased atmospheric water-holding capacity at that scale.
In central Europe, so called “Vb cyclones” that follow a path across the western Mediterranean into central Europe (Van Bebber, 1891), have caused major flood disasters, such as the events of August 2002 and June 2013 (Ulbrich et al., 2003; Blöschl et al., 2013). There has been a concern that the frequency of Vb cyclones may have increased in the current flood-rich period in Europe, contributing to an increase in flood hazard. However, an evaluation of atmospheric reanalysis data suggests that the frequency of Vb cyclones was high in the 1960s and has remained at a lower level since then (Fig. 14a). On the other hand, Vb cyclones do produce much larger extreme precipitation than other cyclone types. For example, in the Erzgebirge region at the Germany–Czech border, the precipitation exceeded on 5 % of the days associated with Vb storms is 62 mm d−1, while it is much lower for other storm types (Fig. 14c). The onset of Vb cyclones has been found to be related to a superposition of the polar and the subtropical jet streams over the western Mediterranean (Hofstätter and Blöschl, 2019), and it is possible that the efficiency of a given Vb cyclone to produce extreme precipitation may have increased.
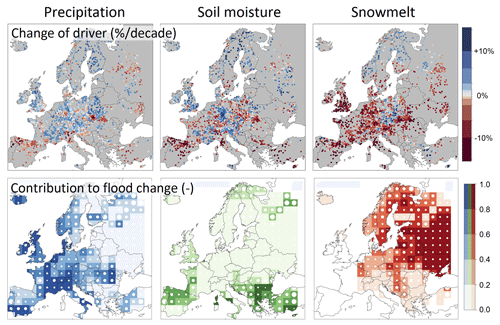
Figure 16Attribution of observed flood changes at 2370 stations in the period 1960–2010 to their climatic drivers based on Bayesian inference. Top panels: observed trends of 7 d maximum annual precipitation, soil moisture and snowmelt. Each point represents a catchment. Blue colours indicate an increasing trend, reddish colours show a decreasing trend. Bottom panels: Attributed contribution to the changes in the 100-year flood for precipitation, soil moisture and snowmelt. Strength of colour shows the relative contribution. For example, a value of 0.5 indicates that half of the observed flood trend is due to that driver. White circles indicate the reliability of the attribution, with small circles referring to a more reliable attribution (Bertola et al., 2021).
Other precipitation changes in recent years can be more clearly linked to the atmospheric circulation. The expansion of the Hadley cell (i.e. a belt of air surrounding the globe that rises along the Equator and descends at 15–30∘ N latitude) towards the North Pole, has led to a northward shift of the subtropical jet stream and associated storm tracks (Lu et al., 2007; Archer and Caldeira, 2008; Kang and Lu, 2012; Xian et al., 2021). This shift has contributed to decreasing precipitation in the south of Europe and increasing precipitation in the north, which is consistent with the flood-trend pattern of Fig. 13.
While heavy precipitation obviously plays a key role in understanding climate-related increases in river flood hazards, other processes are equally important as illustrated in Fig. 15. Under the hypothesis that floods were directly related to heavy precipitation without other effects, their timing within the year would have to be identical, but this is not the case. Floods in northwestern Europe usually occur in winter, a few weeks after the most frequent heavy rains, when the soils are wetter than before. In northern Europe, the most relevant floods occur in spring as a result of snowmelt, while the most extreme precipitation occurs in summer. In other regions of Europe, more subtle, seasonal interactions of soil moisture, snow and extreme precipitation control the timing and thus the magnitude of flooding (Sivapalan et al., 2005; Blöschl et al., 2017). In order to understand changes in flood hazards, it is thus not enough to understand the atmospheric processes, but also the seasonal hydrology involved.
A more formal attribution of observed flood changes to changing precipitation, soil moisture and snowmelt is shown in Fig. 16. In northwestern Europe, the main driver of increasing flood hazards is an increase in heavy precipitation while increases in soil moisture play a more minor role. In southern Europe, the reduction of soil moisture due to increased evaporation is the main control of decreasing flood hazards, and decreases in precipitation are somewhat less important. In eastern Europe, warmer temperatures have resulted in less snowmelt and smaller snowmelt floods which has decreased the flood hazard, and neither heavy precipitation nor soil moisture changes play a relevant role (Kemter et al., 2020). Bertola et al. (2021) suggested that flood peak runoff increases by about 1 % if heavy precipitation increases by 1 % (equivalent to an elasticity of 1 % per percent, and there is little dependence on the return period. In contrast, the elasticity to soil moisture decreases with return period, as would be expected because it approaches soil saturation (see e.g. Grillakis et al., 2016; Wasko and Nathan, 2019), and ranges from about 0.5 % per percent in southern Europe to smaller values in the rest of the continent. The elasticity to snowmelt in northeastern Europe is greater than 1 % per percent and decreased slightly with the return period.
This review article examines three hypotheses sometimes raised in public debate: land-use change increases the flood hazard; hydraulic structures increase the flood hazard; and climate change increases the flood hazard.
Not surprisingly, the answer to all three hypotheses is: “it depends”. Clearly, there is neither an affirmative nor negative answer to all three of them in all cases. All three changes have the potential to not only increase flood hazards, but also to reduce them. For all three changes, the main factors for this potential to be realised are
-
catchment scale,
-
event magnitude,
-
the extent of the impact, and
-
the local (or regional) hydrological situation.
Land use. Small catchments of a few hectares are those where short, intense storms are most critical for flooding because of the short travel times of the raindrops. In these catchments, deforestation, urbanisation and soil compaction by agriculture are likely to significantly increase flood hazards, as the main runoff generation mechanism is usually of the infiltration excess type, controlled by infiltration capacity which is very much affected by land-use change. There is a tendency for the land-use change effect to be greatest for storms of small and moderate magnitudes, while it diminishes for extreme storms when the soil is close to saturation. For extreme storms, however, geomorphologic processes, such as debris flow and landsliding, may become relevant, which may be substantially enhanced by deforestation. On the other hand, in large catchments with a size of a few to thousands of square kilometres, longer, lower-intensity storms tend to be more relevant in producing regional floods. In these catchments, land-use change has much less potential to increase the flood hazard, as runoff generation is often of the saturation excess type (at least in temperate climates), which is impacted very little by land use, but is rather controlled by soil depth and the spatial distribution of soil saturation areas. Figure 17 schematically show the diminishing land-use change effects with catchment scale. Also, large catchments are less likely to undergo complete land-use change than smaller catchments (Rogger et al., 2017; Blöschl et al., 2007), which may further reduce impacts, consistent with a general finding that, at large scales, land-use change effects on flood hazards are much smaller than climate-change effects (see, e.g. Yang et al., 2021).
Hydraulic structures. In small catchments, flood hazards may be efficiently reduced by the construction of flood-retention basins because the critical storms are short, and thus the flood volumes are small. These basins work best for floods with medium return periods, while they become ineffective for the largest floods since they fill up early during the event. In large catchments, retention basins are much less effective, so river training, or channel straightening, and levees are often used as flood management alternatives along medium and large rivers, as illustrated in Fig. 17. Locally, i.e. along the reach they are constructed, these measures reduce the flood hazard (this is the reason why they are built!), at least for floods of small and medium magnitudes. Immediately downstream, however, such measures may have unintended consequences. Because of the loss of floodplain storage, the downstream hazard associated with medium-sized floods (return periods ∼10–100 years) may significantly increase, and the magnitude of this increase is related to the ratio of the volumes of the floodplain and the flood event. For extreme floods, however, there is often little flood-hazard increase as floodplains are flooded early during the event and/or the levees are over-flown. When assessing the future flood hazards, the long-term interactions between people and floods should be taken into account, as two-way feedbacks may substantially modify the flood-hazard situation.
Climate change. In small catchments, short duration, high-intensity storms (often of convective origin) tend to increase with air temperature, which likely increases the flood hazard in a warmer climate, both for small and large return periods, although no final word has been spoken on this matter, since these changes are difficult to observe. For larger catchments, the database is much better, and both increases and decreases in flood hazards have been observed around the world. Over a time scale of centuries, there is a tendency for flood-rich periods to occur during which floods are more frequent and of larger magnitudes. In Europe, such periods were colder than usual, with the exception of the last 3 decades which were particularly flood rich in central Europe, but warmer. At the regional scale, flood-hazard changes are not necessarily directly linked to precipitation, nor are they directly linked to rising air temperatures, but are driven by the seasonal interaction of soil moisture, snow and extreme precipitation via runoff generation. In northwestern Europe, the main driver of increasing flood hazards in the last decades is an increase in heavy precipitation. In southern Europe, the decreasing flood hazard is related to drier soils and somewhat less precipitation, while in eastern Europe, decreasing flood hazards result from less intense snowmelt. While the effect of heavy precipitation and snowmelt on flood hazards does not change much with the return period, that of soil moisture tends to decrease as the soils reach saturation. As highlighted in Fig. 17, climate change may affect flood hazards at all catchment scales, even though the flood generation processes are fundamentally different.
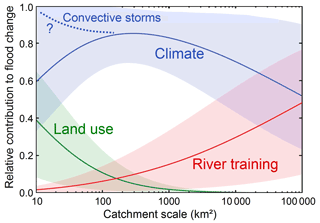
Figure 17Synthesis of the relative contributions of land-use change, river training and climate change to flood-hazard changes as a function of catchment scale. Land-use change is most relevant for small catchments, river training for large catchments and climate change at all catchment scales. For small catchments, an increase in the frequency of convective storms may further exacerbate climate-change effects on floods (Viglione et al., 2016).
Much of the insight brought out in the article is based on work in Europe, and the question arises as to whether it also applies to other continents and climates. Decreasing intensity of extreme storms with storm duration (Courty et al., 2018) and increasing travel times with increasing catchment size (e.g. Gaál et al., 2012; Watt and Chow, 1985) appear to occur globally. This points to a rather universal behaviour of diminishing land-use change effects with catchment scale on flood hazards in those regions where both infiltration excess and saturation excess runoff occur. Analyses of observed floods in China and South America suggest that the findings obtained here are also valid in these regions (e.g. Yang et al., 2021; Chagas et al., 2022). In arid climates, infiltration excess may be more dominant, so large catchments may be similarly susceptible to land-use change effects as small ones, and in cold climates, the dominance of saturation excess may result in little impact in both small and large catchments on flood hazards. On the other hand, given that most runoff generation mechanisms exhibit similar behaviour with respect to soil moisture (Blöschl, 2022), the tendency for land-use change effects to decrease with storm size may be rather universal. Hydraulic structures would be expected to affect floods in very much the same way around the world, given that both mass balance and roughness–velocity relationships are generic. However, long-term interactions between people and flood hazards will depend on both the hydrological setting and the economic and cultural characteristics of the country (see, e.g. Di Baldassarre). Climate-change effects on flood hazards in small catchments resulting from an intensification of convective storms may potentially be similar around the world for thermodynamic reasons, but more observations are needed to shed light on this issue. For extratropical cyclones affecting Europe and other mid-latitude continents, Fig. 11 indicates increases and decreases in flood hazards. In the subtropics, where monsoons are a major cause of flooding, there is some evidence of increased monsoon rainfall, although inconsistencies between observations and physical interpretations remain (Seth et al., 2019). Observations indicate a widening of the areal band around the Equator in which tropical cyclones occur, as well as an intensification of tropical cyclones in some regions of the world (Walsh et al., 2019), but this is not reflected in a corresponding documented increase in flood hazards at this time (Fig. 11).
The assessment of the three hypotheses has a number of implications. First and foremost, it is clear that significant progress has been made in recent years in understanding the role of land use, hydraulic structures and climate in changing river flood hazards. Given that powerful mathematical modelling has become feasible and easily affordable, often more so than monitoring and experimentation, there is a tendency for overreliance on mathematical models. This review has adopted a data-based approach, where possible, and it is strongly believed that modelling and observations need to be in balance. Modelling of course has an important role to play in assessing the affect of future management options (Hamilton et al., 2015), but there is always a danger of attracting a bit of the “garbage in, garbage out” syndrome.
The second implication concerns the choice of flood management instruments for reducing flood hazards. In choosing flood management instruments, we need to be realistic about their ability to really reduce flood hazards. The discussion on nature-based solutions (Kumar et al., 2021; Reaney, 2022) is not always clear on this. Afforestation may be desirable for many reasons, including aesthetical, ecological, touristic and economic, but we need to be aware that its effect on flood hazards tends to diminish to virtually zero for large events. Similarly, green roofs, wetlands and river restoration can only be expected to mitigate floods in proportion to the volume stored relative to the flood-event volume, and again, their effect on extreme floods tends to be small, particularly in large catchments. The efficiency of most other instruments of course also depends on the event magnitude, so, while clearly recognising the limits of individual measures, an integrated portfolio of diverse methods is often a prudent choice for minimising flood hazards.
The third implication regards the public debate on natural disasters, and demonstrates a clear need for avoiding oversimplified narratives. As is often the case, reality is more complex than one would like to think, and flood hazards are no exception. Perhaps as scientists, we need to better live up to our responsibility of making the public debate more evidence-based. Clearly, communication beyond the scientific community is essential. Hydrologists play a particularly important role here, since hydrology is an integrative science and deals with issues such as flood-hazard change at the core of its discipline (Sivapalan, 2018; Blöschl et al., 2019a). While ecologists and foresters, hydraulic engineers and climatologists have specific expertise in land use, hydraulic structures and climate, the hydrologists are the ones tasked with “bringing it all together” to understand, and communicate, flood-hazard changes. It is crucial to consider all three change factors in flood risk management and communicate them to the public in a nuanced way.
No data sets were used in this article.
The author is a member of the editorial board of Hydrology and Earth System Sciences. The peer-review process was guided by an independent editor, and the author also has no other competing interests to declare.
Publisher's note: Copernicus Publications remains neutral with regard to jurisdictional claims in published maps and institutional affiliations.
This article is an outgrowth from the Alfred Wegener Medal Lecture I gave at the General Assembly of the European Geosciences Union in May 2022. Over the years, my own ideas that I have presented here, have benefitted immensely from interactions with numerous friends and collaborators which I would like to thank most cordially. These include the co-authors with which I have published the most on flood-hazard issues: Alberto Viglione, Alberto Montanari, Andrea Kiss, Bruno Merz, David Lun, Jan Szolgay, Juraj Parajka, Jürgen Komma, Magdalena Rogger, Miriam Bertola, Murugesu Sivapalan, Ralf Merz, Abdallah Alaoui, Alessio Domeneghetti, Alexia Fürnkranz-Prskawetz, Ali Gul, Andreas Buttinger-Kreuzhuber, Annegret Thieken, Ardian Bilibashi, Attilio Castellarin, Berit Arheimer, Bernhard Kohl, Christian Reszler, Conor Murphy, Dan Rosbjerg, Daniel Skublics , Daniele Ganora, Denis Hughes, Dieter Gutknecht, Donna Wilson, Elena Szolgayová, Elena Toth, Elena Volpi, Eric Sauquet, Fuqiang Tian, Giovanni B. Chirico, Giugliano DiBaldassarre, Giuseppe Tito Aronica, Graham Jewitt, Günter Humer, Heidi Kreibich, Heiko Apel, Heinz Stiefelmeyer, Hessel Winsemius, Hongyi Li, Ioannis Tsanis, Ivan Čanjevac, Ivan Radevski, Jamie Hannaford, Jarkko J. Koskela, Jean Dominique Creutin, Jery Stedinger, José Salinas, Julia Hall, Jürgen Waser, Klaus Haslinger, Klodian Zaimi, Korbinian Breinl, Ladislav Gaál, Larisa Tarasova, Liudmyla Gorbachova, Long Yang, Luca Solari, Luis Mediero, Manolis Grillakis, Marco Borga, Maria del Carmen Llasat Botija, Maria Kireeva, Maria Mavrova-Guirguinova, Marlies Barendrecht, Marzena Osuch, Matthias Kemter, Michael Hofer, Michael Hofstätter, Michael Wimmer, Miloň Bohač, Mojca Šraj, Natalia Frolova, Neil Macdonald, Neil McIntyre, Nenad Živković, Ognjen Bonacci, Ondrej Ledvinka, Paul Bates, Pedro Chaffe, Peter Molnar, Peter Rutschmann, Peter Strauss, Peter Troch, Peter Valent, Pierluigi Claps, Ruby Leung, Rudolf Brázdil, Rui Perdigão, Saket Pande, Sally Thompson, Serena Ceola, Sergiy Vorogushyn, Shaun Harrigan, Silvia Kohnová, Simone Persiano, Stacey Archfield, Stephan Peth, Tetiana Zabolotnia, Thomas Nester, Thomas R. Kjeldsen, Upmanu Lall, Valeryia Ovcharuk, Vincent Chaplot, Vinícius Chagas, Wei Wang, William Farmer, Wolfgang Schöner, Zbigniew Kundzewicz, Zdeněk Kostka, Zsolt Horváth. I would like to thank the three anonymous reviewers for the assessment of the manuscript.
The research was supported by many projects over the years. European funding agencies: FloodChange (ERC Advanced Grant, 291152), System Risk (Horizon 2020 Etn, 676027), Starflood (Horizon 2020, 793558), Impala (FP7-People, 301953), Switch-On (FP7-Env, 603587). Austrian Science Funds: Vienna Doctoral Programme on Water Resource Systems (W1219), Spate (I 3174, I 4776), Dominant Processes (P 23723). Austrian Academy of Sciences: Mountain Floods. Austrian Ministry of Agriculture: Wetrax+, Flood risk zoning Austria 3.0, Eranet-Crue. State Government of Tirol: AlpRetInn.
This paper was edited by Alberto Guadagnini and reviewed by three anonymous referees.
Alaoui, A., Rogger, M., Peth, S., and Blöschl, G.: Does soil compaction increase floods?, A review, J. Hydrol., 557, 631–642, https://doi.org/10.1016/j.jhydrol.2017.12.052, 2018.
Archer, C. L. and Caldeira, K.: Historical trends in the jet streams, Geophys. Res. Lett. 35, L08803, https://doi.org/10.1029/2008GL033614, 2008.
Barendrecht, M. H., Viglione, A., and Blöschl, G.: A dynamic framework for flood risk, Water Secur., 1, 3–11, https://doi.org/10.1016/j.wasec.2017.02.001, 2017.
Barendrecht, M. H., Viglione, A., Kreibich, H., Merz, B., Vorogushyn, S., and Blöschl, G.: The value of empirical data for estimating the parameters of a sociohydrological flood risk model, Water Resour. Res., 55, 1312–1336, https://doi.org/10.1029/2018WR024128, 2019.
Bertola, M., Viglione, A., Lun, D., Hall, J., and Blöschl, G.: Flood trends in Europe: are changes in small and big floods different?, Hydrol. Earth Syst. Sci., 24, 1805–1822, https://doi.org/10.5194/hess-24-1805-2020, 2020.
Bertola, M., Viglione, A., Vorogushyn, S., Lun, D., Merz, B., and Blöschl, G.: Do small and large floods have the same drivers of change?, A regional attribution analysis in Europe, Hydrol. Earth Syst. Sci., 25, 1347–1364, https://doi.org/10.5194/hess-25-1347-2021, 2021.
Beschta, R. L., Pyles, M. R., Skaugset, A. E., and Surfleet, C. G.: Peakflow responses to forest practices in the western cascades of Oregon, USA, J. Hydrol., 233, 102–120, 2000.
Beven, K. J.: Rainfall-runoff modelling: the primer, John Wiley and Sons, Chichester, UK, 356 pp., 2011.
Blazejewski, R., Pilarczyk, K. W., and Przedwojski, B.: River training techniques: fundamentals, design and applications, CRC Press, ISBN 90 5410 1962, 1995.
Blöschl, G.: Two water problems of a big city, in: The Face of the Earth: The Legacy of Eduard Suess, edited by: Hofmann, T., Blöschl, G., Lammerhuber, L., Piller, W. E., and Şengör, A. M. C., Edition Lammerhuber, Vienna, 35–39, 2014.
Blöschl, G.: Engineering strategies of enhancing the resilience of cities of art to floods, Atti dei convegni Lincei 315, Accademia Nazionale dei Lincei, Bardi Edizioni, Roma, 23–38, 2017.
Blöschl, G.: Flood generation: process patterns from the raindrop to the ocean, Hydrol. Earth Syst. Sci., 26, 2469–2480, https://doi.org/10.5194/hess-26-2469-2022, 2022.
Blöschl, G. and Sivapalan, M.: Scale issues in hydrological modelling – a review, Hydrol. Proc., 9, 251–290, 1995.
Blöschl, G., Ardoin-Bardin, S., Bonell, M., Dorninger, M., Goodrich, D., Gutknecht, D., Matamoros, D., Merz, B., Shand, P., and Szolgay, J.: At what scales do climate variability and land cover change impact on flooding and low flows?, Hydrol. Proc., 21, 1241–1247, https://doi.org/10.1002/hyp.6669, 2007.
Blöschl, G., Nester, T., Komma, J., Parajka, J., and Perdigão, R. A. P.: The June 2013 flood in the Upper Danube Basin, and comparisons with the 2002, 1954 and 1899 floods, Hydrol. Earth Syst. Sci., 17, 5197–5212, https://doi.org/10.5194/hess-17-5197-2013, 2013.
Blöschl, G., Gaál, L., Hall, J., Kiss, A., Komma, J., Nester, T., Parajka, J., Perdigão, R. A. P., Plavcová, L., Rogger, M., Salinas, J. L., and Viglione, A.: Increasing river floods: fiction or reality?, Wiley Interdisciplinary Reviews, Water, 2, 329–344, 2015.
Blöschl, G., Blaschke, A. P., Broer, M., Bucher, C., Carr, G., Chen, X., Eder, A., Exner-Kittridge, M., Farnleitner, A., Flores-Orozco, A., Haas, P., Hogan, P., Kazemi Amiri, A., Oismüller, M., Parajka, J., Silasari, R., Stadler, P., Strauss, P., Vreugdenhil, M., Wagner, W., and Zessner, M.: The Hydrological Open Air Laboratory (HOAL) in Petzenkirchen: a hypothesis-driven observatory, Hydrol. Earth Syst. Sci., 20, 227–255, https://doi.org/10.5194/hess-20-227-2016, 2016.
Blöschl, G., Hall, J., Parajka, J., Perdigão, R. A. P., Merz, B., Arheimer, B., Aronica, G. T., Bilibashi, A., Bonacci, O., Borga, M., Canjevac, I., Castellarin, A., Chirico, G. B., Claps, P., Fiala, K., Frolova, N., Gorbachova, L., Gül, A., Hannaford, J., Harrigan, S., Kireeva, M., Kiss, A., Kjeldsen, T. R., Kohnová, S., Koskela, J. J., Ledvinka, O., Macdonald, N., Mavrova- Guirguinova, M., Mediero, L., Merz, R., Molnar, P., Montanari, A., Murphy, C., Osuch, M., Ovcharuk, V., Radevski, I., Rogger, M., Salinas, J. L., Sauquet, E., Šraj, M., Szolgay, J., Viglione, A., Volpi, E., Wilson, D., Zaimi, K., and Živkovic, N.: Changing climate shifts timing of European floods, Science, 357, 588–590, 2017.
Blöschl, G., Komma, J., Nester, T., Rogger, M., Salinas, J. L., and Viglione, A.: Die Wirkung des Waldes auf Hochwässer, Wildbach-und Lawinenverbau, 88, 288–296, 2018.
Blöschl, G., Bierkens, M. F. P., Chambel, A., et al.: Twentythree Unsolved Problems in Hydrology (UPH) – a community perspective, Hydrolog. Sci. J., 64, 1141–1158, https://doi.org/10.1080/02626667.2019.1620507, 2019a.
Blöschl, G., Hall, J., Parajka, J., Perdigão, R. A. P., Merz, B., Arheimer, B., Aronica, G. T., Bilibashi, A., Boháč, M. Bonacci, O., Borga, M., Čanjevac, I., Castellarin, A., Chirico, G. B., Claps, P., Fiala, K., Frolova, N., Gorbachova, L., Gül, A., Hannaford, J., Harrigan, S., Kireeva, M., Kiss, A., Kjeldsen, T. R., Kohnová, S., Koskela, J. J., Ledvinka, O., Macdonald, N., Mavrova- Guirguinova, M., Mediero, L., Merz, R., Molnar, P., Montanari, A., Murphy, C., Osuch, M., Ovcharuk, V., Radevski, I., Rogger, M., Salinas, J. L., Sauquet, E., Šraj, M., Szolgay, J., Viglione, A., Volpi, E., Wilson, D., Zaimi, K., and Živković, N.: Changing climate both increases and decreases European river floods, Nature, 573, 108–111, https://doi.org/10.1038/s41586-019-1495-6, 2019b.
Blöschl, G., Kiss, A., Viglione, A., Barriendos, M., Böhm, O., Brázdil, R., Coeur, D., Demarée, G., Llasat, M. C., Macdonald, N., Retsö, D., Roald, L., Schmocker-Fackel, P., Amorim, I., Belinová, M., Benito, G., Bertolin, C., Camuffo, D., Cornel, D., Doctor, R., Elleder, L., Enzi, S., Garcia, J. C., Glaser, R., Hall, J., Haslinger, K., Hofstätter, M., Komma, J., Limanówka, D., Lun, D., Panin, A., Parajka, J., Petric, H., Rodrigo, F. S., Rohr, C., Schönbein, J., Schulte, L., Silva, L. P., Toonen, W., Valent, P., Waser, J., and Wetter, O.: Current flood-rich period is exceptional compared to the past 500 years in Europe, Nature, 583, 560–566, https://doi.org/10.1038/s41586-020-2478-3, 2020.
Blöschl, G., Waser, J., Buttinger-Kreuzhuber, A., Cornel, D., Eisl, J., Hofer, M., Hollaus, M., Horváth, Z., Komma, J., Konev, A., Parajka, J., Pfeifer, N., Reithofer, A., Salinas, J., Valent, P., Viglione, A., Wimmer, M., and Stiefelmeyer, H.: HOchwasserRisikozonierung Austria 3.0 (HORA 3.0), Österreichische Wasser-und Abfallwirtschaft, 1–12, https://doi.org/10.1007/s00506-022-00848-7, 2022.
Breinl, K., Lun, D., Müller-Thomy, H., and Blöschl, G.: Understanding the relationship between rainfall and flood probabilities through combined intensity-duration-frequency analysis, J. Hydrol., 602, 1–19, https://doi.org/10.1016/j.jhydrol.2021.126759, 2021.
Brown, A. E., Zhang, L., McMahon, T. A., Western, A. W., and Vertessy, R. A.: A review of paired catchment studies for determining changes in water yield resulting from alterations in vegetation, J. Hydrol., 310, 28–61, 2005.
Burton, I., Kates, R. W., and White, G. F.: The human ecology of extreme geophysical events, FMHI Publications, Paper 78, 1968.
Buttinger-Kreuzhuber, A., Horváth, Z., Noelle, S., Blöschl, G., and Waser, J.: A fast second-order shallow water scheme on two-dimensional structured grids over abrupt topography, Adv. Water Resour., 127, 89–108, https://doi.org/10.1016/j.advwatres.2019.03.010, 2019.
Chagas, V. B. P., Chaffe, P. L. B., and Blöschl, G: Climate and land management accelerate the Brazilian water cycle, Nat. Commun., 13, 1–10, https://doi.org/10.1038/s41467-022-32580-x, 2022.
Contractor, S., Donat, M. G., and Alexander, L. V.: Changes in observed daily precipitation over global land areas since 1950, J. Climate, 34, 3–19, 2021.
Courty, L. G., Wilby, R. L., Hillier, J. K., and Slater, L. J.: Intensity-duration-frequency curves at the global scale, Environ. Res. Lett., 14, 1–10, https://doi.org/10.1088/1748-9326/ab370a, 2019.
Di Baldassarre, G., Viglione, A., Carr, G., Kuil, L., Yan, K., Brandimarte, L., and Blöschl, G.: Debates – Perspectives on socio: Capturing feedbacks between physical and social processes, Water Resour. Res., 51, 4770–4781, 2015.
Do, H. X., Zhao, F., Westra, S., Leonard, M., Gudmundsson, L., Boulange, J. E. S., Chang, J., Ciais, P., Gerten, D., Gosling, S. N., Müller Schmied, H., Stacke, T., Telteu, C.-E., and Wada, Y.: Historical and future changes in global flood magnitude – evidence from a model–observation investigation, Hydrol. Earth Syst. Sci., 24, 1543–1564, https://doi.org/10.5194/hess-24-1543-2020, 2020.
Doocy, S., Daniels, A., Murray, S., and Kirsch, T. D.: The human impact of floods: a historical review of events 1980–2009 and systematic review, PLOS Curr., https://pubmed.ncbi.nlm.nih.gov/23857425/, 2013.
Dunne, T. and Black, R. D.: An experimental investigation of runoff production in permeable soils, Water Resour. Res., 6, 478–490, 1970.
Erskine, W. D.: Channel response to largescale river training works: Hunter River, Australia, Regulated Rivers, Research Manage., 7, 261–278, 1992.
Field, C. B., Barros, V., Stocker, T. F., and Dahe, Q. (Eds.): Managing the risks of extreme events and disasters to advance climate change adaptation: special report of the intergovernmental panel on climate change, Cambridge University Press, ISBN 978-1-107-02506-6, 2012.
Fowler, H. J., Lenderink, G., Prein, A. F., Westra, S., Allan, R. P., Ban, N., Barbero, R., Berg, P., Blenkinsop, S., Do, H. X., Guerreiro, S., Haerter, J. O., Kendon, E. J., Lewis, E., Schär, C., Sharma, A., Villarini, G., Wasko, C., and Zhang, X.: Anthropogenic intensification of short-duration rainfall extremes, Nat. Rev. Earth Environ., 2, 107–122, 2021.
François, B., Schlef, K. E., Wi, S., and Brown, C. M.: Design considerations for riverine floods in a changing climate – a review, J. Hydrol., 574, 557–573, 2019.
Gaál, L., Szolgay, J., Kohnová, S., Parajka, J., Merz, R., Viglione, A., and Blöschl, G.: Flood timescales: Understanding the interplay of climate and catchment processes through comparative hydrology, Water Resour. Res., 48, W04511, https://doi.org/10.1029/2011WR011509, 2012.
Gagen, M. H., Zorita, E., McCarroll, D., Zahn, M., Young, G. H., and Robertson, I.: North Atlantic summer storm tracks over Europe dominated by internal variability over the past millennium, Nat. Geosci., 9, 630–635, 2016.
Gao, M., Li, H. Y., Liu, D., Tang, J., Chen, X., Chen, X., Blöschl, G., and Leung, L. R.. Identifying the dominant controls on macropore flow velocity in soils: A meta-analysis, J. Hydrol., 567, 590–604, https://doi.org/10.1016/j.jhydrol.2018.10.044, 2018.
Gao, S., Liu, P., Pan, Z., Ming, B., Guo, S., Cheng, L., and Wang, J.: Incorporating reservoir impacts into flood frequency distribution functions, J. Hydrol., 568, 234–246, 2019.
Gavin, N. T., Leonard-Milsom, L., and Montgomery, J.: Climate change, flooding and the media in Britain, Public Understanding of Science, 20, 422–438, 2011.
Glade, T.: Landslide occurrence as a response to land use change: a review of evidence from New Zealand, Catena, 51, 297–314, 2003.
Grillakis, M., Koutroulis, A., Komma, J., Tsanis, I., Wagner, W., and Bloschl, G.: Initial soil moisture effects on flash flood generation . A comparison between basins of contrasting hydro-climatic conditions, J. Hydrol., 541, 206–217, https://doi.org/10.1016/j.jhydrol.2016.03.007, 2016.
Hall, J., Arheimer, B., Borga, M., Brázdil, R., Claps, P., Kiss, A., Kjeldsen, T. R., Kriaučiūnienė, J., Kundzewicz, Z. W., Lang, M., Llasat, M. C., Macdonald, N., McIntyre, N., Mediero, L., Merz, B., Merz, R., Molnar, P., Montanari, A., Neuhold, C., Parajka, J., Perdigão, R. A. P., Plavcová, L., Rogger, M., Salinas, J. L., Sauquet, E., Schär, C., Szolgay, J., Viglione, A., and Blöschl, G.: Understanding flood regime changes in Europe: a state-of-the-art assessment, Hydrol. Earth Syst. Sci., 18, 2735–2772, https://doi.org/10.5194/hess-18-2735-2014, 2014.
Hamilton, S. H., ElSawah, S., Guillaume, J. H., Jakeman, A. J., and Pierce, S. A.: Integrated assessment and modelling: overview and synthesis of salient dimensions, Environ. Modell. Softw., 64, 215–229, 2015.
Hess, T. M., Holman, I. P., Rose, S. C., Rosolova, Z., and Parrott, A.: Estimating the impact of rural land management changes on catchment runoff generation in England and Wales, Hydrol. Proc., 24, 1357–1368, https://doi.org/10.1002/hyp.7598, 2010.
Hofstätter, M. and Blöschl G.: Vb cyclones synchronized with the Arctic-/North Atlantic Oscillation, J. Geophys. Res.-Atmos., 124, 3259–3278, https://doi.org/10.1029/2018JD029420, 2019.
Hofstätter, M., Chimani, B., Lexer, A., and Blöschl, G.: A new classification scheme of European cyclone tracks with relevance to precipitation, Water Resour. Res., 52, 7086–7104, https://doi.org/10.1002/2016WR019146, 2016.
Hofstätter, M., Lexer, A., Homann, M., and Blöschl, G.: Largescale heavy precipitation over central Europe and the role of cyclone track types, Int. J. Climatol., 38, 497–517, https://doi.org/10.1002/joc.5386, 2018.
Horváth, Z., Buttinger-Kreuzhuber, A., Konev, A., Cornel, D., Komma, J., Blöschl, G., Noelle, S., and Waser, J.: Comparison of fast shallow-water schemes on real-world floods, J. Hydraul. Eng., 146, 05019005, https://doi.org/10.1061/(ASCE)HY.1943-7900.0001657, 2020.
Hurrell, J. W. and Van Loon, H.: Decadal variations in climate associated with the North Atlantic Oscillation, in: Climatic change at high elevation sites, Springer, Dordrecht, 69–94, 1997.
Jones, J. A. and Grant, G. E.: Peak flow responses to clear-cutting and roads in small and large basins, western Cascades, Oregon, Water Resour. Res., 32, 959–974, 1996.
Kang, S. M. and Lu, J.: Expansion of the Hadley cell under global warming: winter versus summer, J. Climate, 25, 8387–8393, 2012.
Keller, T., Sandin, M., Colombi, T., Horn, R., and Or, D.: Historical increase in agricultural machinery weights enhanced soil stress levels and adversely affected soil functioning, Soil Tillage Res., 194, 104293, https://doi.org/10.1016/j.still.2019.104293, 2019.
Kemter, M., Merz, B., Marwan, N., Vorogushyn, S., and Blöschl, G.: Joint trends in flood magnitudes and spatial extents across Europe, Geophys. Res. Lett., 47, e2020GL087464, https://doi.org/10.1029/2020GL087464, 2020.
Kohnová, S., Roncák, P., Hlavcová, K., Szolgay, J., and Rutkowska, A.: Future impacts of land use and climate change on extreme runoff values in selected catchments of Slovakia, Meteorol. Hydrol. Water Manage., 7, 47–55, 2019.
Kumar, P., Debele, S. E., Sahani, J., Rawat, N., Marti-Cardona, B.,Alfieri, S. M., Basu, B., Basu, A. S., Bowyer, P., Charizopoulos, N., Gallotti, G., Jaakko, J., Leo, L. S.,Loupis, M., Menenti, M., Mickovski, S. B., Mun, S.-J.,Gonzalez-Ollauri, A., Pfeiffer, J., Pilla, F., Pröll, J., Rutzinger, M., Santo, M. A., Sannigrahi, S., Spyrou, C., Tuomenvirta, H., Zieher, T.: Nature-based solutions efficiency evaluation against natural hazards: Modelling methods, advantages and limitations, Sci. Total Environ., 784, 1–27, 2021.
LGH (Landesamt für Gewässerkunde und Hauptnivellements): Jahrbuch für die Gewässerkunde Norddeutschlands, Besondere Mitteilung Bd 5, Nr 2 Berlin, p. 5, 1929.
Liu, J. and Zhang, Y.: Multi-temporal clustering of continental floods and associated atmospheric circulations, J. Hydrol., 555, 744–759, 2017.
Lochbihler, K., Lenderink, G., and Siebesma, A. P.: The spatial extent of rainfall events and its relation to precipitation scaling, Geophys. Res. Lett., 44, 8629–8636, 2017.
Lorente, A., García-Ruiz, J. M., Beguería, S., and Arnáez, J.: Factors explaining the spatial distribution of hillslope debris flows, Mountain Res. Develop., 22, 32–39, 2002.
Lu, J., Vecchi, G. A., and Reichler, T.: Expansion of the Hadley cell under global warming, Geophys. Res. Lett,, 34, 1–5, https://doi.org/10.1029/2006GL028443, 2007.
Lun, D., Fischer, S., Viglione, A., and Blöschl, G.: Detecting flood-rich and flood-poor periods in annual peak discharges across Europe, Water Resour. Res., 56, e2019WR026575, https://doi.org/10.1029/2019WR026575, 2020.
Masson-Delmotte, V., Zhai, P., Pirani, A., Connors, S. L., Péan, C., Berger, S., and Zhou, B.: Climate change 2021: the physical science basis. Contribution of working group I to the sixth assessment report of the intergovernmental panel on climate change, 2, https://www.ipcc.ch/report/ar6/wg1/ (last access: 5 September 2022), 2021.
Matczak, P. and Hegger, D. L. T.: Flood Risk Governance for More Resilience – Reviewing the Special Issue's Contribution to Existing Insights, Water, 12, 2122, https://doi.org/10.3390/w12082122, 2020.
McGrath, M.: Climate change: Big increase in weather disasters over the past five decades, BBC News, 1 September 2021, https://www.bbc.com/news/science-environment-58396975 (last access: 5 September 2022), 2021.
Merz, B., Vorogushyn, S., Uhlemann, S., Delgado, J., and Hundecha, Y.: HESS Opinions “More efforts and scientific rigour are needed to attribute trends in flood time series”, Hydrol. Earth Syst. Sci., 16, 1379–1387, https://doi.org/10.5194/hess-16-1379-2012, 2012.
Merz, B., Aerts, J., Arnbjerg-Nielsen, K., Baldi, M., Becker, A., Bichet, A., Blöschl, G., Bouwer, L. M., Brauer, A., Cioffi, F., Delgado, J. M., Gocht, M., Guzzetti, F., Harrigan, S., Hirschboeck, K., Kilsby, C., Kron, W., Kwon, H.-H., Lall, U., Merz, R., Nissen, K., Salvatti, P., Swierczynski, T., Ulbrich, U., Viglione, A., Ward, P. J., Weiler, M., Wilhelm, B., and Nied, M.: Floods and climate: emerging perspectives for flood risk assessment and management, Nat. Hazards Earth Syst. Sci., 14, 1921–1942, https://doi.org/10.5194/nhess-14-1921-2014, 2014.
Merz, B., Vorogushyn, S. Lall, U., Viglione, A., and Blöschl, G.: Charting unknown waters – On the role of surprise in flood risk assessment and management, Water Resour. Res., 51, 6399–6416, https://doi.org/10.1002/2015WR017464, 2015.
Merz, B., Blöschl, G., Vorogushyn, S., Dottori, F., Aerts, J. C., Bates, P., Bertola, M., Kemter, M., Kreibich, H., Lall, U., and Macdonald, E.: Causes, impacts and patterns of disastrous river floods, Nat. Rev. Earth Environ., 2, 592–609, 2021.
Miller, J. D. and Hutchins, M.: The impacts of urbanisation and climate change on urban flooding and urban water quality: A review of the evidence concerning the United Kingdom, J. Hydrol., 12, 345–362, 2017.
Molnar, P., Fatichi, S., Gaál, L., Szolgay, J., and Burlando, P.: Storm type effects on super Clausius–Clapeyron scaling of intense rainstorm properties with air temperature, Hydrol. Earth Syst. Sci., 19, 1753–1766, https://doi.org/10.5194/hess-19-1753-2015, 2015.
Montanari, A. and Koutsoyiannis, D.: Modeling and mitigating natural hazards: Stationarity is immortal!, Water Resour. Res., 50, 9748–9756, 2014.
Mulvany, T. J.: On the use of self-registering rain and flood gauges, T. Inst. Civ. Eng. Ireland, 4, 1–8, 1851.
Pielke Jr., R. S.: The Honest Broker: Making Sense of Science in Policy and Politics, Cambridge University Press, ISBN 978-0-521-87320-8, 2007.
Reaney, S. M.: Spatial targeting of naturebased solutions for flood risk management within river catchments, J. Flood Risk Manage., 15, 1–12, https://doi.org/10.1111/jfr3.12803, 2022.
Rogger, M., Agnoletti, M., Alaoui, A., Bathurst, J. C., Bodner, G., Borga, M., Chaplot, V., Gallart, F., Glatzel, G., Hall, J., Holden, J., Holko, L., Horn, R., Kiss, A., Kohnova, S., Leitinger, G., Lennartz, B., Parajka, J., Perdigão, R., Peth, S., Plavcova, L., Quinton, J. N., Robinson, M., Salinas, J. L., Santoro, A., Szolgay, J., Tron, S., van den Akker, J. J. H., Viglione, A., and Blöschl, G.: Land-use change impacts on floods at the catchment scale: Challenges and opportunities for future research, Water Resour. Res., 53, 5209–5219, https://doi.org/10.1002/2017WR020723, 2017.
Rosbjerg, D., Blöschl, G., Burn, D. H., Castellarin, A., Croke, B., DiBaldassarre, G., Iacobellis, V., Kjeldsen, T. R., Kuczera, G., Merz, R., Montanari, A., Morris, D., Ouarda, T. B. M. J., Ren, L., Rogger, M., Salinas, J. L., Toth, E., and Viglione, A.: Prediction of floods in ungauged basins, in: Runoff Prediction in Ungauged Basins, Synthesis across Processes, Places and Scales, Chap. 9, edited by: Blöschl, G., Sivapalan, M., Wagener, T., Viglione, A., and Savenije, H., Cambridge University Press, Cambridge, UK, 135–162, 2013.
Salazar, S., Francés, F., Komma, J., Blume, T., Francke, T., Bronstert, A., and Blöschl, G.: A comparative analysis of the effectiveness of flood management measures based on the concept of “retaining water in the landscape” in different European hydro-climatic regions, Nat. Hazards Earth Syst. Sci., 12, 3287–3306, https://doi.org/10.5194/nhess-12-3287-2012, 2012.
Seth, A., Giannini, A., Rojas, M., Rauscher, S. A., Bordoni, S., Singh, D., and Camargo, S. J.: Monsoon responses to climate changes – connecting past, present and future, Curr. Clim. Change Rep., 5, 63–79, 2019.
Sivapalan, M. and Blöschl, G.: Time scale interactions and the coevolution of humans and water, Water Resour. Res., 51, 6988–7022, https://doi.org/10.1002/2015WR017896, 2015.
Sivapalan, M., Blöschl, G., Merz, R., and Gutknecht, D.: Linking flood frequency to long-term water balance: Incorporating effects of seasonality, Water Resour. Res., 41, W06012, https://doi.org/10.1029/2004WR003439, 2005.
Sivapalan, M.: From engineering hydrology to Earth system science: milestones in the transformation of hydrologic science, Hydrol. Earth Syst. Sci., 22, 1665–1693, https://doi.org/10.5194/hess-22-1665-2018, 2018.
Skublics, D., Blöschl, G., and Rutschmann, P.: Effect of river training on flood retention of the Bavarian Danube, J. Hydrol. Hydromech., 64, 349–356, https://doi.org/10.1515/johh-2016-0035, 2016.
Šraj, M., Viglione, A., Parajka, J., and Bloschl, G.: The influence of non-stationarity in extreme hydrological events on flood frequency estimation, J. Hydrol. Hydromech., 64, 426–437, https://doi.org/10.1515/johh-2016-0032, 2016.
Swain, D. L., Wing, O. E., Bates, P. D., Done, J. M., Johnson, K. A., and Cameron, D. R.: Increased flood exposure due to climate change and population growth in the United States, Earth's Future, 8, e2020EF001778, https://doi.org/10.1029/2020EF001778, 2020.
Szolgayova, E., Laaha, G., Blöschl, G., and Bucher, C.: Factors influencing long range dependence in streamflow of European rivers, Hydrol. Proc., 28, 1573–1586, https://doi.org/10.1002/hyp.9694, 2014.
Tarasova, L., Merz, R., Kiss, A., Basso, S., Blöschl, G., Merz, B., Viglione, A., Plötner, S., Guse, B., Schumann, A., Fischer, S., Ahrens, B., Anwar, F., Bárdossy, A., Bühler, P., Haberlandt, U., Kreibich, H., Krug, A., Lun, D., Müller-Thomy, H., Pidoto, R., Primo, C., Seidel, J., Vorogushyn, S., and Wietzke, L.: Causative classification of river flood events, Wiley Interdisciplinary Reviews, Water, 6, e1353, https://doi.org/10.1002/wat2.1353, 2019.
Ulbrich, U., Brucher, T., Fink, A. H., Leckebusch, G. C., Kruger, A., and Pinto, J. G.: The central European floods in August 2002, Part II: synoptic causes and considerations with respect to climatic change, Weather, 58, 434–441, 2003.
UNDRR: United Nations Office for Disaster Risk Reduction GAR2015 – Global Assessment Report on Disaster Risk Reduction, 316 pp., 2015.
van Bebber, W. J.: Die Zugstrassen der barometrischen Minima nach den Bahnenkarten der Deutschen Seewarte für den Zeitraum 1875–1890, Meteorol. Z., 8, 361–366, 1891.
Viglione, A. and Blöschl, G.: On the role of storm duration in the mapping of rainfall to flood return periods, Hydrol. Earth Syst. Sci., 13, 205–216, https://doi.org/10.5194/hess-13-205-2009, 2009.
Viglione, A., Merz, R., and Blöschl, G.: On the role of the runoff coefficient in the mapping of rainfall to flood return periods, Hydrol. Earth Syst. Sci., 13, 577–593, https://doi.org/10.5194/hess-13-577-2009, 2009.
Viglione, A., Di Baldassarre, G., Brandimarte, L., Kuil, L., Carr, G., Salinas, J. L., Scolobig, A., and Blöschl, G.: Insights from socio-hydrology modelling on dealing with flood risk – roles of collective memory, risk-taking attitude and trust, J. Hydrol., 518, 71–82, 2014.
Viglione, A., Merz, B., Viet Dung, N., Parajka, J., Nester, T., and Blöschl, G.: Attribution of regional flood changes based on scaling fingerprints, Water Resour. Res., 52, 5322–5340, https://doi.org/10.1002/2016WR019036, 2016.
Volpi, E., Di Lazzaro, M., Bertola, M., Viglione, A., and Fiori, A.: Reservoir effects on flood peak discharge at the catchment scale, Water Resour. Res., 54, 9623–9636, 2018.
Vorogushyn, S., Lindenschmidt, K. E., Kreibich, H., Apel, H., and Merz, B.: Analysis of a detention basin impact on dike failure probabilities and flood risk for a channel-dike-floodplain system along the river Elbe, Germany, J. Hydrol., 436, 120–131, 2012.
Vorogushyn, S., Bates, P. D., de Bruijn, K., Castellarin, A., Kreibich, H., Priest, S., Schröter, K., Bagli, S., Blöschl, G., Domeneghetti, A., Gouldby, B., Klijn, F., Lammersen, R., Neal, J. C., Ridder, N., Terink, W., Viavattene, C., Viglione, A., Zanardo, S., and Merz, B.: Evolutionary leap in large-scale flood risk assessment needed, Wiley Interdisciplinary Reviews, Water, 5, e1266, https://doi.org/10.1002/wat2.1266, 2018.
Walsh, K. J., Camargo, S. J., Knutson, T. R., Kossin, J., Lee, T. C., Murakami, H., and Patricola, C.: Tropical cyclones and climate change, Trop. Cycl. Res. Rev., 8, 240–250, 2019.
Wang, W., Li, H.-Y., Leung, L. R., Yigzaw, W., Zhao, J., Lu, H., Deng, Z., Demisie, Y., and Bloschl, G.: Nonlinear filtering effects of reservoirs on flood frequency curves at the regional scale, Water Resour. Res., 53, 8277–8292, https://doi.org/10.1002/2017WR020871, 2017.
Wasko, C. and Nathan, R.: Influence of changes in rainfall and soil moisture on trends in flooding, J. Hydrol., 575, 432–441, 2019.
Watt, W. E. and Chow, K. A.: A general expression for basin lag time, Can. J. Civil Eng., 12, 294–300, 1985.
World Economic Forum: The Global Risks Report 2022, 17th Edition, ISBN 978-2-940631-09-4, https://www.weforum.org/reports/global-risks-report-2022/, last access: 5 September 2022.
Wyzga, B.: Changes in the magnitude and transformation of flood waves subsequent to the channelization of the Raba River, Polish Carpathians, Earth Surf. Proc. Land., 21, 749–763, 1996.
Xian, T., Xia, J., Wei, W., Zhang, Z., Wang, R., Wang, L., and Ma, Y.: Is Hadley Cell Expanding?, Atmosphere, 12, 1699, https://doi.org/10.3390/atmos12121699, 2021.
Yang, L., Yang, Y., Villarini, G., Li, X., Hu, H., Wang, L., Blöschl, G., and Tian, F.: Climate more important for Chinese flood changes than reservoirs and land use, Geophys. Res. Lett., 48, e2021GL093061, https://doi.org/10.1029/2021GL093061, 2021.
Zhang, Y., Viglione, A., and Blöschl, G.: Temporal scaling of streamflow elasticity to precipitation: a global analysis, Water Resour. Res., 58, e2021WR030601, https://doi.org/10.1029/2021WR030601, 2022.
- Abstract
- Introduction
- First hypothesis: land-use change increases flood hazards
- Second hypothesis: hydraulic structures increase flood hazards
- Third hypothesis: climate change increases flood hazards
- Conclusions
- Data availability
- Competing interests
- Disclaimer
- Acknowledgements
- Financial support
- Review statement
- References
- Abstract
- Introduction
- First hypothesis: land-use change increases flood hazards
- Second hypothesis: hydraulic structures increase flood hazards
- Third hypothesis: climate change increases flood hazards
- Conclusions
- Data availability
- Competing interests
- Disclaimer
- Acknowledgements
- Financial support
- Review statement
- References