the Creative Commons Attribution 4.0 License.
the Creative Commons Attribution 4.0 License.
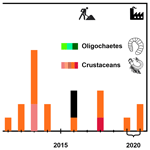
Temporal shift in groundwater fauna in southwestern Germany
Philipp Blum
Heide Stein
Andreas Fuchs
Hans Jürgen Hahn
Kathrin Menberg
Groundwater is an important source of freshwater; drinking water; and service water for irrigation, industrial and geothermal uses. It is also the largest terrestrial freshwater biome in the world. In many areas, this habitat is naturally or anthropogenically threatened. This study uses long-term groundwater data from southwestern Germany to identify shifts in groundwater fauna due to natural or anthropogenic impacts. Comprehensive analysis of metazoan groundwater fauna and abiotic parameters from 16 monitoring wells over 2 decades revealed no overall temporal trends in faunal abundance or biodiversity (in terms of number of species) and no significant large-scale trends in abiotic parameters. While 9 wells out of 16 show stable ecological and hydrochemical conditions at a local level, the remaining wells exhibit shifting or fluctuating faunal parameters. At some locations, these temporal changes are linked to natural causes, such as decreasing dissolved oxygen contents or fluctuating temperatures. A multivariate PHATE (Potential of Heat-diffusion for Affinity-based Trajectory Embedding) analysis suggests that, beside the hydrogeological setting, varying contents of sediment and detritus impact faunal abundance. By examining aerial images of the surroundings of individual wells, we found that anthropogenic impacts, such as construction sites and surface sealing, can cause significant shifts in groundwater fauna and changes in the ecological status in positive as well as negative direction. However, variable faunal compositions and abundances were also observed for sites with very stable abiotic conditions in anthropogenically less affected areas such as the Black Forest. These findings indicate that hydro(geo)logical changes and surface conditions, such as land use, should be assessed in line with hydrochemical parameters to better understand changes in groundwater fauna. Accordingly, reference sites for natural conditions in ecological assessment and biomonitoring schemes for groundwater protection should be selected carefully.
- Article
(7178 KB) - Full-text XML
-
Supplement
(752 KB) - BibTeX
- EndNote
Groundwater is an important source of freshwater; drinking water; and service water for irrigation, geothermal and industrial uses (Job, 2022; Siebert et al., 2010; Stauffer et al., 2013). Furthermore, groundwater ecosystems build the largest terrestrial freshwater biome in the world (Griebler et al., 2014a). This habitat is considered species-rich (>100 000 species) and comprises many endemic taxa (Culver and Holsinger, 1992).
However, human activities and natural changes threaten this habitat in many areas (Becher et al., 2022), putting pressure on its ecosystem and groundwater communities and altering general biogeochemical processes (Griebler et al., 2016). Changes in water quality and water volume are driven by natural processes (Goater, 2009); however, groundwater abstraction for irrigation, drinking water and mining activities changes the groundwater volume and, therefore, groundwater levels (Danielopol et al., 2003; Hancock et al., 2005). Changes in groundwater quality can also be caused by pollutants originating from agriculture (Korbel et al., 2022; Di Lorenzo et al., 2020; Di Lorenzo and Galassi, 2013), urbanisation (Hallam et al., 2008), surface waters (Danielopol et al., 2003; Kristensen et al., 2018), heavy industry (Hose et al., 2014) and thermal pollution (Menberg et al., 2013; Taylor and Stefan, 2009; Tissen et al., 2019; Zhu et al., 2010). Moreover, climate change, which increases groundwater temperatures (Figura et al., 2011; Menberg et al., 2014; Tissen et al., 2019), puts pressure on groundwater and its ecosystems. Those environmental pressures can alter groundwater fauna community structures (Korbel et al., 2022) and, therefore, induce changes in groundwater biodiversity and species dominance (Goater, 2009). Typical implications of this are changes in the natural variation in population cycles; shifts in the community composition, as ubiquitous surface-water species can outcompete and replace groundwater species; and, finally, species extinction (Danielopol et al., 2003). The potential decline or even loss of groundwater communities compromises the functioning of an aquifer and its ecosystem, resulting in the deterioration of groundwater health (Hancock, 2002; Hancock et al., 2005).
To assess groundwater's health or ecological status, schemes for monitoring this ecosystem (i.e. biomonitoring) become increasingly important, as already implemented for surface water (Haase et al., 2023). Biomonitoring is defined as the “use of biological systems (organisms and organism communities) to monitor environmental change over space and/or time” (DIN EN 16413: VDI, 2018). More specifically, by repeatedly or permanently monitoring organisms or organism communities, the quality of a habitat's environment is recorded and assessed and the state of an ecosystem and its dynamics in time and space are evaluated (Mösslacher and Notenboom, 1999; Underwood, 1997). For application in groundwater, two strategies are currently available: “active” and “passive” biomonitoring (Friberg et al., 2011; Mösslacher et al., 2001; Mösslacher and Notenboom, 1999). In active biomonitoring, standardised organisms with a known origin from the laboratory are inserted in groundwater, and their behaviour is then monitored in this habitat (see the biological early-warning system of the GroundCare project in Spengler et al., 2017). In contrast, in passive biomonitoring, bioindicators (wildlife population) are sampled from their natural habitat and their behaviour is examined in the laboratory (Brielmann et al., 2011) or, as in most studies, the faunal communities found are analysed to conclude the prevailing conditions (Fuchs et al., 2006; Hahn and Fuchs, 2009; Korbel and Hose, 2015; Stein et al., 2010; VDI 16413, 2018).
The advantage of biomonitoring compared with monitoring abiotic parameters is that organisms and communities act as remote sensors integrating environmental conditions and stress over their lifetime, undergo quantitative and qualitative alterations, and thus can provide information on medium- to long-term environmental conditions and changes (Conti, 2008). Hence, for most existing biomonitoring programmes, the faunal community composition, different species and taxa, and an abundance of other taxonomic groups are considered (Conti, 2008; Friberg et al., 2011). Macroinvertebrates are often used for biomonitoring, as their taxonomy is well known; moreover, they are sensitive to many stressors and can be sampled easily and repeatedly over specific time periods, which results in more accurate indications of diversity, richness and composition among all groundwater biota (Conti, 2008; Friberg et al., 2011; Lennon, 2019).
Surface waters have been a key focus of aquatic research due to their accessibility and visibility. The assessment of surface waters is typically based on biological, hydromorphological and physicochemical criteria and is defined in detail in the European Water Framework Directive (WFD). Accordingly, there is a large number of studies with long-term data on aquatic surface ecosystems. In this context, a recent study collected 1816 time series from riverine systems in 22 European countries from 1968 to 2020 (714 698 observations) to investigate freshwater biodiversity (Haase et al., 2023). The authors conclude that standardised, long-term and large-scale monitoring can be used to effectively characterise temporal changes in biodiversity and environmental drivers and identify sites at high risk.
A prime example of long-term monitoring in the field is the Swedish national surface water monitoring programme, which began with research on the Mälaren lake in 1964 that aimed to better understand the eutrophication occurring there. This project contributed significantly to understanding the effects of climate change, land use and post-glacial rebound on water quality. Today, the combined programme comprises monitoring of water chemistry and biodiversity in 114 streams and 110 lakes and a probability-sampling programme including 4800 lakes (Fölster et al., 2014). With the advent of the WFD, reference sites from the Swedish monitoring programme were used for the intercalibration of data from northern continental Europe. Data from this monitoring programme “play a key role in past and present national and international environmental commitments including Swedish environmental objectives, critical load assessments, and many aspects of EU legislation, such as the WFD, Habitats Directive, and Nitrate Vulnerable Zone Directive” (Fölster et al., 2014).
However, until now, a lack of repeated and long-term sampling of groundwater fauna has limited the understanding of the basic biology and ecology of many species, and there is a notable absence of analyses of the variation and temporal evolution of groundwater fauna (Koch et al., 2023). Repeated sampling over multiple years can be found in very few locations, mainly in central Europe and Australia (Goater, 2009; Korbel and Hose, 2017; Marmonier et al., 2000; Menció et al., 2014). Goater (2009) analysed groundwater fauna data from an 8-year monitoring programme (1999–2007) at 21 wells in Australia, observing changes in stygofauna species presence, population numbers and community assemblages as well as a shift from an amphipod-dominated to a copepod-dominated system. A second study in Australia conducted four sampling campaigns between 2007 and 2010 at 20 wells to investigate the stream–aquifer relationship (Menció et al., 2014). Further ecological research focused on examining environmental and human influences on the distribution of biota and groundwater ecosystem health. A study in New South Wales at 15 sites with six sampling campaigns revealed only minor variation in ecological conditions between 2007 and 2015 (Korbel and Hose, 2017). Moreover, ecosystem health benchmarks are associated with aquifer typology, rather than applied only to local areas. In Europe, Marmonier et al. (2000) also revealed limited temporal variation in the biodiversity of the interstitial fauna of artificial aquatic systems for 3 successive years (1995–1997). Long-term data on groundwater ecology and chemistry are available across the state of Baden-Württemberg, Germany, from a continuous monitoring programme of 44 sites sampled annually or biannually between 2002 and 2022 (Fuchs, 2007; Fuchs et al., 2006; Stein et al., 2015), making Baden-Württemberg one of the most densely investigated areas worldwide with respect to groundwater fauna – 2026 samples from 950 sites (Koch et al., 2023).
The main objective of this study is to identify changes in groundwater fauna in the German state of Baden-Württemberg over the last few decades at both regional and local scales. We hypothesise that these changes could be related to natural or anthropogenic stress observable through changes in abiotic parameters (such as temperature increase due to climate change and high nitrate concentrations stemming from agricultural fertilisation) and land use changes (such as an increase in surface sealing). To this end, multiple abiotic and biotic groundwater parameters of wells are jointly analysed over time. Individual sites are then scrutinised with respect to small-scale changes. Furthermore, changes in groundwater ecosystems at different spatial scales and the implications of the observed changes for biomonitoring are assessed. Finally, we identify the ecological and physicochemical parameters most suitable for robust biomonitoring.
The following workflow was developed to address our objective (Fig. 1). The available groundwater data from the study site (i.e. the state of Baden-Württemberg) were reviewed, and observation wells for additional sampling in 2020 were selected (Step 1, site selection; Fig. 1). Afterwards, the biotic and abiotic data were temporally and statistically analysed at different spatial scales (Step 2 and 3, local to state-wide analysis and temporal and statistical analyses; Fig. 1). Finally, three individual wells were analysed in detail concerning changes in land use and abiotic parameters (Step 4, local-scale analysis; Fig. 1).
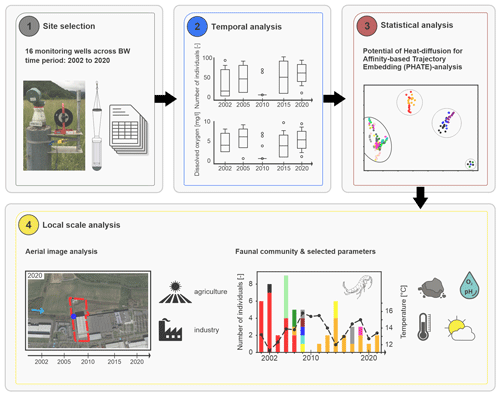
Figure 1Developed workflow including the following four steps: (1) site selection and database review (image source: Fabien Koch, KIT), (2) temporal analysis, (3) statistical analysis, and (4) local-scale analysis (image source: © Google Earth Pro, Google LLC, 2022).
2.1 Site selection
The State Office of Environment, Measurements and Nature Conservation Baden-Württemberg (Landesanstalt für Umwelt, Messungen und Naturschutz Baden-Württemberg, LUBW) maintains an extensive network of up to 2600 groundwater observation wells (Landesanstalt für Umwelt Messungen und Naturschutz Baden-Württemberg, 2013). In addition, the locations of the groundwater level monitoring network and the database of all monitoring sites in Baden-Württemberg are also considered, which results in 50 000 initial sites. Of these, 304 wells have undergone faunal analysis at least twice in the past (Fig. 2; Fuchs, 2007). These 304 sites were selected based on (1) a representative distribution within the different natural areas and aquifer types in Baden-Württemberg and (2) accessibility and the absence of installed measurement devices, pumps, etc. within the wells (Fuchs, 2007). Based on the initial measurement results in 2001/2002, 44 of the 304 sites were selected for annual or biannual sampling between 2006 and 2022 (Stein et al., 2015). Faunal colonisation, a good area- and aquifer-type coverage, and the availability of physicochemical measurements were considered for this selection. Out of these 44 sites, 16 wells were selected for the current study based on the spatial coverage of the study area, aquifer type, land use type, well depth, faunal colonisation during the past 2 decades, availability of time series of physicochemical parameters and an average content of dissolved oxygen higher than 1 mg L−1 (which is a limiting factor for faunal colonisation; Griebler et al., 2014b).
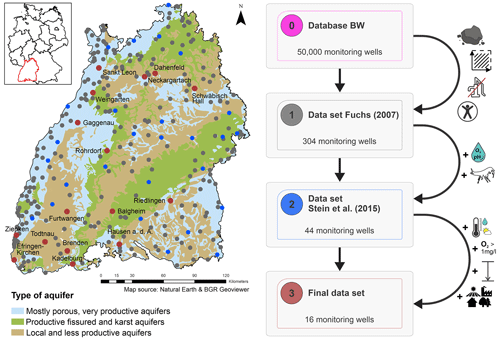
Figure 2The study area (the state of Baden-Württemberg in southwestern Germany) including the aquifer types according to the classification of the Federal Institute for Geosciences and Natural Resources (inset on the left-hand side) and selected monitoring wells according to the selection process (shown on the right-hand side) (Hydrogeologie von Deutschland 1:1 000 000 – Klassifikation gemäß der Standardlegende für Hydrogeologische Karten (HY1000–SLHyM), 2022).
2.2 Groundwater sampling
Faunal groundwater sampling in the 16 selected observation wells took place twice in 2001/2002 (although only data from June–September were used for analysis in this study), annually from 2006 to 2014, and then biannually in August/September until 2020. At the beginning of each sampling campaign, the depths of the groundwater table and of the well were measured using an electrical contact gauge. Afterwards, water standing in the well (i.e. well water) was taken with a bailer (750 mL AquaSampler, Bürkle GmbH, Lörrach) from the bottom of the well. In these samples, oxygen concentrations (IntelliCAL LD0101, luminescence-based optical probe; accuracy: ±0.1 mg L−1), carbonate hardness, pH (IntelliCAL PHC101; accuracy: ±0.002) and electrical conductivity (TDS-measuring instrument Meditech/TenYua) were measured using an HQ40D portable two-channel multimeter (Hach Lang GmbH, 2022). Additional samples were taken to determine the amount of sediment and for further chemical analysis (content of dissolved carbon, organic nitrogen, phosphate, ammonium, orthophosphate and total phosphate; total bacterial count; and total cell count).
The faunal sampling was conducted using a modified Cvetkov net, as described by Hahn and Fuchs (2009). More specifically, a plankton net consisting of a gauze funnel with a mesh size of 73 µm attached to a collection vessel (50 mL centrifuge tube) with a weight was used (Step 1; Fig. 1). With the help of a fishing rod or a winch for larger depths, the net sampler was lowered down to the bottom of the well. To sample as many fauna as possible, the net sampler was quickly raised about 1.5 m and lowered again 10 times. The collected samples were stored in a cooling box at about 8 °C until fixation with 96 % ethanol on the same day. Rose Bengal dye (C20H2Cl4I4Na2O5, Thermo Scientific Chemicals) was added until 2015 and Eosin B (C20H6Br2N2Na2O9) was used from 2015 onwards; these products dye organic matter a pink hue for easier determination of groundwater fauna. Faunal samples were sorted at the taxonomical order level and determined at species level under 10 to 20 times magnification. Based on this determination, further faunal parameters were calculated, such as the total abundance, number of taxa or species, classification into stygofaunal classes (stygoxen, stygophile, or stygobiont), and ecological status according to Griebler et al. (2014b). Limitations regarding the sampling method must be considered when interpreting the faunal results. With the help of a net sampler, only the standing water in a groundwater monitoring well is sampled. This water is affected by filter effects, which can lead to an overrepresentation of larger individuals, such as amphipods (Hahn and Matzke, 2005; Korbel et al., 2017). Larger, more active and sessile organisms, on the other hand, may not be fully captured by sampling using pumps, as they are subject to filter effects, especially in fine sediments. However, studies have shown that the proportion of species and the presence/absence of taxa is similar between the two sampling methods (Hahn and Gutjahr, 2014; Hahn and Matzke, 2005; Korbel et al., 2017).
Hydrochemical groundwater sampling from pumped aquifer water was performed by the LUBW, with measurement results being provided in an annual, publicly accessible online catalogue (Jahresdatenkatalog Grundwasser, 2022). Of the 144 available parameters, 42 are used in this study, in particular, standard cations and anions, heavy metals and inorganic trace substances, pesticides, and aromatic hydrocarbons (Table S1). Parameters with a lack of temporal resolution are excluded. Aquifer water is analysed in situ in the field with probes, in accordance with the LUBW groundwater sampling guidelines and in line with the applicable DIN (Deutsches Institut für Normung) standards for electrical conductivity (DIN EN 27888), water temperature (DIN 38404 4), pH value (DIN EN ISO 10523), oxygen (DIN EN 25814, in situ or using a flow-through system), and acid and base capacity (DIN 38409-7) (Landesanstalt für Umwelt Messungen und Naturschutz Baden-Württemberg, 2013). Laboratory measurements are conducted in an accredited laboratory according to DIN EN ISO/IEC 17025. Annual air temperature data were taken from the Deutscher Wetterdienst (German Meteorological Service; DWD, 2022).
2.3 Statistical analysis
To better understand large-scale spatial relationships and the structure of the high-dimensional groundwater data, a PHATE (Potential of Heat-diffusion for Affinity-based Trajectory Embedding) analysis is conducted (Moon et al., 2019). This method has been shown to provide meaningful results for handling financial data (Grzybowska and Karwański, 2022), prediction of disease outbreaks (Kuchroo et al., 2022), learning of brain activation manifolds (Busch et al., 2022), RNA sequencing (Moon et al., 2019) and investigation of groundwater ecosystems (Koch et al., 2021). In contrast to most existing methods, a PHATE analysis allows one to obtain biologically interesting structures from increasingly large, modern data sets while also accounting for the high level of noise inherent in biological data sets.
PHATE is a dimensionality reduction method that generates a low-dimensional embedding specific for visualisation, in the form of scatter maps. Thus, an accurate, denoised representation of a data set's global and local structures is provided without imposing strong assumptions on the design of the data. The PHATE algorithm computes the pairwise distances from the data matrix. It transforms the distances to affinities to encode local information by applying a Gaussian kernel function to Euclidian distances. Afterwards, the local affinity matrix undergoes row normalisation to get an N×N stochastic matrix. Thus, the normalised kernels form a probability transition matrix. Using diffusion processes, global relationships are learnt and encoded using the potential distance. In detail, the von Neumann entropy is used to determine a diffusion timescale. Markov processes are then used to define the distance between the points in t steps. In the next step, a random walk is performed, and fixed initial conditions are used for the data generation process. The resulting distance is then used for the final step of the algorithm (for more details, the reader is referred to Grzybowska and Karwański, 2022). Metric multidimensional scaling (MDS) embeds the likely distance information into low dimensions for visualisation (Moon et al., 2019). Thus, objects with similar characteristics are close to each other in the final graph. The axis labels PHATE1 and PHATE2 are dimensionless and represent parameter combinations, i.e. distinct axes in the multidimensional parameter space (comparable to similar analyses, e.g. MDS). Another advantage of this method is that biological and abiotic parameters can be considered together, allowing for the identification of further dependency structures. In this study, the analysis is conducted using 15 physical, biotical and (hydro)geological input parameters (Table S2). The selection of parameters is based on previous studies as well as empirical values of influencing factors from the literature (Hahn, 2006; Koch et al., 2021; Korbel et al., 2018; Stein et al., 2012). The PHATE analysis is carried out using a Python code provided by Krishnaswamy Lab on GitHub (see Moon et al., 2019). From each well, data from all annual measurement campaigns are analysed separately to assess temporal variations in faunal communities and abiotic conditions.
3.1 Faunistic overview
Considering the entire investigation period (2002–2020), the faunal colonisation differs across the study area, with between 52 and 1800 individuals and up to 42 different species per well (Fig. 3). The highest abundances in Baden-Württemberg were found in the porous aquifers in the northeastern part of the state and in the southern Upper Rhine Valley, whereas the lowest abundances were found in fractured and karst aquifers as well as in less productive aquifers (Fig. 2). The spatial distribution of the faunal community according to taxonomic groups is shown in Fig. 3b. In the southeast of the state, crustaceans (mainly cyclopoids) dominate. In karst aquifers of the Swabian Alb and the local and less productive fissured aquifers of the southern Black Forest, amphipods are more frequent (e.g. Balgheim, Todtnau and Brenden). Wells in the northern Black Forest and along the Rhine in more productive aquifers are additionally colonised by isopods, harpacticoids, nematodes, ostracodes and/or annelids. Synacrids were found only in wells in the northern part of Baden-Württemberg (except for Kadelburg), in the north of the Upper Rhine Valley and the Neckar River catchment.
3.2 Temporal analysis
From 2002 to 2020, the number of individuals of all 16 monitoring wells ranged between 10 and 50 on average per year, with no observable temporal change in the average total abundance or its variation (Fig. 4a). The same applies to the number of species (Fig. 4b). Moreover, no significant changes were revealed over time for the abiotic parameters (Fig. S1). The year 2007 stands out with a high number of individuals and species (Fig. 4) as well as a low share of stygobiont species. This year also shows a higher dissolved oxygen content and electrical conductivity (Fig. S1). This is particularly true for wells in Efringen-Kirchen, Rohrdorf, Zienken, Schwäbisch Hall, and Gaggenau.
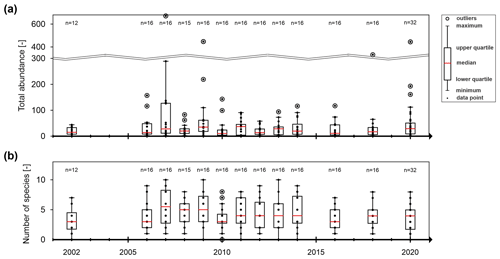
Figure 4Box plots of important biotic parameters between 2002 and 2020 for all 16 wells combined: (a) total abundance and (b) number of sampled species. The dots represent all available data points for each year. “n” indicates the number of measuring points. No sampling was conducted in years with no box plot.
While there is no apparent trend at the regional scale, there are significant variations between individual years for some individual wells (Fig. 5). Regarding the faunal parameters, no single well exhibits a significant positive development concerning faunal abundance or biodiversity. Only the well in Schwäbisch Hall shows a slightly increasing trend in abundance over the last 5 years. This well is characterised by the presence of tardigrades (Tardigrada, light-brown colour in Fig. 3 and in the online data set; Koch et al., 2024), which are typical of small surface water bodies and wells with organic material from the surface (leaves, moss, etc.) (Schminke et al., 2007). Moreover, this well shows a gradual change in the faunal community from one single stygobiont species to multiple stygobiont and stygophile species. On the other hand, there is a clear decrease in the abundance and faunal diversity in Todtnau and Zienken (Fig. 5a, b); in the case of Zienken, this is linked to a decreasing dissolved oxygen content, with <1 mg L−1 in 2014 (Fig. 5e). In Zienken, periods with higher abundances from 2007 to 2009 and from 2013 to 2014 coincided with higher numbers of ubiquitous species from the surface and a higher bacterial count. Moreover, fluctuations in the abundance and number of species are present in several wells, such as in Gaggenau and Efringen-Kirchen (Fig. 5a, b). Besides four stygobiont indicator species in Gaggenau, all other species only appear sporadically. Furthermore, the increase in the oxygen concentration after 2006 in Gaggenau is striking, as is the higher number of oligochaetes and nematodes (Fig. 5c; Koch et al., 2024).
An important parameter for the ecosystem status is the ratio of crustaceans to oligochaetes, which serves as a basis for the ecological assessment scheme of Griebler et al. (2014b). According to this scheme, monitoring wells with more than 70 % crustaceans and less than 20 % oligochaetes have a “natural” status (indicating very good or good ecological conditions). Considering the entire study period, most monitoring wells in this study fall within this category (Fig. 5c).
Abiotic parameters show mostly constant conditions in the individual wells (Fig. 5d, e, f). One notable exception with respect to electrical conductivity is Efringen-Kirchen, where electrical conductivity values decrease over time (Fig. 5f). In terms of well water temperature (Fig. 5d), several sites show an increasing trend, such as Weingarten, Riedlingen and Furtwangen. More pronounced temperature changes are present in Kadelburg, with temperature variations of >5 °C between individual measurements, and Furtwangen, with an increase of 4.6 K between 2002 and 2020.
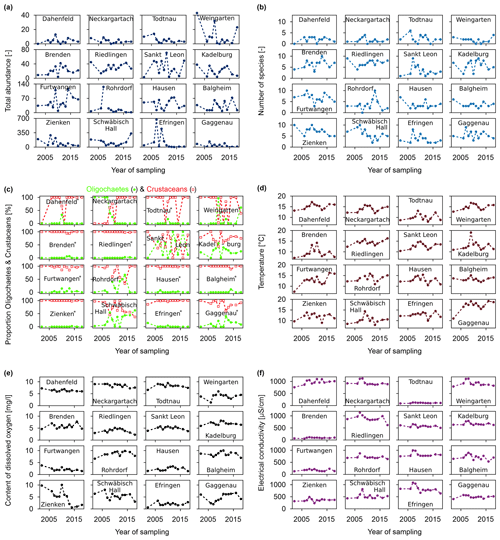
Figure 5Time series of individual monitoring wells for (a) total abundance (please note the different scales on the y axes); (b) number of species; (c) proportion of crustaceans and oligochaetes (* wells with a very good or good ecological status according to the assessment scheme of Griebler et al., 2014b); (d) temperature of the well water; (e) dissolved oxygen content of the well water; and (f) electrical conductivity of the well water.
3.3 Statistical analysis
Correlation analyses are performed using the Spearman rank correlation coefficient to identify potential relationships between faunal and abiotic parameters, such as well configuration, geology and groundwater quality. In total, 164 parameters are considered, 92 of which are biotic parameters (65 taxonomic parameters and 27 other biotic parameters; see Table S4). Moreover, 72 abiotic parameters from the physicochemical parameters monitored by the LUBW are used. Samples are considered significantly correlated if the coefficient is >0.5 or <−0.5.
The correlation coefficients reveal that the dissolved organic carbon (DOC) content of the sampled aquifer water is significantly correlated with the amount of biologically usable sediment (ρ=0.53). Both parameters reflect the food content delivered through surface water input. Moreover, the DOC content is correlated with the number of individuals and species of the class Ostracoda (ρ=0.51; ρ=0.53) and the number of juvenile copepods (ρ=0.51). Furthermore, there are correlations of the measured depth of the well with the abundance of Ostracoda and the total number of species (ρ=0.51; ρ=0.51). Moreover, some statistically significant correlations exist between faunal parameters and several anions and cations. These correlations are not further addressed here, as only limited data are available for those parameters (mercury, strontium, etc.).
A PHATE analysis is conducted to visualise the high-dimensional data set in low dimensions. As can be seen in the visualisation, three distinct groups can be identified (Fig. 6). Group I contains monitoring wells in the karst aquifers of the Muschelkalk and Keuper formations in the northeast of Baden-Württemberg and a fissured aquifer in the southern Black Forest, which generally have a low abundance. In contrast, Group II includes samples in all other wells, with the remaining wells of the southern Black Forest (Brenden and Furtwangen) located at the edge of this group. Group III consists of samples that had no groundwater fauna.
Temporal variations at the individual sites are also noticeable in the PHATE results by observing the spread of samples from individual locations. The sampling of very stable locations (e.g. Todtnau, Dahenfeld, Balgheim and Riedlingen) with small parameter fluctuations over time are spatially more concentrated in the PHATE graph than wells with unstable conditions (e.g. Schwäbisch Hall and Weingarten). Generally, sites with stable conditions with respect to both fauna and hydrochemical parameters (as identified in Table S3) are concentrated in the lower right-hand region of Group II, labelled Subgroup II.a (except for Dahenfeld and Rohrdorf), and the upper left-hand region of Group II, labelled Subgroup II.b (Fig. 6). The categorisation of measurement sites into stable and unstable according to their condition is based on the variance in faunistic and abiotic (hydrogeochemical) parameters over the period under investigation (Table S3). The variance is parameter-specific and depends on natural and seasonal fluctuations, such as groundwater temperature varying by 1–2 °C over the course of a year (Gibert et al., 1994; Taylor and Stefan, 2009). Measurement wells with a total standard deviation over all observed parameters above 14 are classified as unstable wells.
While the affiliation of the wells to a specific group is constant over time (except for the mentioned samples with no fauna), the location of different samples within the group can be associated with concrete changes in specific parameters, specifically abundance, sediment content and temperature. Despite stable overall conditions, the well in Rohrdorf shows one outlier further down in the graph (orange triangle) representing the measurement in 2007 with significantly more individuals (126) than in other years. The amount of sediment is responsible for a clustering of samples from different wells (Hausen, Gaggenau and Weingarten, among others) in the upper left-hand corner of Group II; these samples are from 2009, 2011 and 2012 and lack information about the amount of sediment. The samples from Gaggenau (dark-pink dots) show a further outlier in 2002, located more to the right, which is related to a relatively low sediment content (<1 mL). The same applies to Schwäbisch Hall, which has an outlier (red dot) on the right-hand side of the graph that also contains a low sediment content, little detritus and a low abundance (eight individuals). The samples from Furtwangen (yellow triangles) are also spread over a wide area, with those at the bottom of Subgroup II.a from 2002 and 2020 exhibiting significantly lower temperatures (4.3 and 8.7 °C), which are close to the measured temperatures in Brenden (light pink).
3.4 Local-scale analysis
As described above, certain changes in faunal parameters in individual wells can be related to changes in abiotic parameters, for example, to a decreasing dissolved oxygen content (as in Zienken), whereas other changes, such as the fluctuating ecological status in Neckargartach and Sankt Leon, cannot directly be related to varying abiotic parameters (Fig. 5). Hence, we assess these two unstable wells in more detail with respect to changes to the surface surrounding the wells and compare them to the well in Todtnau, which shows very stable conditions.
Neckargartach
The well in Neckargartach is 35 m deep with filter screens between 27 and 34 m in a fractured and karst aquifer formed by a clay-containing limestone (Fig. 2). The overall number of individuals is very low (maximum of nine individuals per sample) and decreased during the observation period (Fig. 7). Faunal analysis at the species level shows a distinct change in the faunal community and dominant species between 2002 and 2020. Between 2002 and 2007, oligochaetes dominate the faunal community (Fig. 7). Most of these individuals belong to the species Dorydrillus michaelseni, which is only occasionally found in groundwater and is an indicator of slight contamination (Moog, 2002). In 2008 and 2009, the dominant species was Chappuisius inopinus (Crustacea: Harpacticoida), while from 2011 onwards, mainly crustaceans were found, with the rare species Parabathynella badenwuerttembergensis being dominant. The temperature of the sampled well water fluctuated between 11.3 and 15.8 °C, although without a specific trend (see also Fig. 5d). The nitrate content decreased from 51.4 to 25.9 mg L−1 during the study period (Fig. 7), and the dissolved oxygen content decreased slightly (from 10.9 to 8.0 mg L−1) (Figs. 5, S2).
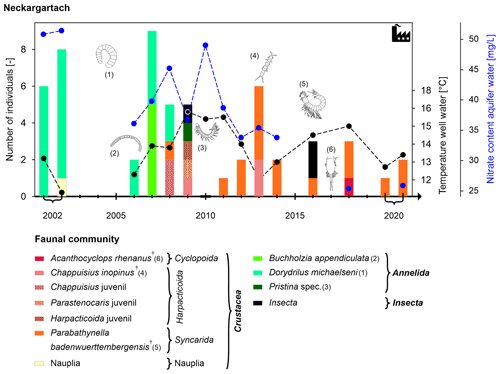
Figure 7Temporal development of the faunal community (abundance and composition of the community; higher taxa are shown in bold and † denotes stygobiont species) and the well water temperature (secondary y axis) at the bottom of the monitoring well in Neckargartach during the period of investigation (2002–2020). No sampling was conducted in years with no bar.
Sankt Leon
The Sankt Leon well is located next to a field path between a forest and an agricultural area near the village of Sankt Leon (Fig. S3a). The well has filter screens between 4 m and the well bottom at 10 m, in the Quaternary glacial sand and gravels of the Upper Rhine Valley (Fig. 2). The well shows unstable abiotic and faunal conditions (between 0 and >400 individuals per sampling; Fig. 5) and significant variations in the faunal composition (Fig. 8).
Common taxa in this well are nematodes and different cyclopoids (Crustacea). In the first decade, rather ubiquitous species colonised the well in large numbers, with Graeteriella unisetigera and Diacyclops languidoides (Crustacea: Cyclopoida) being the dominant species. The latter is one of Germany's most common and widespread groundwater species (Matzke et al., 2009; Schminke et al., 2007). From 2010 onwards, fewer individuals and different taxa were found, with Bathynella freiburgensis (Crustacea: Syncarida) being the dominant species in recent years. Well water temperature showed significant fluctuations between 11 and 14 °C, although without a visible trend. The nitrate content decreased from 70 mg L−1 to below 20 mg L−1 (Fig. 8).
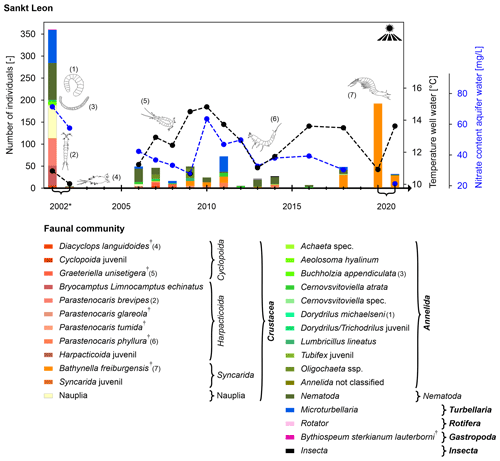
Figure 8Temporal development of the faunal community (abundance and composition of the community; higher taxa are shown in bold and † denotes stygobiont species), the content of nitrate (data from the LUBW, blue y axis on the right) and the well water temperature (black y axis on the right) at the bottom of the well in Sankt Leon between 2002 (* fauna sampling in May and December) and 2020. No sampling was conducted in years with no bar.
Todtnau
The well in Todtnau is also located in an agricultural area near a small stream (Prägbach) and close to the village of Todtnau-Geschwend (Fig. S3b). The well has a depth of 39 m with well screens between 5 and 36 m in a fractured crystalline aquifer in the southern Black Forest. The number of individuals and species is generally low (Fig. 9). The well in Todtnau is colonised by stygobiont species only, except for Collembola (Insecta) in 2 years, which live in the soil and on the water's surface. The most common species over the past 20 years is Crangonyx subterraneus (Crustacea: Amphipoda), which is widespread, ecologically very flexible and inhabits all kinds of underground habitats, although it prefers fractures and gravel (Schminke et al., 2007). The species Proasellus cavaticus (Crustacea: Isopoda) is also commonly present at this location. Individuals of this species can be up to 1 cm long and, therefore, prefer larger cavities (e.g. caves and fractured aquifers), such as the one in Todtnau. Another common species is Niphargus auerbachi (Crustacea: Amphipoda), a comparably big, stygobiont groundwater species. The groundwater species Troglochaetus beranecki (Annelida: Polychaeta) found in 2001, 2007 and 2011 is cold stenotherm, has low water chemistry requirements (Schminke et al., 2007) and also inhabits deep groundwater wells (Matzke et al., 2009). It is also noted that juvenile crustaceans, especially niphargids, are predominant in the last 2 years of sampling. Well water temperatures are generally low due to the higher altitude in the Black Forest. The same applies to the electrical conductivity related to filter screens in crystalline rocks and organic matter content due to the depth of the well.
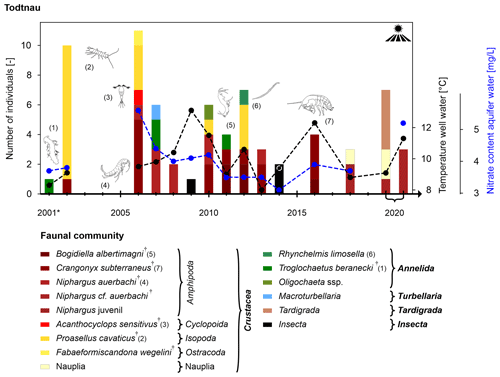
Figure 9Temporal development of the faunal community (abundance and composition of the community; higher taxa are shown in bold and † denotes stygobiont species), the content of nitrate (data from the LUBW, blue y axis on the right) and the well water temperature (black y axis on the right) at the bottom of the well in Todtnau-Geschwend between 2001 (* fauna sampling in November) and 2020. No sampling was conducted in years with no bar.
4.1 Faunistic overview
Overall, there is no regional pattern in the spatial distribution of faunal abundance and biodiversity (Fig. 3). However, there is a visual relationship between abundance and biodiversity, as monitoring wells with many individuals also show more species (and vice versa). Potential reasons for this are a high hydrogeological and hydrochemical heterogeneity in combination with a small number of monitoring wells, the time between sampling, superimposing effects of local influences, and site-specific parameters linked to variations in topography and geology. The spatial distribution of the faunal community aligns with findings regarding distribution patterns of groundwater fauna from previous studies (Fuchs, 2007; Fuchs et al., 2006; Hahn and Fuchs, 2009; Stein et al., 2012, 2015). According to Fuchs et al. (2006), for example, the main distribution focus of syncarids is also in the north of Upper Rhine Valley and in the Neckar River catchment.
4.2 Temporal analysis
There is no observable temporal change in the average total abundance or its variation or in the number of species, indicating that the large-scale biodiversity has remained stable over the past 2 decades (Fig. 4). Moreover, no significant changes are revealed over time for the abiotic parameters, suggesting stable hydrochemical conditions over time. Outliers in individual years, such as in 2007, hint at a more pronounced surface influence and disturbed conditions, particularly in Efringen-Kirchen, Rohrdorf, Zienken, Schwäbisch Hall and Gaggenau.
While there is no apparent trend at the regional scale, there are significant variations between individual years for some individual wells, indicating more complex temporal behaviours at the local scale (Fig. 5). Gradual changes in the faunal community from stygobiont species to multiple stygobiont and stygophile species are potentially linked to surface water influence (Schminke et al., 2007). For example, in Schwäbisch Hall, where the well appears to be intact and no anomalies were found during sampling, it is more likely that changes in the faunal community and abundance are related to the dried-out lakes in the wooded area, but potential contamination cannot be ruled out. Decreasing dissolved oxygen contents, as in Zienken, could be related to microbial oxygen degradation due to a high organic matter input. Furthermore, a strong surface influence in Zienken is linked to periods with higher numbers of ubiquitous species from the surface and a higher bacterial count, as also observed by Stein et al. (2015). Fluctuations in the abundance and number of species in several wells indicate unstable conditions likely caused by varying surface influence. Thus, species appearing only sporadically at one location can indicate an intermittent surface water input into this well, as can be seen in Gaggenau with respect to the increase in oxygen concentration after 2006 and the higher number of oligochaetes and nematodes. These results are consistent with previous studies, which showed that groundwater communities strongly depend on the hydrologic exchange with surface water (Fuchs et al., 2006; Gutjahr et al., 2014; Hose et al., 2015).
The ratio of crustaceans to oligochaetes is an important parameter for the ecosystem status. Only the following six wells have a proportion of oligochaetes >20 % in 1 or multiple years, indicating disturbed conditions: Kadelburg, Schwäbisch Hall, Rohrdorf, Sankt Leon, Weingarten and Neckargartach (Fig. 5c).
While the wells in Kadelburg, Schwäbisch Hall, Rohrdorf and Sankt Leon show a fluctuating ecological status over time, probably related to varying surface water influence, the well in Neckargartach shows a distinct change in the ecological status in 2008 that does not seem to be related to other biotic or abiotic parameters. Abiotic parameters show mostly constant conditions in the individual wells. The increase in electrical conductivity in Efringen-Kirchen is most likely caused by the reconstruction of the well from an underfloor to a surface observation well (see Fig. 5c).
In terms of well water temperature, several sites show an increasing trend, which is in the same range as observed changes in groundwater temperature in previous studies (Figura et al., 2011; Menberg et al., 2014). More pronounced temperature changes are more likely related to varying surface influence or environmental conditions during measurements.
In summary, faunistic and abiotic parameters are mostly constant over time in the individual wells of the study area. A total of 9 out of 16 wells (Dahenfeld, Balgheim, Hausen, Riedlingen, Brenden, Kadelburg, Rohrdorf, Furtwangen and Todtnau) show stable conditions over time with respect to the variance in the faunistic and abiotic parameters (Table S3). In contrast, seven wells (Weingarten, Schwäbisch Hall, Efringen-Kirchen, Zienken, Gaggenau, Neckargartach and Sankt Leon) show higher standard deviations and, therefore, unstable conditions. These are often linked to a varying influence of surface water, which aligns with previous studies (Dole-Olivier, 1998; Foulquier et al., 2011; Stein et al., 2012).
4.3 Statistical analysis
The observed correlation between the DOC and ostracodes can be explained by the fact that individuals of the class Ostracoda rely on a temporary surface influence, as they need nutrients (mainly detritus) from the surface, and are thus typical of the interstitial and porous aquifers (Mösslacher and Hahn, 2003). A positive relationship between depth and the number of ostracodes is also observed in Reeves et al. (2007). Moreover, these findings are consistent with a positive relationship between invertebrate density and dissolved and particulate organic matter found in previous studies (Datry et al., 2005; Hahn, 2006; Mösslacher and Notenboom, 1999). Besides the DOC, no correlations are found between faunal parameters and those reflecting food supply for groundwater ecosystems. Thus, food is not a limiting factor for the occurrence of groundwater fauna in the studied wells. In general, only a few significant correlations were found. This is in line with observations by previous studies in which correlation analysis revealed significant relationships between chemical variables but no or very few and only weak correlations between abiotic and biotic parameters (Dumas et al., 2001; Griebler et al., 2014b; Hahn, 2006; Koch et al., 2021; Schmidt and Hahn, 2012; Steube et al., 2009). A commonly noted reason for this is that ecosystems are complex multivariate systems exposed to multiple stressors simultaneously (Steube et al., 2009).
In the PHATE analysis, the affiliation of the wells to a specific group is generally constant over time, and the clustering of wells with stable conditions confirms the categorisation of the wells using the variance of their faunistic and hydrochemical parameters. In addition, the PHATE analysis shows that biodiversity, illustrated by the number of taxa and individuals, and geological conditions, such as the type of aquifer, have the largest impact on the clustering of the monitoring wells into three distinct groups. Although, the correlation analysis does not indicate any relationship between the amount of sediment and other parameters, except the proportion of biologically usable sediment, a trend in sediment content in the PHATE analysis is visible. This trend is due to very local effects over time in conjunction with other fluctuating parameters.
Previous studies have also shown that hydrogeological parameters strongly influence the occurrence and composition of groundwater fauna (Koch et al., 2021; Stein et al., 2012). Geology and hydrological connectivity significantly influence water chemistry and habitat availability and, therefore, biotic distribution (Hahn, 2006; Fuchs, 2007; Fuchs et al., 2006; Korbel et al., 2018; Korbel and Hose, 2015; Tione et al., 2016). For instance, the abundance and species richness of crustacean fauna in the alluvial aquifers are most related to hydrological conditions, oxygen concentrations and geologic structures (Mösslacher, 1998), which is consistent with our findings. Moreover, this is consistent with the results from Korbel et al. (2018), who state that “sediment size, and thus the size of interstitial voids, is a key limiting factor” for stygofauna.
These findings show that the amount of sediment can be used as an indicator of the pore structure, which determines hydraulic conductivity and living space (Mösslacher, 1998), and can thus be used as a proxy for living conditions. Temperature, on the other hand, can be used as an indicator for surface influence, as it is an indirect marker of the degree of hydrological exchange with the surface (Schönborn, 2003; Hahn, 2006). This was also observed by Koch et al. (2021) at the city scale, where local geology influenced the occurrence of groundwater fauna, the number of individuals and the food supply. Other studies have also observed a significant effect of groundwater temperature on fauna, as diversity decreased with increasing temperature in laboratory and small-scale field studies (see Brielmann et al., 2009; Spengler, 2017).
4.4 Local-scale analysis
Neckargartach
Overall, the observed faunal changes in Neckargartach represent a change from stygophile species dominance to stygobiont species dominance as well as a simultaneous change from oligochaete to crustacean dominance and, thus, to the aforementioned change in the ecological status (Fig. 5c). The observed faunal changes also coincide with alterations in the land use in the surrounding area (Fig. 10). After the first oligochaete-dominant period, a previously unsealed area was first converted into a gravel-covered car park in 2008 and later into an industrial warehouse in 2018. During that time, different crustacean species began to dominate the faunal community (Fig. 10). Surprisingly, this surface change is not reflected in the well water temperatures, even though groundwater under covered surfaces typically shows higher temperatures than under unsealed surfaces (Tissen et al., 2019). In Neckargartach, this is likely linked to groundwater inflow from the adjacent agricultural area to the monitoring well (Lang et al., 2004). The nitrate content decreases, most likely due to the absence or reduction of fertilisation. Moreover, observed changes in the nitrate and oxygen contents are consistent with the shielding from the surface, as the surface is the main source of oxygen, and the change from stygophile to stygobiont species (Bork et al., 2009; Hahn, 2006; Malard et al., 1996; Mösslacher, 1998; Pospisil, 1999; Sket, 1999).
Thus, there is a clear link in Neckargartach between decreasing surface influence, caused by increasing surface sealing, and the dissolved oxygen content and the composition of groundwater ecosystems, as also observed by Korbel et al. (2013). In this study, agriculture in areas with different land uses affects groundwater ecosystems (composition of stygofauna and microbial assemblages) due to changes in groundwater quality (nitrate and phosphorus contents). Hence, higher abundances of cyclopoids, harpacticoids and oligochaetes were found under irrigated areas. However, it has to be mentioned that the improvement in the ecological status according to Griebler et al. (2014b) due to surface sealing is a site-specific observation for Neckargartach. Generally, surface sealing and the related decrease in dissolved oxygen will more likely lead to the deterioration of groundwater ecosystems (Hervant and Malard, 1999; Korbel et al., 2022; Mösslacher, 1998).
Sankt Leon
Although these faunal changes indicate a weakening surface influence over time, no changes in land use or surface conditions were observed (Fig. S3). As Bathynella freiburgensis tolerates a large range of temperatures and is typical of the Upper Rhine Valley (Fuchs, 2007; Spengler, 2017), individuals of that species might have a competitive advantage over other species. High nitrate contents in the first years are most likely linked to intensive agricultural fertilisation, in particular for asparagus cultivation, which is typical of this region. However, these fluctuations do not seem to correlate with the observed faunal shifts. Furthermore, previous studies have shown that a nitrate concentration below 50 mg L−1 has no direct impact on groundwater fauna (Fakher el Abiari et al., 1998; Di Lorenzo et al., 2020; Di Lorenzo and Galassi, 2013; Mösslacher and Notenboom, 1999). Accordingly, more detailed and site-specific investigations (e.g. more accurate, in situ groundwater temperature and dissolved oxygen measurements over depth) would be needed to clarify the drivers of the unstable conditions at Sankt Leon.
Todtnau
In contrast to the two wells discussed previously, the well in Todtnau shows stable conditions (Table S3, Fig. 5). Despite its location in an agricultural area, the nitrate content is below the geogenic background (Fig. 9). However, despite stable physicochemical conditions and no change in land use or surface conditions, certain changes in the faunal community can be observed, as different species occur in different years, although without any visible trends. These are also the samples that show a non-natural status of the ecosystem according to Griebler et al. (2014b) due to the absence of crustaceans (Fig. 5c). The predominance of juvenile crustaceans, especially niphargids, may indicate a recovery of the population after a lack of colonisation in 2014. Years without colonisation may point to a lack of nutrients and, therefore, challenging living conditions. In general, the faunal composition in Todtnau reflects the hydrogeological conditions of the well, with many species typical of large cavities and higher altitudes.
This study analyses the faunal composition and abiotic parameters of 16 groundwater measurement wells in the German state of Baden-Württemberg over the past 2 decades. Statistical analyses are used to distinguish wells with stable and unstable faunal conditions and to assess the impact of specific hydrological and hydrochemical parameters on this characterisation. Time series of individual wells are also discussed in combination with past aerial images to analyse the impact of changes in surface conditions.
Considering the entire study area, we observed no long-term changes or trends in abiotic or faunal parameters, indicating generally stable and ecologically good conditions. However, temporal fluctuations in faunal parameters, such as total abundance and number of species, and thus unstable conditions are observed for 7 out of 16 wells. In some cases, these changes are directly related to gradual changes in abiotic parameters, such as decreasing abundances due to reduced dissolved oxygen contents. However, more often, there are no clear patterns in individual abiotic parameters; instead, superimposing the effects of multiple parameters linked to increasing or weakening surface influence leads to changes in groundwater fauna. Results from a multivariate PHATE analysis confirm these findings and highlight the hydrogeological setting, the content of sediment and detritus in the well and the temperature as influential factors.
Examining faunal changes at the species level for selected wells reveals that unstable conditions can be linked to changes in surface sealing by anthropogenic construction measures, which even changed the ecological status at one specific site. However, variable faunal composition and fluctuating abundances were also observed for sites with no visual changes in land use and surface influence as well as (although this was less obvious) for a deep, well-shielded site with very stable abiotic groundwater conditions. Thus, more long-term studies of groundwater ecology with higher spatial and temporal resolution are necessary to further improve our understanding of faunal shifts over time.
These findings have direct implications for large-scale biomonitoring of groundwater, which is becoming increasingly important. Transferability of local observations to a larger scale is very limited due to small-scale heterogeneities in hydrogeological conditions and the superimposition of site-specific effects. Noticeable environmental changes for wells in Baden-Württemberg were often linked to changes in the dissolved oxygen content, well water temperature and sediment content. Accordingly, these parameters should be accurately and representatively measured in the water column of the well (if possible using depth-resolved measurement) and assessed in combination with hydrogeological and surface conditions to obtain more reliable, representative and robust biomonitoring results. Limitations regarding the sampling method must be also considered when interpreting faunal results. This will help to design management tools for agencies and local authorities.
As has been shown, various parameters, such as surface conditions (built-up areas, underground infrastructure, sealing, etc.), hydrogeological conditions (type of aquifer, size of pore cavities, groundwater flow, etc.), and physicochemical conditions (temperature, content of dissolved oxygen, nutrient supply, etc.) influence the health of the ecosystem. Thus, future assessment schemes should consider a more comprehensive range of indicators. Moreover, future groundwater fauna sampling campaigns should employ shorter sampling intervals (e.g. on a monthly basis) to also address the effects of seasonality. Furthermore, the observed faunal fluctuations in wells in natural, unaffected areas with stable abiotic conditions stress that reference locations for ecological groundwater assessments and biomonitoring have to be carefully selected, ideally using multiyear data. Therefore, reference sites should comprise different settings, including forests, green areas, cities, industrial areas and surface waters, to account for small-scale heterogeneities in hydrogeological conditions and land use. This is crucial for the transferability of findings to larger scales and longer time frames as well as to identify sites at high risk.
Results of the physicochemical, faunistic and statistical analysis are available at https://doi.org/10.35097/m4gepnhuc3yengju (Koch et al., 2024).
The supplement related to this article is available online at: https://doi.org/10.5194/hess-28-4927-2024-supplement.
PB, KM and HJH provided the topic and supervised the work. AF and FK executed the fieldwork. AF evaluated the samples. FK evaluated the collected data, interpreted and visualised the results, and wrote the first draft of the paper. KM, AF, HS, HJH and PB edited the paper.
The contact author has declared that none of the authors has any competing interests.
Publisher’s note: Copernicus Publications remains neutral with regard to jurisdictional claims made in the text, published maps, institutional affiliations, or any other geographical representation in this paper. While Copernicus Publications makes every effort to include appropriate place names, the final responsibility lies with the authors.
The authors are grateful to the State Office of Environment, Measurements and Nature Conservation Baden-Württemberg (LUBW), especially to Klaus-Peter Barufke, for support. Moreover, we would like to thank Cornelia Spengler (IGÖ GmbH) for support at the beginning of the study. Finally, we acknowledge support from the KIT Publication Fund of the Karlsruhe Institute of Technology.
Funding for the present work was provided by the State Graduate Scholarship fund (Landes-Graduierten-Förderung LGF) (awarded to Fabien Koch), the Margarete von Wrangell program of the Ministry for Science and Art (MWK) Baden-Württemberg (awarded to Kathrin Menberg), and the German Federal Environment Foundation (DBU, AZ 3392) within the framework of the “Thermostress” project.
The article processing charges for this open-access publication were covered by the Karlsruhe Institute of Technology (KIT).
This paper was edited by Jan Seibert and reviewed by two anonymous referees.
Becher, J., Englisch, C., Griebler, C., and Bayer, P.: Groundwater fauna downtown – Drivers, impacts and implications for subsurface ecosystems in urban areas, J. Contam. Hydrol., 248, 104021, https://doi.org/10.1016/j.jconhyd.2022.104021, 2022.
Bork, J., Berkhoff, S. E., Bork, S., and Hahn, H. J.: Using subsurface metazoan fauna to indicate groundwater-surface water interactions in the Nakdong River floodplain, South Korea, Hydrogeol. J., 17, 61–75, https://doi.org/10.1007/s10040-008-0374-2, 2009.
Brielmann, H., Griebler, C., Schmidt, S. I., Michel, R., and Lueders, T.: Effects of thermal energy discharge on shallow groundwater ecosystems, FEMS Microbiol. Ecol., 68, 273–286, https://doi.org/10.1111/j.1574-6941.2009.00674.x, 2009.
Brielmann, H., Lueders, T., Schreglmann, K., Ferraro, F., Avramov, M., Hammerl, V., Blum, P., Bayer, P., and Griebler, C.: Oberflächennahe Geothermie und ihre potenziellen Auswirkungen auf Grundwasserökosysteme, Grundwasser, 16, 77–91, https://doi.org/10.1007/s00767-011-0166-9, 2011.
Busch, E. L., Huang, J., Benz, A., Wallenstein, T., Lajoie, G., Wolf, G., Krishnaswamy, S., and Turk-Browne, N. B.: Temporal PHATE: A multi-view manifold learning method for brain state trajectories, bioRxiv [preprint], https://doi.org/10.1101/2022.05.03.490534, 2022.
Conti, M. E.: Biomonitoring of freshwater environment, in: Biological Monitoring: Theory and Applications, vol. 17, WIT Press, Boston, 47–79, https://doi.org/10.2495/978184564002603, 2008.
Culver, D. C. and Holsinger, J. R.: How many species of troglobites are there?, in: Journal of Caves and Karst Studies, vol. 54, edited by: Flurkey, A. J., The National Speleological Society, Lake Mills, 79–80, 1992.
Danielopol, D. L., Griebler, C., Gunatilaka, A., and Notenboom, J.: Present state and future prospects for groundwater ecosystems, Environ. Conserv., 30, 104–130, https://doi.org/10.1017/S0376892903000109, 2003.
Datry, T., Malard, F., and Gibert, J.: Response of invertebrate assemblages to increased groundwater recharge rates in a phreatic aquifer, J. North. Am. Benthol. Soc., 24, 461–477, https://doi.org/10.1899/04-140.1, 2005.
Dole-Olivier, M.-J.: Surface water–groundwater exchanges in three dimensions on a backwater of the Rhône River, Freshw. Biol., 40, 93–109, 1998.
Di Lorenzo, T. and Galassi, D. M. P.: Agricultural impact on Mediterranean alluvial aquifers: Do groundwater communities respond?, Fund. Appl. Limnol., 182, 271–282, https://doi.org/10.1127/1863-9135/2013/0398, 2013.
Di Lorenzo, T., Fiasca, B., Di Cicco, M., and Galassi, D. M. P.: The impact of nitrate on the groundwater assemblages of European unconsolidated aquifers is likely less severe than expected, Environ. Sci. Pollut. R., 28, 11518–11527, https://doi.org/10.1007/s11356-020-11408-5, 2020.
Dumas, P., Bou, C., and Gibert, J.: Groundwater macrocrustaceans as natural indicators of the Ariège alluvial aquifer, Int. Rev. Hydrobiol., 86, 619–633, https://doi.org/10.1002/1522-2632(200110)86:6<619::AID-IROH619>3.0.CO;2-P, 2001.
DWD Climate Data Center (CDC): Raster der Monatsmittel der Lufttemperaturminima (2 m) für Deutschland, Version v19.3, https://cdc.dwd.de/portal/ (last access: 22 May 2022), 2022.
Fakher el Abiari, A., Oulbaz, Z., Yacoubi-Khebiza, M., Coineau, N., and Boutin, C.: Etude expérimentale de la sensibilité comparée de trois crustacés stygobies vis-à-vis de diverses substances toxiques pouvant se rencontrer dans les eaux souterraines, Mem. Biospeol., 25, 167–181, 1998.
Figura, S., Livingstone, D. M., Hoehn, E., and Kipfer, R.: Regime shift in groundwater temperature triggered by the Arctic Oscillation, Geophys. Res. Lett., 38, 1–5, https://doi.org/10.1029/2011GL049749, 2011.
Fölster, J., Johnson, R. K., Futter, M. N., and Wilander, A.: The Swedish monitoring of surface waters: 50 years of adaptive monitoring, Ambio, 43, 3–18, https://doi.org/10.1007/s13280-014-0558-z, 2014.
Foulquier, A., Malard, F., Mermillod-Blondin, F., Montuelle, B., Dolédec, S., Volat, B., and Gibert, J.: Surface Water Linkages Regulate Trophic Interactions in a Groundwater Food Web, Ecosystems, 14, 1339–1353, https://doi.org/10.1007/s10021-011-9484-0, 2011.
Friberg, N., Bonada, N., Bradley, D. C., Dunbar, M. J., Edwards, F. K., Grey, J., Hayes, R. B., Hildrew, A. G., Lamouroux, N., Trimmer, M., and Woodward, G.: Biomonitoring of Human Impacts in Freshwater Ecosystems, The Good, the Bad and the Ugly, 44, 1–68, https://doi.org/10.1016/B978-0-12-374794-5.00001-8, 2011.
Fuchs, A.: Erhebung und Beschreibung der Grundwasserfauna in Baden-Württemberg, Universität Koblenz-Landau, 1–109, 2007.
Fuchs, A., Hahn, H. J., and Barufke, K.-P.: Grundwasser-Überwachungsprogramm – Erhebung und Beschreibung der Grundwasserfauna in Baden-Württemberg, Karlsruhe, 76 pp., 2006.
Gibert, J., Danielopol, D. L., and Stanford, J. A.: Groundwater ecology, Academic Press, San Diego, 571 pp., ISBN 978-0-08-050762-0, 1994.
Goater, S. E.: Are Stygofauna Really Protected In Western Australia?, The University of Western Australia, 186 pp., 2009.
Google LLC.: Google Earth Pro, May 2022.
Griebler, C., Malard, F., and Lefébure, T.: Current developments in groundwater ecology – from biodiversity to ecosystem function and services, Curr. Opin. Biotechnol., 27, 159–167, https://doi.org/10.1016/j.copbio.2014.01.018, 2014a.
Griebler, C., Stein, H., Hahn, H. J., Steube, C., Kellermann, C., Fuchs, A., Berkhoff, S. E., and Brielmann, H.: Entwicklung biologischer Bewertungsmethoden und -kriterien für Grundwasserökosysteme, Umweltbundesamt, Dessau, 153 pp., 2014b.
Griebler, C., Brielmann, H., Haberer, C. M., Kaschuba, S., Kellermann, C., Stumpp, C., Hegler, F., Kuntz, D., Walker-Hertkorn, S., and Lueders, T.: Potential impacts of geothermal energy use and storage of heat on groundwater quality, biodiversity, and ecosystem processes, Environ. Earth Sci., 75, 1–18, https://doi.org/10.1007/s12665-016-6207-z, 2016.
Grzybowska, U. and Karwański, M.: Archetypal Analysis and DEA Model, Their Application on Financial Data and Visualization with PHATE, Entropy, 24, 88, https://doi.org/10.3390/e24010088, 2022.
Gutjahr, S., Schmidt, S. I., and Hahn, H. J.: A proposal for a groundwater habitat classification at local scale, Subterr. Biol., 14, 25–49, https://doi.org/10.3897/subtbiol.14.5429, 2014.
Haase, P., Bowler, D. E., Baker, N. J., Bonada, N., Domisch, S., Garcia Marquez, J. R., Heino, J., Hering, D., Jähnig, S. C., Schmidt-Kloiber, A., Stubbington, R., Altermatt, F., Álvarez-Cabria, M., Amatulli, G., Angeler, D. G., Archambaud-Suard, G., Jorrín, I. A., Aspin, T., Azpiroz, I., Bañares, I., Ortiz, J. B., Bodin, C. L., Bonacina, L., Bottarin, R., Cañedo-Argüelles, M., Csabai, Z., Datry, T., de Eyto, E., Dohet, A., Dörflinger, G., Drohan, E., Eikland, K. A., England, J., Eriksen, T. E., Evtimova, V., Feio, M. J., Ferréol, M., Floury, M., Forcellini, M., Forio, M. A. E., Fornaroli, R., Friberg, N., Fruget, J. F., Georgieva, G., Goethals, P., Graça, M. A. S., Graf, W., House, A., Huttunen, K. L., Jensen, T. C., Johnson, R. K., Jones, J. I., Kiesel, J., Kuglerová, L., Larrañaga, A., Leitner, P., L'Hoste, L., Lizée, M. H., Lorenz, A. W., Maire, A., Arnaiz, J. A. M., McKie, B. G., Millán, A., Monteith, D., Muotka, T., Murphy, J. F., Ozolins, D., Paavola, R., Paril, P., Peñas, F. J., Pilotto, F., Polášek, M., Rasmussen, J. J., Rubio, M., Sánchez-Fernández, D., Sandin, L., Schäfer, R. B., Scotti, A., Shen, L. Q., Skuja, A., Stoll, S., Straka, M., Timm, H., Tyufekchieva, V. G., Tziortzis, I., Uzunov, Y., van der Lee, G. H., Vannevel, R., Varadinova, E., Várbíró, G., Velle, G., Verdonschot, P. F. M., Verdonschot, R. C. M., Vidinova, Y., Wiberg-Larsen, P., and Welti, E. A. R.: The recovery of European freshwater biodiversity has come to a halt, Nature, 620, 582–588, https://doi.org/10.1038/s41586-023-06400-1, 2023.
Hach Lang GmbH: Intellical LDO101 lumineszenzbasierte/optische Sonde für gelösten Sauerstoff, für das Labor, 1 m Kabel & Intellical PHC101 gelgefüllte pH-Elektrode für das Labor, geringer Wartungsbedarf, 1 m Kabel, https://de.hach.com/intellical-ldo101-lumineszenzbasierte-optische-sonde-fur (last access: 23 May 2022), 2022.
Hahn, H. J.: A first approach to a quantitative ecological assessment of groundwater habitats: The GW-Fauna-Index, Limnologica, 36, 119–137, 2006.
Hahn, H. J. and Fuchs, A.: Distribution patterns of groundwater communities across aquifer types in south-western Germany, Freshw. Biol., 54, 848–860, https://doi.org/10.1111/j.1365-2427.2008.02132.x, 2009.
Hahn, H. J. and Gutjahr, S.: Bioindikation im Grundwasser funktioniert – Erwiderung zum Kommentar von T. Scheytt zum Beitrag “ Grundwasserfauna als Indikator für komplexe hydrogeologische Verhältnisse am westlichen Kaiserstuhl” von Gutjahr, S., Bork, J. & Hahn, H. J. in Grundwasser 18, Grundwasser, 19, 215–218, https://doi.org/10.1007/s00767-014-0266-4, 2014.
Hahn, H. J. and Matzke, D.: A comparison of stygofauna communities inside and outside groundwater bores, Limologica, 35, 31–44, 2005.
Hallam, F., Yacoubi-Khebiza, M., Oufdou, K., and Boulanouar, M.: Groundwater quality in an arid area of Morocco: Impact of pollution on the biodiversity and relationships between crustaceans and bacteria of health interest, Environ. Technol., 29, 1179–1189, https://doi.org/10.1080/09593330802180237, 2008.
Hancock, P. J.: Human impacts on the stream-groundwater exchange zone, Environ. Manage., 29, 763–781, https://doi.org/10.1007/s00267-001-0064-5, 2002.
Hancock, P. J., Boulton, A. J., and Humphreys, W. F.: Aquifers and hyporheic zones: Towards an ecological understanding of groundwater, Hydrogeol. J., 13, 98–111, https://doi.org/10.1007/s10040-004-0421-6, 2005.
Hervant, F. and Malard, F.: Oxygen supply and the adaptations of animals in groundwater, Freshw. Biol., 41, 1–30, https://doi.org/10.1046/j.1365-2427.1999.00379.x, 1999.
Hose, G. C., Asmyhr, M. G., Cooper, S. J. B., and Humphreys, W. F.: Down under down under: Austral groundwater life, in: Austral Ark: The State of Wildlife in Australia and New Zealand, edited by: Stow, A., Maclean, N., and Holwell, G. I., Cambridge University Press, Cambridge, 512–536, https://doi.org/10.1017/CBO9781139519960.026, 2014.
Hose, G. C., Sreekanth, J., Barron, O., and Pollino, C.: Stygofauna in Australian groundwater systems: Extent of knowledge, CSIRO, 71 pp., 2015.
Hydrogeologie von Deutschland 1:1.000.000 – Klassifikation gemäß der Standardlegende für Hydrogeologische Karten (HY1000–SLHyM), https://produktcenter.bgr.de/terraCatalog/DetailResult.do?fileIdentifier=f0b8086e-9dc1-402c-9f38-15b9933b5b77, last access: 18 October 2022.
Jahresdatenkatalog Grundwasser: http://jdkgw.lubw.baden-wuerttemberg.de/servlet/is/200/?csrt=11385035495993194480, last access: 18 October 2022.
Job, C. A.: Production, Use, and Sustainability of Groundwater: Groundwater Economics, in: 2nd Edn., CRC Press, 435 pp., ISBN 9780429552793, 2022.
Koch, F., Menberg, K., Schweikert, S., Spengler, C., Hahn, H. J., and Blum, P.: Groundwater fauna in an urban area – natural or affected?, Hydrol. Earth Syst. Sci., 25, 3053–3070, https://doi.org/10.5194/hess-25-3053-2021, 2021.
Koch, F., Blum, P., Korbel, K. L., and Menberg, K.: Global overview on groundwater fauna, Ecohydrology, 17, 1–28, https://doi.org/10.1002/eco.2607, 2023.
Koch, F., Blum, P., Stein, H., Fuchs, A., Hahn, H. J., and Menberg, K.: Dataset: Results of the physical-chemical, faunistic and statistical analysis of groundwater wells in South-West Germany, Temporal shift of groundwater fauna in South-West Germany, Karlsruhe Institute of Technology [data set], https://doi.org/10.35097/m4gepnhuc3yengju, 2024.
Korbel, K. L. and Hose, G. C.: Habitat, water quality, seasonality, or site? Identifying environmental correlates of the distribution of groundwater biota, Freshw. Sci., 34, 329–342, https://doi.org/10.1086/680038, 2015.
Korbel, K. L. and Hose, G. C.: The weighted groundwater health index: Improving the monitoring and management of groundwater resources, Ecol. Indic., 75, 164–181, https://doi.org/10.1016/j.ecolind.2016.11.039, 2017.
Korbel, K. L., Hancock, P. J., Serov, P., Lim, R. P., and Hose, G. C.: Groundwater Ecosystems Vary with Land Use across a Mixed Agricultural Landscape, J. Environ. Qual., 42, 380–390, https://doi.org/10.2134/jeq2012.0018, 2013.
Korbel, K. L., Chariton, A., Stephenson, S., Greenfield, P., and Hose, G. C.: Wells provide a distorted view of life in the aquifer: Implications for sampling, monitoring and assessment of groundwater ecosystems, Sci. Rep., 7, 1–14, https://doi.org/10.1038/srep40702, 2017.
Korbel, K. L., Chariton, A. A., and Hose, G. C.: Biotic distribution within groundwater- is it really unpredictable?, ARPHA Conference Abstracts, 1, 1–2, https://doi.org/10.3897/aca.1.e29806, 2018.
Korbel, K. L., Greenfield, P., and Hose, G. C.: Agricultural practices linked to shifts in groundwater microbial structure and denitrifying bacteria, Sci. Total Environ., 807, 14, 150870, https://doi.org/10.1016/j.scitotenv.2021.150870, 2022.
Kristensen, P., Whalley, C., Nihat Zal, F. N., and Christiansen, T.: European waters: Assessment of status and pressures 2018, Publications Office of the European Union, Copenhagen, 1–373, https://doi.org/10.2800/303664, 2018.
Kuchroo, M., Huang, J., Wong, P., Grenier, J. C., Shung, D., Tong, A., Lucas, C., Klein, J., Burkhardt, D. B., Gigante, S., Godavarthi, A., Rieck, B., Israelow, B., Simonov, M., Mao, T., Oh, J. E., Silva, J., Takahashi, T., Odio, C. D., Casanovas-Massana, A., Fournier, J., Obaid, A., Moore, A., Lu-Culligan, A., Nelson, A., Brito, A., Nunez, A., Martin, A., Wyllie, A. L., Watkins, A., Park, A., Venkataraman, A., Geng, B., Kalinich, C., Vogels, C. B. F., Harden, C., Todeasa, C., Jensen, C., Kim, D., McDonald, D., Shepard, D., Courchaine, E., White, E. B., Song, E., Silva, E., Kudo, E., DeIuliis, G., Wang, H., Rahming, H., Park, H. J., Matos, I., Ott, I. M., Nouws, J., Valdez, J., Fauver, J., Lim, J., Rose, K. A., Anastasio, K., Brower, K., Glick, L., Sharma, L., Sewanan, L., Knaggs, L., Minasyan, M., Batsu, M., Tokuyama, M., Muenker, M. C., Petrone, M., Kuang, M., Nakahata, M., Campbell, M., Linehan, M., Askenase, M. H., Simonov, M., Smolgovsky, M., Grubaugh, N. D., Sonnert, N., Naushad, N., Vijayakumar, P., Lu, P., Earnest, R., Martinello, R., Herbst, R., Datta, R., Handoko, R., Bermejo, S., Lapidus, S., Prophet, S., Bickerton, S., Velazquez, S., Mohanty, S., Alpert, T., Rice, T., Schulz, W., Khoury-Hanold, W., Peng, X., Yang, Y., Cao, Y., Strong, Y., Farhadian, S., Dela Cruz, C. S., Ko, A. I., Hirn, M. J., Wilson, F. P., Hussin, J. G., Wolf, G., Iwasaki, A., and Krishnaswamy, S.: Multiscale PHATE identifies multimodal signatures of COVID-19, Nat. Biotechnol., 40, 681–691, https://doi.org/10.1038/s41587-021-01186-x, 2022.
Landesanstalt für Umwelt Messungen und Naturschutz Baden-Württemberg: Leitfaden Grundwasserprobennahme, LUBW Landesanstalt für Umwelt, Messungen und Naturschutz Baden-Württemberg, 52 pp., 1437-0131, 2013.
Lang, U., Rausch, R., and Gudera, T.: Modellierung der großräumigen Grundwasserströmungsverhältnisse im Bereich der Heilbronner Mulde, https://www.researchgate.net/publication/329542726 (last access: 12 November 2024), 2004.
Lennon, J.: Inter catchment comparisons of groundwater communities from the Lower Murray Darling Basin, Maquarie University, 70 pp., https://figshare.mq.edu.au/articles/thesis/Inter_catchment_comparisons_of_groundwater_communities_from_the_Lower_Murray_Darling_Basin/19440497?file=34539686 (last access: 12 November 2024), 2019.
Malard, F., Plénet, S., and Gibert, J.: The use of Invertebrates in groundwater monitoring: A rising research field, Ground Water Monit. R., 16, 103–113, 1996.
Marmonier, P., Claret, C., and Dole-Olivier, M.-J.: Interstitial fauna in newly-created floodplain canals of a large regulated river, Regul. River, 16, 23–36, 2000.
Matzke, D., Fuchs, A., Berkhoff, S. E., Bork, J., and Hahn, H. J.: Erhebung und Bewertung der Grundwasserfauna Sachsen-Anhalts, Landau in der Pfalz, 100 pp., https://lhw.sachsen-anhalt.de/fileadmin/Bibliothek/Politik_und_Verwaltung/Landesbetriebe/LHW/neu_PDF/5.0_GLD/Dokumente_GLD/Berichte_Dokumente_GW/Abschlussbericht_GW_Fauna_2009.pdf (last access: 12 November 2024), 2009.
Menberg, K., Bayer, P., Zosseder, K., Rumohr, S., and Blum, P.: Subsurface urban heat islands in German cities, Sci. Total Environ., 442, 123–133, https://doi.org/10.1016/j.scitotenv.2012.10.043, 2013.
Menberg, K., Blum, P., Kurylyk, B. L., and Bayer, P.: Observed groundwater temperature response to recent climate change, Hydrol. Earth Syst. Sci., 18, 4453–4466, https://doi.org/10.5194/hess-18-4453-2014, 2014.
Menció, A., Korbel, K. L., and Hose, G. C.: River-aquifer interactions and their relationship to stygofauna assemblages: A case study of the Gwydir River alluvial aquifer (New South Wales, Australia), Sci. Total Environ., 479–480, 292–305, https://doi.org/10.1016/j.scitotenv.2014.02.009, 2014.
Moog, O.: Fauna Aquatica Austriaca Katalog zur autökologischen Einstufung aquatischer Organismen Österreichs, Wasserwirtschaftskataster, Bundesministerium für Land- und Forstwirtschaft, Umwelt und Wasserwirtschaft. Wien, Österreich, Wien, 670 pp., ISBN 3-85 174-044-0, 2002.
Moon, K. R., van Dijk, D., Wang, Z., Gigante, S., Burkhardt, D. B., Chen, W. S., Yim, K., Elzen, A. van den, Hirn, M. J., Coifman, R. R., Ivanova, N. B., Wolf, G., and Krishnaswamy, S.: Visualizing structure and transitions in high-dimensional biological data, Nat. Biotechnol., 37, 1482–1492, https://doi.org/10.1038/s41587-019-0336-3, 2019.
Mösslacher, F.: Subsurface Dwelling Crustaceans as Indicators of Hydrological Conditions, Oxygen Concentrations, and Sediment Structure in an Alluvial Aquifer, Int. Rev. Hydrobiol., 83, 349–364, 1998.
Mösslacher, F. and Hahn, H. J.: Die Fauna, in: Grundwasserökologie, edited by: Griebler, C. and Mösslacher, F., Facultas UTB Verlags- und Buchhandels AG, Wien, 159–204, https://doi.org/10.36198/9783825221119, 2003.
Mösslacher, F. and Notenboom, J.: Groundwater biomonitoring, Environmental Science Forum, 96, 119–140, 1999.
Mösslacher, F., Griebler, C., and Notenboom, J.: Biomonitoring of groundwater systems: Methods, applications and possible indicators among the groundwater biota, in: Groundwater ecology: A tool for management of water resources, edited by: Griebler, C., Danielopol, D. L., Gibert, J., Nachtnebel, H. P., and Notenboom, J., European Communities, 132–170, ISBN 9289416394, 2001.
Pospisil, P.: The Composition of Cyclopoid Assemblages in Ecologically Different Groundwater Habitats of a Danube Riverine Wetland in Austria, Crustaceana, 72, 883–892, 1999.
Reeves, J. M., De Deckker, P., and Halse, S. A.: Groundwater Ostracods from the arid Pilbara region of northwestern Australia: Distribution and water chemistry, Hydrobiologia, 585, 99–118, https://doi.org/10.1007/s10750-007-0632-7, 2007.
Schmidt, S. I. and Hahn, H. J.: What is groundwater and what does this mean to fauna? – An opinion, Limnologica, 42, 1–6, https://doi.org/10.1016/j.limno.2011.08.002, 2012.
Schminke, H. K., Grad, G., Ahlrichs, W., Bartsch, I., Christl, H., Gerecke, R., Martin, P., Rumm, P., and Wägele, J. W.: Grundwasserfauna Deutschlands – Ein Bestimmungswerk: DWA-Themen, 1stt Edn., Deutsche Vereinigung für Wasserwirtschaft, Abwasser und Abfall, Hennef, 628 pp., ISBN 3939057444, 2007.
Schönborn, W.: Lehrbuch der Limnologie, Schwarzbart’sche Verlagsbuchhandlung, Stuttgart, 588 pp., ISBN 3-510-65204-5, 2003.
Siebert, S., Burke, J., Faures, J. M., Frenken, K., Hoogeveen, J., Döll, P., and Portmann, F. T.: Groundwater use for irrigation – a global inventory, Hydrol. Earth Syst. Sci., 14, 1863–1880, https://doi.org/10.5194/hess-14-1863-2010, 2010.
Sket, B.: The nature of biodiversity in hypogean waters and how it is endangered, Biodivers. Conserv., 8, 1319–1338, https://doi.org/10.1023/A:1008916601121, 1999.
Spengler, C.: Die Auswirkungen von anthropogenen Temperaturerhöhungen auf die Crustaceagemeinschaften im Grundwasser, Universität Koblenz-Landau, 226 pp., 2017.
Spengler, C., Gerhardt, A., Rütz, N., Van den Berg-Stein, S., Avramov, M., Wolters, V., Marxsen, J., Griebler, C., and Hahn, H. J.: Faunistische Grundwasserbewertung, Korrespondenz Wasserwirtschaft, 10, 272–279, https://doi.org/10.3243/kwe2017.05.001, 2017.
Stauffer, F., Bayer, P., Blum, P., Giraldo Molina, N., and Kinzelbach, W.: Thermal use of shallow groundwater, 1 st., CRC Press, Boca Raton, 287 pp., https://doi.org/10.1201/b16239, 2013.
Stein, H.: Biologische Bewertung der Grundwasserökosysteme in Deutschland: Untersuchungen zum Auftreten der Fauna auf unterschiedlichen räumlichen Skalen, Universität Koblenz-Landau, 161 pp., https://kola.opus.hbz-nrw.de/frontdoor/deliver/index/docId/740/file/Stein_Dissertation_2012.pdf (last access: 12 November 2024), 2012.
Stein, H., Kellermann, C., Schmidt, S. I., Brielmann, H., Steube, C., Berkhoff, S. E., Fuchs, A., Hahn, H. J., Thulin, B., and Griebler, C.: The potential use of fauna and bacteria as ecological indicators for the assessment of groundwater quality, J. Environ. Monitor., 12, 242–254, https://doi.org/10.1039/b913484k, 2010.
Stein, H., Griebler, C., Berkhoff, S., Matzke, D., Fuchs, A., and Hahn, H. J.: Stygoregions-a promising approach to a bioregional classification of groundwater systems, Sci. Rep., 2, 1–9, https://doi.org/10.1038/srep00673, 2012.
Stein, H., Berkhoff, S. E., Fuchs, A., and Hahn, H. J.: Ökologisches Dauermonitoring an ausgewählten Grundwassermessstellen in Baden-Württemberg, Landau in der Pfalz, Institut für Grundwasserökologie IGÖ GmbH, Landau, 181 pp., 2015.
Steube, C., Richter, S., and Griebler, C.: First attempts towards an integrative concept for the ecological assessment of groundwater ecosystems, Hydrogeol. J., 17, 23–35, https://doi.org/10.1007/s10040-008-0346-6, 2009.
Taylor, C. A. and Stefan, H. G.: Shallow groundwater temperature response to climate change and urbanization, J. Hydrol. (Amst), 375, 601–612, https://doi.org/10.1016/j.jhydrol.2009.07.009, 2009.
Tione, M. L., Bedano, J. C., and Blarasin, M.: Land Use and Hydrogeological Characteristics Influence Groundwater Invertebrate Communities, Water Environ. Res., 88, 756–767, https://doi.org/10.2175/106143016x14609975747162, 2016.
Tissen, C., Benz, S. A., Menberg, K., Bayer, P., and Blum, P.: Groundwater temperature anomalies in central Europe, Environ. Res. Lett., 14, 104012, https://doi.org/10.1088/1748-9326/ab4240, 2019.
Underwood, A. J.: Experiments in ecology and management: Their logical design and interpretation using analysis of variance, Cambridge University Press, 524 pp., ISBN 9780521556965, 1997.
Verein Deutscher Ingenieure e.V. (VDI): VDI 4230 Biological procedures to determine environmental impact (bioindication), Verein Deutscher Ingenieure e.V., Berlin, 88 pp., ISBN 0070224706, 2018.
Zhu, K., Blum, P., Ferguson, G., Balke, K. D., and Bayer, P.: The geothermal potential of urban heat Islands, Environ. Res. Lett., 5, 1–6, https://doi.org/10.1088/1748-9326/6/1/019501, 2010.