the Creative Commons Attribution 4.0 License.
the Creative Commons Attribution 4.0 License.
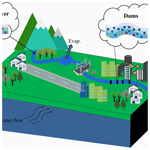
Effects of urbanization on the water cycle in the Shiyang River basin: based on a stable isotope method
Rui Li
Siyu Lu
Liyuan Sang
Gaojia Meng
Longhu Chen
Yinying Jiao
Qinqin Wang
In water-scarce arid areas, the water cycle is affected by urban development and natural river changes, and urbanization has a profound impact on the hydrological system of the basin. Through an ecohydrological observation system established in the Shiyang River basin in the inland arid zone, we studied the impact of urbanization on the water cycle of the basin using isotope methods. The results showed that urbanization significantly changed the water cycle process in the basin and accelerated the rainfall-runoff process due to the increase in urban land area, and the mean residence time (MRT) of river water showed a fluctuating downward trend from upstream to downstream and was shortest in the urban area in the middle reaches, and the MRT was mainly controlled by the landscape characteristics of the basin. In addition, our study showed that river water and groundwater isotope data were progressively enriched from upstream to downstream due to the construction of metropolitan landscape dams, which exacerbated evaporative losses of river water and also strengthened the hydraulic connection between groundwater and river water around the city. Our findings have important implications for local water resource management and urban planning and provide important insights into the hydrologic dynamics of urban areas.
- Article
(5517 KB) - Full-text XML
- BibTeX
- EndNote
According to the “World Cities Report 2020”, urban areas are currently home to more than half of the worldwide people, which amounts to 56.2 %. This pattern is expected to continue over the course of the next decade, culminating in an urbanization rate of 60.4 % by the year 2030. In addition, the study forecasts that by the year 2050, approximately 70 % of the world's population will reside in urban areas (Chen et al., 2020; UN, 2019; UN-Habitat, 2020). Unlike other regions, urban regions have a substantial influence on the hydrological system, resulting in significant consequences on water balance and the water cycle (Gillefalk et al., 2021). To meet the diverse household and industrial requirements in metropolitan areas, where the population is concentrated and water demands are high, a complex interplay between natural and human-made components of the water cycle is required. These components include both natural features such as streams and groundwater and human-made systems like drinking water and drainage networks (Gessner et al., 2014). Urbanization exacerbates water depletion and has far-reaching impacts on groundwater (Flörke et al., 2018; McDonough et al., 2020), affecting the environment and water availability (Bhaskar and Welty, 2015). Rapid urbanization will seriously pressure the structure, function, and water quality degradation of basin ecosystems (Grimm et al., 2008; Sun and Lockaby, 2012; Sun et al., 2015).
Urbanization's effects on basin hydrology and the related processes have complex and varying consequences (Caldwell et al., 2012; Martin et al., 2017). In the past few decades, with the continuous acceleration of urbanization, human activities in urban areas have become more frequent, and the hydrological effects of urbanization have become more intense, attracting widespread attention worldwide (Salvadore et al., 2015). The rise of impervious rivers in urbanized regions increases the rate of urban water runoff, which raises the danger of urban floods (Wing et al., 2018). In addition, high-intensity human activities have led to increased discharge of domestic sewage and industrial wastewater, deteriorating water quality and the ecological environment (Pickett et al., 2011). Meanwhile, basin water cycle processes are influenced by a combination of meteorological and sub-river factors. It has been found that urbanization has led to significant increases in runoff and peak flows in rivers (Liu et al., 2018; Han et al., 2022) and has resulted in shorter runoff response times (Anderson et al., 2022), which also exacerbates the intensity and frequency of flooding in basins (De Niel and Willems, 2019; Blum et al., 2020). On the other hand, the urbanization process leads to an increase in the amount of rainfall in the basin as well as an increase in the frequency of extreme rainfall events (Shastri et al., 2015; Fu et al., 2019; Yang et al., 2021), whereas in dryland inland river basins in arid zones that are dependent on water resources for development, the impacts of urbanization on the water cycle processes of the basins are still not clear, and they need to be explored in depth the effects of urbanization on basin water cycle processes. Hence, study into how human activities alter the features of river runoff and the water cycle within a basin is essential for the prudent use and sustainable development of water resources.
Stable isotopes of hydrogen and oxygen are very useful tools for investigating hydrological issues that are connected to river water and groundwater sources (Fekete et al., 2006; Förstel and Hützen, 1983; Vystavna et al., 2021). Researchers have been conducting studies using stable isotopes as tracers over the course of the past few years in order to explore the impact that urbanization has had on the water cycle. Urbanization has the potential to trigger and intensify convective activity and warm-season rainfall in both urban areas and their surrounding regions (Burian and Shepherd, 2005). Researchers generally agree that urbanization reduces depressions on the underlying river, weakens water permeability, and increases runoff. At the same time, the lower roughness of the underlying river shortens the confluence time (Guan et al., 2015; Oudin et al., 2018). Moreover, against the backdrop of swift urbanization, the swift proliferation of urban regions has resulted in a sharp surge in impermeable areas, alterations to regional microclimates, and the erection of a vast number of infrastructures (including overpasses and subways), all of which have significantly impacted the water cycle process in urban areas (Jacobson, 2011; Westra et al., 2014). The complex connection between the permeable and impermeable zones influences the river confluence processes (Bruwier et al., 2020). The construction of urban water conservation projects, such as rubber dams and pumping stations, also affects the confluence process of urban areas to a certain extent (Zhu et al., 2021). Limited long-term and continuous monitoring has hampered accurate depiction of urbanization's spatiotemporal effects on basin hydrology. Furthermore, scientific research still lacks sufficient research on arid regions that heavily depend on mountain river runoff for sustenance and development.
Against the background of increasing urbanization, it is particularly important to study the hydrological impacts of urbanization on basins and their corresponding countermeasures, especially in arid inland river basins, where the impacts of human activities in urban areas on rivers may be more prominent. Therefore, the Shiyang River (SYR) basin, located in the inland arid zone of northwest China, was used as an example to study the impact of urbanization on the hydrology of the basin using the stable isotope method. The following problems are proposed to be solved: (1) an examination of the mechanisms underlying evaporation and infiltration of river water within urban aquatic ecosystems, (2) assessment of the effects of urbanization on water body connectivity through a comprehensive analysis, and (3) analysis of the influence of urbanization on the precipitation-runoff process. This provides us with essential information on how to maintain and manage the water resources found in inland river basins, which is especially useful in light of the fact that the rate of urbanization is growing.
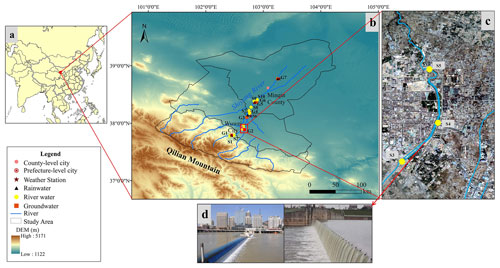
Figure 1(a) The location of the study area. (b) Comprehensive observation system for the study area. (c) Urban river water sampling points (from © Google Maps). (d) Common urban landscape dams in the SYR basin.
2.1 Study area
The SYR basin is located in Gansu Province, China, to the east of the Hexi Corridor. The SYR basin is bounded to the west by the Wushaoling Mountain and to the north by the foothills of the Qilian Mountains (Zhu et al., 2019). The basin in question is situated within the continental temperate belt, characterized by a parched climate and diverse topography. Annual precipitation hovers within the range of 100 to 600 mm, while pan evaporation levels exhibit greater variability, ranging from 700 to 2600 mm annually. The majesty of the Qilian Mountains is where the SYR begins its journey, and the Qilian Mountains are the source of its eight main tributaries. The SYR is principally supported by the convergence of precipitation, snowmelt, and glacier runoff (Wei et al., 2013).
The city of Wuwei is crossed by four important rivers, namely the Xiying, Zamu, Huangyang, and Jinta, which cover a catchment area of 3986 km2. As the principal water source for the entire region, the SYR basin is one of the most highly utilized inland river basins in terms of water resource development and consumption worldwide. The dams in the SYR basin are predominantly situated in close proximity to the urbanized regions of Liangzhou District, located within Wuwei. Liangzhou District, situated in the middle of the basin, boasts of a relatively high population density and a notable commercial concentration. At the turn of the millennium, Wuwei only boasted a paltry five landscape dams positioned on its rivers. As of 2019, this figure has surged dramatically, with a staggering total of 51 urban landscape dams now gracing both urban and peri-urban areas of the city. These dams are primarily composed of human-made landscape waterfalls and rubber dams, fulfilling their core function of creating public landscape water bodies within the urban expanse (Zhu et al., 2021).
2.2 Sampling and data analysis
Since 2017, a comprehensive observation system has been established in the SYR basin, and stable isotope observations and hydrometeorological observations have been carried out on river water, shallow groundwater and rainfall. Continuous sampling in the SYR basin was carried out from April 2017 to March 2021. Different water bodies were sampled, and we collected a total of 846 samples from 24 sampling points (Table 1). The river sampling location ought to be selected such that it is physically possible to go as close to the middle of the river as possible, with the goal of minimizing the impact of areas with standing water and sewage. Artesian well water was collected as groundwater samples at seven sampling locations around the basin. The automated weather station was used to measure meteorological factors such as temperature and relative humidity while collecting precipitation samples. Water samples were sealed in high-density polyethylene bottles to avoid evaporation and leakage during transit and storage, and precipitation samples were collected using weather station standard rain gauges. These samples were then frozen and wrapped with plastic tape.
Analysis of the water samples is conducted through liquid water isotope analysis utilizing the DLT-100 (Los Gatos Research, USA) in the Stable Isotope Laboratory at Northwest Normal University. Each water sample and isotope standard are injected six times in succession to ensure reliable findings, with the first two injection values eliminated and the average of the last four injections used for final analysis, thereby avoiding any potential isotope analysis memory effect. The isotope measurements were denoted by the symbol “δ,” which indicates the deviation in thousandths from the Vienna Standard Mean Ocean Water (VSMOW):
where Rs is the ratio of or in the collected sample, is the ratio of or of the Vienna standard sample, and the analytical accuracy of δD and δ18O is ±0.6 ‰ and ±0.2 ‰, respectively.
3.1 Calculation and indication of d-excess
Dansgaard (1964) introduced the concept of d-excess as the difference in isotopic composition between global precipitation and the Vienna Standard Mean Ocean Water (VSMOW) reference water, which corresponds to a value of 10 %. This parameter reflects the average isotopic composition of air masses associated with precipitation and is widely used to identify atmospheric source regions (Deng et al., 2016). d-excess was proposed by Dansgaard (Dansgaard, 1964) and is defined as
3.2 Calculation of evaporation losses of river water
The losses of river water through evaporation and the resulting fluctuations in water levels of rivers, lakes, and wetlands are key aspects of the terrestrial water cycle that merit significant attention (Gammons et al., 2006; Hamilton et al., 2005). Evaporation is the primary mechanism of water losses in the water cycle. For river water in dry regions and urban river water that flows slowly due to human-made constraints, evaporation cannot be ignored. Thus, it is vital to address the alteration of urban landscape dam water caused by non-equilibrium isotope fractionation during evaporation. Equation (3) can be used to estimate the rate of evaporative water losses from the body of water in question (Skrzypek et al., 2015):
The variables in the equation are as follows: f represents the ratio of water lost to evaporation; δ denotes the measured values of the water body located in the urban dam area of Wuwei, situated in the middle reaches of the SYR; and δ0 represents the initial value of the hydrogen and oxygen stable isotope of the water body. It is widely assumed that the point of intersection between the local meteoric water line (LMWL) and the local evaporation line (LEL) represents the average isotopic composition of the input water body within the basin (Gibson et al., 2005). In the current investigation, the intersection point marked by δ18O = −7.24 ‰ and δD = −46.9 ‰ has been designated as the δ0 value, while δ∗ denotes the maximum isotope enrichment factor and m corresponds to the enrichment slope. The calculation of the above parameters in this paper is realized in Hydrocalculator software (Skrzypek et al., 2015) (http://hydrocalculator.gskrzypek.com, last access: 7 November 2023). According to studies (Qian et al., 2007), it is more accurate to use δ18O when calculating the evaporation loss ratio, so this study calculates the f value of SYR water using δ18O values.
3.3 Periodic regression analysis and the mean residence time (MRT)
Seasonal fluctuations in δ18O values were analysed using periodic regression analysis to determine how these values changed over time. This method entailed fitting seasonal sine wave curves to annual δ18O variations using least-squares optimization (Rodgers et al., 2005):
The modelled δ18O values and the mean weighted annual measured δ18Oave values were both utilized in the analysis of seasonal fluctuations in δ18O levels. Additionally, the measured δ18O annual amplitude (A), the radial frequency of annual fluctuations (c), and the time in days after the start of the sampling period (t) were also considered in this analysis. Furthermore, the phase lag or time of the annual peak δ18O in radians (θ) was determined through this approach.
An exponential model was used for the purpose of estimating the mean residence time (MRT). This model operates on the presumption that precipitation inputs quickly mix with resident water. In order to do this, the following equation was used (Małoszewski et al., 1983; Rodgers et al., 2005):
The amplitude of precipitation (AZ1), the amplitude of the river water outputs (AZ2), and the radial frequency of the annual fluctuation (c) as defined in Eq. (4) were taken into consideration to estimate the MRT.
4.1 Spatiotemporal distribution of isotopes in different water bodies
The isotope values of the river water in the SYR basin show a clear enrichment from upstream to downstream when viewed from space. It is worth noting that landscape dams and reservoirs in urban areas alter this pattern significantly, producing markedly higher isotopic compositions of river water around such structures (Fig. 2). To be more specific, the river water throughout the entire basin had average isotope values that were lower than those of the sampling points in the dam region, which had values that were greater (Table 2). In addition, the dams slowed the flow of the river, and this resulted in isotope enrichment of the river water. Notably, these values exhibit spatial and temporal variability, with the largest δD and δ18O values observed in river water and the lowest in groundwater.
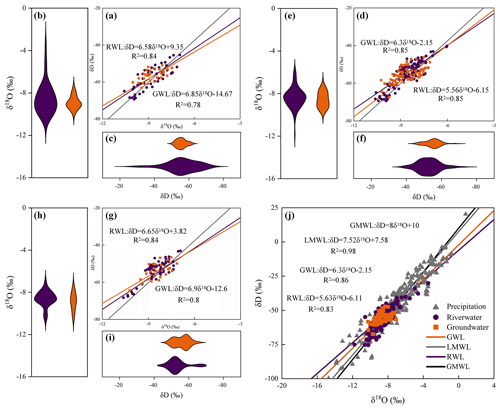
Figure 3Relationship between δD and δ18O in various water bodies in the SYR basin during different seasons (a, d, and g represent spring, summer, and autumn; j represents the comparison of RWL, GWL, LMWL, and GMWL during the entire sampling period; b and c, e and f, and h and i represent the distribution of δD and δ18O in river water and groundwater in spring, summer, and autumn).
To be more specific, over the course of time, these values shift seasonally from spring to autumn (Table 2, Fig. 3). There was a range of values from −75.43 ‰ to −40.62 ‰ for the δD values of river water, with an average of −53.53 ‰. The δ18O values display a varied range, from −10.43 ‰ to −5.53 ‰, with an average of −8.54 ‰, whereas the d-excess values demonstrate variability ranging from 10.26 ‰ to 29.72 ‰, with 15.28 ‰ as the average value. A broad spectrum of δD values is observed during the summer season, ranging from −61.27 ‰ to −31.16 ‰, with an average of −48.90 ‰. Meanwhile, δ18O values fluctuate between −9.52 ‰ and −3.41 ‰, with an average −8.12 ‰. The phenomenon that was observed can be traced back primarily to the aftereffects of the Hongyashan Reservoir built downstream. Because the reservoir has such a large capacity for water retention, it causes significant amounts of river water to evaporate in summer, which ultimately results in a discernible enrichment of the isotopic composition. In both river water and groundwater, δD and δ18O showed significant seasonal variations (Fig. 3). Seasonal variations were more pronounced in river water than in groundwater, with river water showing the largest amplitude in spring and the smallest amplitude in fall, while groundwater showed closer amplitudes in all seasons, which also indicates that groundwater is less disturbed.
4.2 The relationship between δD and δ18O values
As shown by the linear fitting equation δD = 7.52δ18O + 7.58, there is a significant linear positive correlation (R2 = 0.96) between δD and δ18O in atmospheric precipitation in the SYR basin (Fig. 3). It is clear that the slope (7.52) and intercept (7.58) of the local meteoric water line (LMWL) are smaller than the global meteoric water line (GMWL), which can be attributed to the basin's location in an inland arid region, where precipitation disturbances are less frequent and evaporative fractionation of precipitation is stronger. On the other hand, compared with the slopes of the LMWL, the slopes of the river water line (RWL) and the groundwater line (GWL) are relatively close (Fig. 3), indicating that there is a strong hydraulic connection between groundwater and river water in the SYR basin, and the slopes of GWL and RWL show GWL > RWL in all seasons, suggesting that the river water is most affected by evaporation and groundwater is less affected by evaporation. In addition, both river water and groundwater sampling points were distributed near the LMWL, indicating that both river water and groundwater receive recharge from precipitation. Overall, the H–O isotopic composition of river water samples from the SYR showed a linear regression of δD = 5.63δ18O − 6.11, and the slope of RWL was the largest in the autumn (slope = 6.65) and the smallest in the summer (slope = 5.56), which indicated that the river water evaporated the weakest in the autumn and the strongest in the summer.
Isotopic analysis of groundwater samples reveals a range of δD and δ18O values spanning from −50.7 ‰ to −71.9 ‰ and from −7.23 ‰ to −10.4 ‰, respectively. Moreover, the groundwater samples analysed in the study displayed a linear regression of δD = 6.3δ18O − 2.15 (R2 = 0.86). And it is interesting to note that groundwater also shows significant enrichment near the urban landscape dams (Fig. 2), indicating that groundwater is also affected by evaporation, mainly because the Wuwei urban area is in the region of a large alluvial fan in front of the mountains, the sand and gravel aquifers are very permeable, and the depth of groundwater burial is shallow, making the groundwater more susceptible to the effects of evaporation.
4.3 Impact of urbanization on groundwater
We compared monthly variations in isotopic values of groundwater near the city with monthly variations in river water from a landscaped dam and found that the monthly variations in groundwater near the city were closely related to river water from a landscaped dam. The concentration of groundwater sampling sites near the city near the sampling sites of the dam water indicates that the groundwater around the city has similar isotopic signatures to the dam and river water (Fig. 4a). This suggests that groundwater near the city is recharged by river water during the summer months. In addition, we demonstrated this by comparing the data of the dam river water with the groundwater level (Fig. 4). In addition, a portion of the groundwater sampling sites around the city are located in the lower-right corner of the LMWL, which suggests that the groundwater around the city may also experience some degree of evaporation.
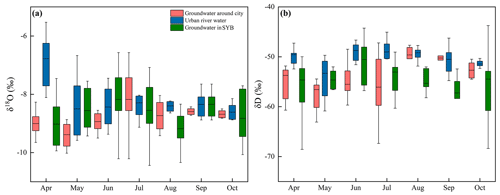
Figure 5(a) Monthly variations of δ18O in urban river water and groundwater around the city. (b) Monthly variations of δD in urban river water and groundwater around the city.
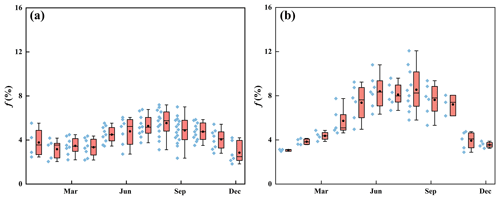
Figure 6Evaporation losses from river water in different areas of the SYR (a) Upper-reach mountainous area. (b) Middle-reach urban areas.
In addition, we also compared and analysed the changes of groundwater isotope values with those of groundwater around the city in the whole basin and found that there was a close correlation between the changes of groundwater around the city and those of the river, while the other groundwater isotope values did not have a strong correlation with the river (Fig. 5). In the urban area, the mean values of δD and δ18O of the dammed river water were −7.49 ‰ and −48.31 ‰, respectively, while the mean values of δD and δ18O of the groundwater around the city were −8.44 ‰ and −50.36 ‰, respectively, which indicated that the δD and δ18O values of the groundwater around the city were similar to those of the river water in the dammed city. In addition, the isotopic mean values of δD and δ18O of groundwater throughout the SYR basin were −8.73 ‰ and −54.78 ‰, which are significantly different from the isotopic values of river water in the urban dam.
4.4 Temporal and spatial variation of river water evaporation losses in the urban area of Wuwei
In addition to being an essential part of the hydrological cycle, evaporation is widely recognized as one of the most significant factors driving climate change in semi-arid regions and in telluric ecosystems (Gibson et al., 2002; Gibson and Edwards, 2002). An obviously spatial and temporal fluctuation can be seen in the amount of river water that is lost to evaporation in the upper mountain area as well as the intermediate urban area of the SYR basin (Fig. 6). Analysed from a time-varying perspective, there is significant seasonal variation in river water evaporation losses, both in the upstream mountainous region and the midstream urban area of Wuwei, with the highest rates occurring during summer and the lowest during winter (Fig. 6). Additionally, a spatial comparison reveals that river water evaporation losses in the midstream urban area of Wuwei are significantly greater than those in the upstream mountainous area.
Differences contributing to evaporation losses from the river in the upstream and midstream urban areas can be explained mainly by the landscape characteristics of the basin. In the upstream of the SYR, higher vegetation cover and atmospheric humidity in the mountainous areas result in weaker evaporation losses, while the midstream areas are dominated by urban land, and urban landscapes increase the watershed area and slow down the river, exacerbating evaporation losses from the river.
5.1 Effects of urbanization on the rainfall-runoff process
Figure 7 depicts the regression model of rainfall events in the SYR basin, represented by a sine wave, and the fitting of river water δ18O across the research season. The δ18O levels of precipitation reported in the SYR basin have an excellent regularity (R2 = 0.46) and a seasonal pattern trend that effectively depicts the influence of the monsoon climate on the local environment (Zhu et al., 2019). Seasonal variations are seen in the generally steady δ18O and δ18O values of the upstream water. These results indicate that the predominant component of the river water is the baseflow resulting from recent precipitation runoff. Throughout the duration of the study, the majority of the lowest δ18O values in the 10 river water sample points were recorded during the winter, whilst the highest values were recorded during the summer. These trends coincide with both the temporal variation of precipitation isotopes in the SYR basin, indicating that precipitation input is the underlying cause of isotope changes in river water. Nevertheless, variations in the isotopes of river water differ in range across various regions within the SYR basin, with significant variation in the degree of fit for the regression curve. The fitting degree of river water in the upper and lower reaches is relatively low (R2 = 0.37, R2 = 0.28, R2 = 0.23), implying limited seasonal isotopic variability in these regions. The midstream river water exhibits a notably higher degree of conformity as compared to its upstream and downstream counterparts (R2 = 0.38, R2 = 0.48, R2 = 0.62, R2 = 0.78, R2 = 0.54, R2 = 0.48, R2 = 0.52). Moreover, the isotopic composition of river water throughout this area exhibits notable cyclic variations.
The reasons for differences in isotope periodicity in different regions may be attributed to local water management systems, topographic features, and urban development. At points S1, S2, and S10, the correlation of model simulations was low, which could be attributed to the presence of Xiying Reservoir in the upstream as well as Hongyashan Reservoir in the downstream (Sang et al., 2023), where seasonal variations in the isotope values of the river water are interfered by the reservoir dispatching activities. At points S3–S5, the correlation of the model simulation is higher, which is because in the middle reaches of the SYR basin, the expansion of urban built-up areas leads to a significant increase in river runoff during the rainy season, and according to the land use data, the land area of the towns in Wuwei has continued to increase by 134.38 km2 from 2010 to 2018, resulting in the river water showing a cyclical trend comparable to that of the precipitation. Since the 1950s, in order to better utilize water resources, 13 small- and medium-sized reservoirs with a total storage capacity of 900 000 m3 have been constructed (Ma et al., 2010), increasing the proportion of rainfall in the runoff constituents as a result of the correlation of the model simulation is at a high level at points S6–S9, where, in contrast to the high-elevation areas in the upper reaches, the terrain in the middle and lower reaches of the SYR basin is relatively flat, mainly with cultivated land and deserts, and is less disturbed by human activities (Sun et al., 2021), which further reflects the responsiveness to recent precipitation inputs.
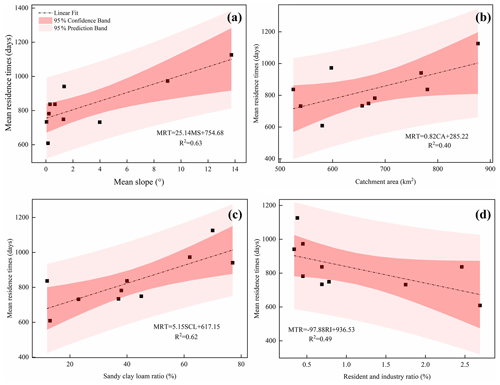
Figure 8Correlation between MRT and (a) mean slope of the basin, (b) catchment area, (c) sand clay loam ratio, and (d) percentage area of residential and industrial use in the basin, with 95 % Confidence and Prediction bands.
Dunnett's test revealed a significant difference (P < 0.05) between the MRT of the river and the annual magnitude of δ18O of the river. We further investigated the relationship between the estimated mean residence time and basin landscape features such as topography (Fig. 8). Using the digital elevation model (DEM) to calculate the mean slope of the SYR basin, we found that the mean residence time was also strongly correlated with the mean basin slope (R2 = 0.63) and that the upper reaches of the SYR basin are mainly high-elevation mountainous areas, where the topography is sloped, but where the vegetation cover is high and dominated by alpine meadows, subalpine scrub, and Qinghai spruce (Zhang et al. 2023), the greater slope leads to a higher gravitational potential, which tends to result in a negative correlation with mean residence time (McGuire et al., 2005), which also contributes to the potentially higher MRT values in the upstream mountains. In our study, catchment area (CA) had a low correlation with MRT (R2 = 0.40), and a weak relationship between catchment area and MRT has been observed in other studies (McGlynn et al., 2003; McGuire et al., 2005).
Soil is an important component of basin hydrology, and the physical properties of soil, such as water-holding capacity and pore space distribution, have an important influence on the response to precipitation in the basin, and the sand–clay–loam soil ratio is used here to investigate the possible relationship with MRT. The results showed that the content of sand–clay–loam ratio showed a strong positive correlation with MRT (R2 = 0.62). Wuwei is located in the pre-mountain flood-fan belt, and the soil is dominated by sandy soil (Zhang et al., 2023), which is loose in texture, has good permeability and good water retention properties, and is mainly used for agricultural cultivation. Its good permeability increases the vertical movement of water and the length of flow paths, leading to a longer MRT. There is a strong negative correlation between the MRT and the ratios of resident and industrial areas (RI) (R2 = 0.49), which also indicates that as urbanization progresses, with the increase in urban land, this undoubtedly leads to a significant shortening of the MRT. However, the MRT in the mid-river urban area is not much shorter as compared to the downstream, which may be attributed to the fact that the large number of landscape dams constructed in the urban areas, currently 51 urban landscape dams, have been built in the peri-urban areas of Wuwei, and the considerable number of landscape dams may have counteracted the impact of the urban land use, resulting in a lengthening of the MRT in the middle reaches as well.
5.2 Effects of water conservancy projects in urban areas on isotope dynamics
Recent studies have suggested that the development of dam–reservoir systems may result in river fragmentation and modifications in flow regimes in terms of their volume, frequency, and duration (Négrel et al., 2016; Murgulet et al., 2016; Peñas and Barquín, 2019; Maavara et al., 2020). Furthermore, chemical-containing nutrient migration, such as phosphorus, may occur during sediment movement, resulting in widespread eutrophication problems (Yang et al., 2007; Duan et al., 2019). As of 2019, a total of 51 urban landscape dams, primarily consisting of artificial landscape waterfalls and rubber dams, have been constructed in and around Wuwei (Zhu et al., 2021). On the metropolitan coast of Wuwei, many landscape dams have led to isotopic enrichment in river water. This damping effect has been observed in numerous dammed rivers across the globe, including the Rio Grande in the southwestern United States (Vitvar et al., 2007) and the Ebro River in Spain (Négrel et al., 2016), as evidenced by isotopic tracers. In the metropolitan coast of Wuwei, a number of landscape dams have led to the enrichment of isotopic tracers in the river water. The results indicate that the δD and δ18O levels of the river water at the outflow of Wuwei are greater than those at the inflow (Fig. 2). Moreover, the influence of evaporation on isotopic composition should not be overlooked, as it can lead to a decrease in d-excess values (Peng et al., 2012). Consistent with previous studies (Wang et al., 2019), we observed that the d-excess of influent water was higher than that of urban river water (Fig. 9). This observation further supports the accumulation of heavy H–O isotopes in the river waters of the dam areas, as shown in Fig. 9a. In contrast, due to the confluence of tributaries prior to the S7 sampling point, the river water has lower isotopic values, resulting in elevated d-excess values between S6 and S8.
5.3 Effects of urbanization on the water cycle of basins
Localized microclimates in urban areas allow for changes in precipitation and evapotranspiration processes, while urbanization alters the pristine sub-river, complicating water cycle processes in the basin (Jacobson, 2011; Westra et al., 2014; Oudin et al., 2018). In terms of the impact on runoff, it is mainly reflected in the increase in river impermeability due to urbanization; the land use area of Wuwei urban land increased by about 134.38 km2 from 2010 to 2018, which greatly weakened the infiltration process in urban areas, and the rainfall runoff process simulated by the sinusoidal cyclic regression method showed that there were significant differences in the river metro in different parts of the SYR basin and that the middle reaches of the river had the highest degree of urbanization, and the time of the metro was the shortest, which further increases the contribution of rainfall to runoff. Regarding the effect of urbanization on evapotranspiration, a large number of dams were constructed on the SYR and flowed through the urban area of Wuwei, causing significant evapotranspiration losses; in addition, these landscape dams also led to hydrogen and oxygen isotope enrichment. Numerous reservoirs were constructed for the construction and development of the city (Ma et al., 2010), and these reservoirs also contributed to a significant evapotranspiration loss effect, which has been previously confirmed in our study was also confirmed (Sang et al., 2023). On the other hand, our study found that the isotopic compositions and trends of urban nearshore groundwater were similar to those of river water, which suggests that there is a close correlation between urban nearshore groundwater and river water and that the difference in water levels between river water and groundwater may be the main reason for river recharge of urban nearshore groundwater (Fig. 4d). In the rainy season, the river level gradually rises, which decreases the difference between the water levels of urban nearshore groundwater and river water, and the river water recharges the groundwater. In the dry season, the river level decreases, and the urban nearshore groundwater, which is buried at a shallow depth, in turn recharges the river.
In addition, the growth of urbanization has had a dramatic impact on the water environment in cities, where water problems occur frequently (Giri and Qiu, 2016; Ma et al., 2022). Urbanization has increased impervious rivers such as parking lots, rooftops, roads, and sidewalks, leading to increased runoff, which creates additional pathways for pollutants to be transported from landscapes to water bodies (Ren et al., 2014; Wilson and Weng, 2010; Nolan et al., 2023). On the other hand, agricultural activities have increased some of the fertilizers, pesticides, herbicides, and dairy manure that move in the farmland into the nearest water bodies, which can directly and indirectly affect and reduce water quality (Yu et al., 2013). The SYR basin in the Northwest Arid Zone is an inland river basin with the highest development intensity and the sharpest conflict between water supply and demand in the region. The Liangzhou district in the central part of the SYR basin is the most densely populated artificial oasis, with the largest scale of water demand in the entire basin. Our previous study found that direct discharge of industrial and community domestic wastewater into the river led to deterioration of river water quality around the SYR basin (Ma et al., 2021). In addition agricultural activities have less impact on the upper reaches of the SYR and relatively more impact on the middle and lower reaches, and the application of nitrogen-based fertilizers during agricultural cultivation is the main cause of high NH4+ and NO3− concentrations in the area (Ma et al., 2021), which may also lead to increased salinity and accelerated eutrophication of the river, threatening the safety of the basin's water environment. Overall, human activities (urbanization) may alter the water cycle processes inherent in inland river basins, and the implications of such changes need to be further explored.
In this study, we investigated the hydrometeorological and isotopic data of the Shiyang River basin from 2017 to 2021, and our investigations showed that urbanization had a significant impact on the water cycle of the basin. The results showed that the isotopic values of the river water showed a significant enrichment from upstream to downstream, but facilities such as landscape dams and reservoirs in the urban area significantly altered this natural pattern, and the isotopic values of the river water in the urban area (δD = −48.31 ‰; δ18O = −7.49 ‰) were higher than those of the natural river water (δD = −55.77 ‰; δ18O = −8.98 ‰). Landscape dams aggravated the evaporation losses of river water, due to the increase in urban land area, which accelerated the rainfall-runoff conversion process; the residence time of river water in different regions of the Shiyang River basin had obvious differences; and the MRT from the upstream to the downstream showed a fluctuating downward process, which was shortened from 1126 d in the upstream to 941 d in the downstream, and the MRT was mainly controlled by the basin's landscape features. In addition, there was a strong relationship between the isotopic composition of the reservoir and the surrounding groundwater. Overall, urbanization has a profound impact on the hydrological system of the basin, and the results of this study can provide some references for future research on urbanization and the water cycle and improve our understanding of the hydrological processes of basin in arid zones.
The isotopic data that support the findings of this study are openly available in Zhu (2022), “Stable water isotope monitoring network of different water bodies in Shiyang River basin, a typical arid river in China”, Mendeley Data, V1, https://doi.org/10.17632/vhm44t74sy.1. The source of soil data is the Harmonized World Soil Database (HWSD), constructed by the Food and Agriculture Organization of the United Nations (FAO) and the International Institute for Applied Systems (IIASA) in 2009. The land use and land cover change data of the Shiyang River basin were obtained from the Data Center of Resources and Environmental Science, Chinese Academy of Sciences (http://www.resdc.cn, last access: 7 November 2023.).
RL: writing (original draft preparation); GZ: writing (reviewing and editing); SL: methodology; LS and GM: data processing and experiment; LC and YJ: methodology and visualization; QW: visualization.
The contact author has declared that none of the authors has any competing interests.
Publisher's note: Copernicus Publications remains neutral with regard to jurisdictional claims made in the text, published maps, institutional affiliations, or any other geographical representation in this paper. While Copernicus Publications makes every effort to include appropriate place names, the final responsibility lies with the authors.
We thank the editor, Nadia Ursino, and the three anonymous reviewers for providing a list of critical and very valuable comments that helped to improve the manuscript.
This research was financially supported by the National Natural Science Foundation of China (grant nos. 42371040, 41971036), the Key Natural Science Foundation of Gansu Province (grant no. 23JRRA698), the Key Research and Development Program of Gansu Province (grant no. 22YF7NA122), the Cultivation Program of Major key projects of Northwest Normal University (grant no. NWNU-LKZD-202302), and the Oasis Scientific Research achievements Breakthrough Action Plan Project of Northwest Normal University (grant no. NWNU-LZKX-202303).
This paper was edited by Nadia Ursino and reviewed by three anonymous referees.
Anderson, B. J., Slater, L. J., Dadson, S. J., Blum, A. G., and Prosdocimi, I.: Statistical Attribution of the Influence of Urban and Tree Cover Change on Streamflow: A Comparison of Large Sample Statistical Approaches, Water Resour. Res., 58, e2021WR030742, https://doi.org/10.1029/2021WR030742, 2022.
Bhaskar, A. S. and Welty, C.: Analysis of subriver storage and streamflow generation in urban basins, Water Resour. Res., 51, 1493–1513, https://doi.org/10.1002/2014WR015607, 2015.
Blum, A. G., Ferraro, P. J., Archfield, S. A., and Ryberg, K. R.: Causal Effect of Impervious Cover on Annual Flood Magnitude for the United States, Geophys. Res. Lett., 47, e2019GL086480, https://doi.org/10.1029/2019GL086480, 2020.
Bruwier, M., Maravat, C., Mustafa, A., Teller, J., Pirotton, M., Erpicum, S., Archambeau, P., and Dewals, B.: Influence of urban forms on river flow in urban pluvial flooding, J. Hydrol., 582, 124493, https://doi.org/10.1016/j.jhydrol.2019.124493, 2020.
Burian, S. J. and Shepherd, J. M.: Effect of urbanization on the diurnal rainfall pattern in Houston, Hydrol. Process., 19, 1089–1103, https://doi.org/10.1002/hyp.5647, 2005.
Caldwell, P. V., Sun, G., McNulty, S. G., Cohen, E. C., and Moore Myers, J. A.: Impacts of impervious cover, water withdrawals, and climate change on river flows in the conterminous US, Hydrol. Earth Syst. Sci., 16, 2839–2857, https://doi.org/10.5194/hess-16-2839-2012, 2012.
Chen, G., Li, X., Liu, X., Chen, Y., Liang, X., Leng, J., Xu, X., Liao, W., Qiu, Y., Wu, Q., and Huang, K.: Global projections of future urban land expansion under shared socioeconomic pathways, Nat. Commun., 11, 537, https://doi.org/10.1038/s41467-020-14386-x, 2020.
Dansgaard, W.: Stable isotopes in precipitation, Tellus, 16, 436–468, https://doi.org/10.1111/j.2153-3490.1964.tb00181.x, 1964.
De Niel, J. and Willems, P.: Climate or land cover variations: what is driving observed changes in river peak flows? A data-based attribution study, Hydrol. Earth Syst. Sci., 23, 871–882, https://doi.org/10.5194/hess-23-871-2019, 2019.
Deng, K., Yang, S., Lian, E., Li, C., Yang, C., and Wei, H.: Three Gorges Dam alters the Changjiang (Yangtze) river water cycle in the dry seasons: Evidence from H-O isotopes, Sci. Total Environ., 562, 89–97, https://doi.org/10.1016/j.scitotenv.2016.03.213, 2016.
Duan, W., Hanasaki, N., Shiogama, H., Chen, Y., Zou, S., Nover, D., Zhou, B., and Wang, Y.: Evaluation and Future Projection of Chinese Precipitation Extremes Using Large Ensemble High-Resolution Climate Simulations, J. Climate, 32, 2169–2183, https://doi.org/10.1175/JCLI-D-18-0465.1, 2019.
Fekete, B. M., Gibson, J. J., Aggarwal, P., and Vörösmarty, C. J.: Application of isotope tracers in continental scale hydrological modeling, J. Hydrol., 330, 444–456, https://doi.org/10.1016/j.jhydrol.2006.04.029, 2006.
Flörke, M., Schneider, C., and McDonald, R. I.: Water competition between cities and agriculture driven by climate change and urban growth, Nat. Sustain., 1, 51–58, https://doi.org/10.1038/s41893-017-0006-8, 2018.
Förstel, H. and Hützen, H.: Oxygen isotope ratios in German groundwater, Nature, 304, 614–616, https://doi.org/10.1038/304614a0, 1983.
Fu, X., Yang, X., and Sun, X.: Spatial and Diurnal Variations of Summer Hourly Rainfall Over Three Super City Clusters in Eastern China and Their Possible Link to the Urbanization, J. Geophys. Res.-Atmos., 124, 5445–5462, https://doi.org/10.1029/2019JD030474, 2019.
Gammons, C. H., Poulson, S. R., Pellicori, D. A., Reed, P. J., Roesler, A. J., and Petrescu, E. M.: The hydrogen and oxygen isotopic composition of precipitation, evaporated mine water, and river water in Montana, USA, J. Hydrol., 328, 319–330, https://doi.org/10.1016/j.jhydrol.2005.12.005, 2006.
Gessner, M. O., Hinkelmann, R., Nützmann, G., Jekel, M., Singer, G., Lewandowski, J., Nehls, T., and Barjenbruch, M.: Urban water interfaces, J. Hydrol., 514, 226–232, https://doi.org/10.1016/j.jhydrol.2014.04.021, 2014.
Gibson, J. J. and Edwards, T. W. D.: Regional water balance trends and evaporation‐transpiration partitioning from a stable isotope survey of lakes in northern Canada, Global Biogeochem. Cy., 16, 10-11–10-14, https://doi.org/10.1029/2001GB001839, 2002.
Gibson, J. J., Prepas, E. E., and McEachern, P.: Quantitative comparison of lake throughflow, residency, and catchment runoff using stable isotopes: modelling and results from a regional survey of Boreal lakes, J. Hydrol., 262, 128–144, https://doi.org/10.1016/S0022-1694(02)00022-7, 2002.
Gibson, J. J., Edwards, T. W. D., Birks, S. J., St Amour, N. A., Buhay, W. M., McEachern, P., Wolfe, B. B., and Peters, D. L.: Progress in isotope tracer hydrology in Canada, Hydrol. Process., 19, 303–327, https://doi.org/10.1002/hyp.5766, 2005.
Gillefalk, M., Tetzlaff, D., Hinkelmann, R., Kuhlemann, L.-M., Smith, A., Meier, F., Maneta, M. P., and Soulsby, C.: Quantifying the effects of urban green space on water partitioning and ages using an isotope-based ecohydrological model, Hydrol. Earth Syst. Sci., 25, 3635–3652, https://doi.org/10.5194/hess-25-3635-2021, 2021.
Giri, S. and Qiu, Z.: Understanding the relationship of land uses and water quality in Twenty First Century: A review, J. Environ. Manage., 173, 41–48, https://doi.org/10.1016/j.jenvman.2016.02.029, 2016.
Grimm, N. B., Faeth, S. H., Golubiewski, N. E., Redman, C. L., Wu, J., Bai, X., and Briggs, J. M.: Global Change and the Ecology of Cities, Science, 319, 756–760, https://doi.org/10.1126/science.1150195, 2008.
Guan, M., Sillanpää, N., and Koivusalo, H.: Storm runoff response to rainfall pattern, magnitude and urbanization in a developing urban catchment, Hydrol. Proc., 30, 543–557, https://doi.org/10.1002/hyp.10624, 2016.
Hamilton, S. K., Bunn, S. E., Thoms, M. C., and Marshall, J. C.: Persistence of aquatic refugia between flow pulses in a dryland river system(Cooper Creek, Australia), Limnol. Oceanogr., 50, 743–754, https://doi.org/10.4319/lo.2005.50.3.0743, 2005.
Han, S., Slater, L., Wilby, R. L., and Faulkner, D.: Contribution of urbanisation to non-stationary river flow in the UK, J. Hydrol., 613, 128417, https://doi.org/10.1016/j.jhydrol.2022.128417, 2022.
Jacobson, C. R.: Identification and quantification of the hydrological impacts of imperviousness in urban catchments: A review, J. Environ. Manage., 92, 1438–1448, https://doi.org/10.1016/j.jenvman.2011.01.018, 2011.
Liu, J., Shen, Z., and Chen, L.: Assessing how spatial variations of land use pattern affect water quality across a typical urbanized basin in Beijing, China, Landscape Urban Plan., 176, 51–63, https://doi.org/10.1016/j.landurbplan.2018.04.006, 2018.
Ma, H., Zhu, G., Zhang, Y., Sang, L., Wan, Q., Zhang, Z., Xu, Y., and Qiu, D.: Ion migration process and influencing factors in inland river basin of arid area in China: a case study of Shiyang River Basin, Environ. Sci. Pollut. R., 28, 56305–56318, https://doi.org/10.1007/s11356-021-14484-3, 2021.
Ma, J., Pan, F., Chen, L., Edmunds, W. M., Ding, Z., He, J., Zhou, K., and Huang, T.: Isotopic and geochemical evidence of recharge sources and water quality in the Quaternary aquifer beneath Jinchang city, NW China, Appl. Geochem., 25, 996–1007, https://doi.org/10.1016/j.apgeochem.2010.04.006, 2010.
Ma, X., Li, N., Yang, H., and Li, Y.: Exploring the relationship between urbanization and water environment based on coupling analysis in Nanjing, East China, Environ. Sci. Pollut. R., 29, 4654–4667, https://doi.org/10.1007/s11356-021-15161-1, 2022.
Maavara, T., Chen, Q., Van Meter, K., Brown, L. E., Zhang, J., Ni, J., and Zarfl, C.: River dam impacts on biogeochemical cycling, Nat. Rev. Earth. Environ., 1, 103–116, https://doi.org/10.1038/s43017-019-0019-0, 2020.
Małoszewski, P., Rauert, W., Stichler, W., and Herrmann, A.: Application of flow models in an alpine catchment area using tritium and deuterium data, J. Hydrol., 66, 319–330, https://doi.org/10.1016/0022-1694(83)90193-2, 1983.
Martin, K. L., Hwang, T., Vose, J. M., Coulston, J. W., Wear, D. N., Miles, B., and Band, L. E.: basin impacts of climate and land use changes depend on magnitude and land use context, Ecohydrology, 10, e1870, https://doi.org/10.1002/eco.1870, 2017.
McDonough, L. K., Santos, I. R., Andersen, M. S., O'Carroll, D. M., Rutlidge, H., Meredith, K., Oudone, P., Bridgeman, J., Gooddy, D. C., Sorensen, J. P. R., Lapworth, D. J., MacDonald, A. M., Ward, J., and Baker, A.: Changes in global groundwater organic carbon driven by climate change and urbanization, Nat. Commun., 11, 1279, https://doi.org/10.1038/s41467-020-14946-1, 2020.
McGlynn, B., McDonnell, J., Stewart, M., and Seibert, J.: On the relationships between catchment scale and streamwater mean residence time, Hydrol. Process., 17, 175–181, https://doi.org/10.1002/hyp.5085, 2003.
McGuire, K. J., McDonnell, J. J., Weiler, M., Kendall, C., McGlynn, B. L., Welker, J. M., and Seibert, J.: The role of topography on catchment‐scale water residence time, Water Resour. Res., 41, 2004WR003657, https://doi.org/10.1029/2004WR003657, 2005.
Murgulet, D., Murgulet, V., Spalt, N., Douglas, A., and Hay, R. G.: Impact of hydrological alterations on river-groundwater exchange and water quality in a semi-arid area: Nueces River, Texas, Sci. Total Environ., 572, 595–607, https://doi.org/10.1016/j.scitotenv.2016.07.198, 2016.
Négrel, P., Petelet-Giraud, E., and Millot, R.: Tracing water cycle in regulated basin using stable δ18O–δ2H isotopes: The Ebro river basin (Spain), Chem. Geol., 422, 71–81, https://doi.org/10.1016/j.chemgeo.2015.12.009, 2016.
Nolan, T. M., Reynolds, L. J., Sala-Comorera, L., Martin, N. A., Stephens, J. H., O'Hare, G. M. P., O'Sullivan, J. J., and Meijer, W. G.: Land use as a critical determinant of faecal and antimicrobial resistance gene pollution in riverine systems, Sci. Total Environ., 871, 162052, https://doi.org/10.1016/j.scitotenv.2023.162052, 2023.
Oudin, L., Salavati, B., Furusho-Percot, C., Ribstein, P., and Saadi, M.: Hydrological impacts of urbanization at the catchment scale, J. Hydrol., 559, 774–786, https://doi.org/10.1016/j.jhydrol.2018.02.064, 2018.
Peñas, F. J. and Barquín, J.: Assessment of large-scale patterns of hydrological alteration caused by dams, J. Hydrol., 572, 706–718, https://doi.org/10.1016/j.jhydrol.2019.03.056, 2019.
Peng, T.-R., Huang, C.-C., Wang, C.-H., Liu, T.-K., Lu, W.-C., and Chen, K.-Y.: Using oxygen, hydrogen, and tritium isotopes to assess pond water's contribution to groundwater and local precipitation in the pediment tableland areas of northwestern Taiwan, J. Hydrol., 450–451, 105–116, https://doi.org/10.1016/j.jhydrol.2012.05.021, 2012.
Pickett, S. T. A., Cadenasso, M. L., Grove, J. M., Boone, C. G., Groffman, P. M., Irwin, E., Kaushal, S. S., Marshall, V., McGrath, B. P., Nilon, C. H., Pouyat, R. V., Szlavecz, K., Troy, A., and Warren, P.: Urban ecological systems: Scientific foundations and a decade of progress, J. Environ. Manage., 92, 331–362, https://doi.org/10.1016/j.jenvman.2010.08.022, 2011.
Qian, H., Dou, Y., Li, X. J., Yang, B. C., and Zhao, Z. H.: Changes of δ18O and δD along Dousitu River and its indication of river water evaporation, Hydrogeol. Eng. Geol., 34, 107–112, https://doi.org/10.3969/j.issn.1000-3665.2007.01.024, 2007.
Ren, L., Cui, E., and Sun, H.: Temporal and spatial variations in the relationship between urbanization and water quality, Environ. Sci. Pollut. R., 21, 13646–13655, https://doi.org/10.1007/s11356-014-3242-8, 2014.
Rodgers, P., Soulsby, C., Waldron, S., and Tetzlaff, D.: Using stable isotope tracers to assess hydrological flow paths, residence times and landscape influences in a nested mesoscale catchment, Hydrol. Earth Syst. Sci., 9, 139–155, https://doi.org/10.5194/hess-9-139-2005, 2005.
Salvadore, E., Bronders, J., and Batelaan, O.: Hydrological modelling of urbanized catchments: A review and future directions, J. Hydrol., 529, 62–81, https://doi.org/10.1016/j.jhydrol.2015.06.028, 2015.
Sang, L., Zhu, G., Xu, Y., Sun, Z., Zhang, Z., and Tong, H.: Effects of Agricultural Large-And Medium-Sized Reservoirs on Hydrologic Processes in the Arid Shiyang River Basin, Northwest China, Water Resour. Res., 59, e2022WR033519, https://doi.org/10.1029/2022WR033519, 2023.
Shastri, H., Paul, S., Ghosh, S., and Karmakar, S.: Impacts of urbanization on Indian summer monsoon rainfall extremes, J. Geophys. Res.-Atmos., 120, 496–516, https://doi.org/10.1002/2014JD022061, 2015.
Skrzypek, G., Mydłowski, A., Dogramaci, S., Hedley, P., Gibson, J. J., and Grierson, P. F.: Estimation of evaporative loss based on the stable isotope composition of water using Hydrocalculator, J. Hydrol., 523, 781–789, https://doi.org/10.1016/j.jhydrol.2015.02.010, 2015.
Sun, G. and Lockaby, B. G.: Water Quantity and Quality at the Urban-Rural Interface, in: Urban-Rural Interfaces, edited by: Laband, D. N., Lockaby, B. G., and Zipperer, W. C., American Society of Agronomy, Soil Science Society of America, Crop Science Society of America, Inc., Madison, WI, USA, 29–48, https://doi.org/10.2136/2012.urban-rural.c3, 2012.
Sun, G., Caldwell, P. V., and McNulty, S. G.: Modelling the potential role of forest thinning in maintaining water supplies under a changing climate across the conterminous United States: Response of Water Yield to Forest Thinning and Climate Change, Hydrol. Process., 29, 5016–5030, https://doi.org/10.1002/hyp.10469, 2015.
Sun, Z., Zhu, G., Zhang, Z., Xu, Y., Yong, L., Wan, Q., Ma, H., Sang, L., and Liu, Y.: Identifying river water evaporation loss of inland river basin based on evaporation enrichment model, Hydrol. Process., 35, e14093, https://doi.org/10.1002/hyp.14093, 2021.
UN-Habitat: World cities report 2020: the value of sustainable urbanization, UN-Habitat, Nairobi, Kenya, 377 pp., 2020.
United Nations Department of Economic and Social Affairs: World Urbanization Prospects 2018: Highlights, United Nations, https://doi.org/10.18356/6255ead2-en, 2019.
Vitvar, T., Aggarwal, P. K., and Herczeg, A. L.: Global network is launched to monitor isotopes in rivers, Eos T. Am. Geophys. Un., 88, 325–326, https://doi.org/10.1029/2007EO330001, 2007.
Vystavna, Y., Harjung, A., Monteiro, L. R., Matiatos, I., and Wassenaar, L. I.: Stable isotopes in global lakes integrate catchment and climatic controls on evaporation, Nat. Commun., 12, 7224, https://doi.org/10.1038/s41467-021-27569-x, 2021.
Wang, B., Zhang, H., Liang, X., Li, X., and Wang, F.: Cumulative effects of cascade dams on river water cycle: Evidence from hydrogen and oxygen isotopes, J. Hydrol., 568, 604–610, https://doi.org/10.1016/j.jhydrol.2018.11.016, 2019.
Wei, W., Shi, P., Zhou, J., Feng, H., Wang, X., and Wang, X.: Environmental suitability evaluation for human settlements in an arid inland river basin: A case study of the Shiyang River Basin, J. Geogr. Sci., 23, 331–343, https://doi.org/10.1007/s11442-013-1013-y, 2013.
Westra, S., Fowler, H. J., Evans, J. P., Alexander, L. V., Berg, P., Johnson, F., Kendon, E. J., Lenderink, G., and Roberts, N. M.: Future changes to the intensity and frequency of short-duration extreme rainfal, Rev. Geophys., 52, 522–555, https://doi.org/10.1002/2014RG000464, 2014.
Wilson, C. and Weng, Q.: Assessing river Water Quality and Its Relation with Urban Land Cover Changes in the Lake Calumet Area, Greater Chicago, Environ. Manage., 45, 1096–1111, https://doi.org/10.1007/s00267-010-9482-6, 2010.
Wing, O. E. J., Bates, P. D., Smith, A. M., Sampson, C. C., Johnson, K. A., Fargione, J., and Morefield, P.: Estimates of present and future flood risk in the conterminous United States, Environ. Res. Lett., 13, 034023, https://doi.org/10.1088/1748-9326/aaac65, 2018.
Yang, L., Ni, G., Tian, F., and Niyogi, D.: Urbanization Exacerbated Rainfall Over European Suburbs Under a Warming Climate, Geophys. Res. Lett., 48, e2021GL095987, https://doi.org/10.1029/2021GL095987, 2021.
Yang, S. L., Zhang, J., and Xu, X. J.: Influence of the Three Gorges Dam on downstream delivery of sediment and its environmental implications, Yangtze River, Geophys. Res. Lett., 34, L10401, https://doi.org/10.1029/2007GL029472, 2007.
Yu, D., Shi, P., Liu, Y., and Xun, B.: Detecting land use-water quality relationships from the viewpoint of ecological restoration in an urban area, Ecol. Eng., 53, 205–216, https://doi.org/10.1016/j.ecoleng.2012.12.045, 2013.
Zhang, W., Wan, Q., Zhu, G., and Xu, Y.: Distribution of soil organic carbon and carbon sequestration potential of different geomorphic units in Shiyang river basin, China, Environ. Geochem. Hlth., 45, 4071–4086, https://doi.org/10.1007/s10653-022-01472-w, 2023.
Zhu, G.: Stable water isotope monitoring network of different water bodies in Shiyang River Basin, a typical arid river in China, Mendeley Data [data set], https://doi.org/10.17632/vhm44t74sy.1, 2022.
Zhu, G., Guo, H., Qin, D., Pan, H., Zhang, Y., Jia, W., and Ma, X.: Contribution of recycled moisture to precipitation in the monsoon marginal zone: Estimate based on stable isotope data, J. Hydrol., 569, 423–435, https://doi.org/10.1016/j.jhydrol.2018.12.014, 2019.
Zhu, G., Sang, L., Zhang, Z., Sun, Z., Ma, H., Liu, Y., Zhao, K., Wang, L., and Guo, H.: Impact of landscape dams on river water cycle in urban and peri-urban areas in the Shiyang River Basin: Evidence obtained from hydrogen and oxygen isotopes, J. Hydrol., 602, 126779, https://doi.org/10.1016/j.jhydrol.2021.126779, 2021.