the Creative Commons Attribution 4.0 License.
the Creative Commons Attribution 4.0 License.
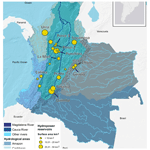
Patterns and drivers of water quality changes associated with dams in the Tropical Andes
Silvia López-Casas
Daniel Valencia-Rodríguez
Camilo Bernal-Forero
Juliana Delgado
Bernhard Wehrli
Luz Jiménez-Segura
The Tropical Andes is a biodiversity hotspot facing pressure from planned and ongoing hydropower development. However, the effects of dams on the region's river ecosystems, as mediated by physicochemical changes in the water quality, are poorly known. Colombia is unique among its peers in South America with respect to managing central public environmental databases, including surface water quality data sets associated with the environmental monitoring of dams. To assess the relationship between hydropower and Colombian river conditions, we analyze monitoring data associated with 15 dams, focusing on oxygen availability, thermal regimes and sediment losses because these properties are influenced directly by river damming and impose fundamental constraints on the structure of downstream aquatic ecosystems. We find that most Colombian dams (7 of 10) seasonally reduce concentrations of total suspended solids by large percentages (50 %–99 %) through sediment trapping. Most dams (8 of 15) also, via the discharge of warm reservoir surface waters, seasonally increase river temperatures by 2 to 4 ∘C with respect to upstream conditions. A subset of four dams generate downstream hypoxia (< 4 mg L−1) and water that is 2 to 5 ∘C colder than inflows, with both processes driven by the turbination and discharge of cold and anoxic hypolimnetic waters during periods of reservoir stratification. Reliance on monitoring data likely leads us to under-detect impacts: many rivers are only sampled once or twice per year, which cannot capture temporal shifts across seasons and days (i.e., in response to hydropeaking). Despite these blind spots, the monitoring data point to some opportunities for planners and hydropower companies to mitigate downstream ecological impacts. These findings highlight the importance of implementing environmental monitoring schemes associated with hydrologic infrastructure in developing countries.
Please read the corrigendum first before continuing.
-
Notice on corrigendum
The requested paper has a corresponding corrigendum published. Please read the corrigendum first before downloading the article.
-
Article
(3327 KB)
- Corrigendum
- Spanish version
-
Supplement
(486 KB)
-
The requested paper has a corresponding corrigendum published. Please read the corrigendum first before downloading the article.
- Article
(3327 KB) - Full-text XML
- Corrigendum
- Spanish version
-
Supplement
(486 KB) - BibTeX
- EndNote
Freshwater ecosystems and the services they provide to society are threatened worldwide, especially biodiversity (He et al., 2019) and fisheries resources (Deines et al., 2013; Stone, 2016). One of the major threats is the construction of dams for hydropower generation, which is booming, especially in tropical regions (Zarfl et al., 2014) where biodiversity is high (Ailly et al., 2014), as is fish protein dependence for poor rural populations (Kirby et al., 2010). Hydropower development is routinely promoted for its potential to meet the United Nations Sustainable Development Goals (SDGs), but the negative side effects of dam construction may create obstacles for achieving SDGs.
The benefits of hydropower are well understood – namely the provision of a renewable source of energy with a potentially low carbon emission intensity (Almeida et al., 2019), thereby contributing toward SDG 7 (affordable and clean energy) and SDG 13 (climate action). Critics, however, argue that the external costs of hydropower are frequently overlooked (Opperman et al., 2015). Dams cause the fragmentation of rivers (Anderson et al., 2018), disrupt hydrologic regimes critical for life histories of potamodromous freshwater fish (de Fex-wolf et al., 2019; Carvajal-Quintero et al., 2017) and alter the downstream physicochemical condition of river water (Winton et al., 2019). These threats to freshwater ecosystems misalign with SDG 14 (life below water), SDG 2 (zero hunger) and SDG 1 (no poverty).
Although retrospective case studies have been able to identify major ecological changes associated with dam construction, catastrophic ecological consequences of damming are not inevitable (Winemiller et al., 2016). Careful siting, design and operation can potentially mitigate the most costly of externalities associated with new reservoirs for hydropower (Moran et al., 2018; Opperman et al., 2017). With thousands of new projects under construction or in a planning phase globally (Opperman et al., 2015, 2017; Zarfl et al., 2014), the amount of work needed to analyze each case easily outpaces the available expertise, especially as hydropower planning and impact assessment spans financial, economic, social, engineering, hydrologic and ecologic dimensions. Synthetic assessments of existing hydropower portfolios have the potential to identify guidelines that could provide a shortcut for planners, helping them prioritize projects less likely to cause ecological harm and shelve more problematic proposals. Such assessments have been conducted at global scales with a focus on specific processes, such as sediment transport (Maavara et al., 2015; Vörösmarty et al., 2003), fragmentation (Grill et al., 2015), greenhouse gas emissions (Harrison et al., 2021), water supply (Opperman et al., 2017) and water quality (Winton et al., 2019).
Global-scale analyses, however, may have limited applicability to local-scale contextual realities and be less useful for decision-makers and on-site managers. Regional studies may play an important role with respect to providing synthetic understanding from a portfolio of existing hydropower projects while remaining relevant for a specific geography (Flecker et al., 2022; Kummu et al., 2010). In this study, we aim to provide a gap-bridging regional assessment focused on one of the many regional concentrations of hydropower expansion, the Tropical Andes of South America, a hotspot for freshwater biodiversity with 967 known endemic species and 17.5 % of fish species at risk of extinction (Tognelli et al., 2016). The region is rich in untapped hydropower resources thanks to steep elevational gradients and humid climates. Among the countries representing the region (including Peru, Ecuador and Bolivia), Colombia stands out as an ideal case for study because it maintains a centralized and publicly available data repository for all hydropower monitoring data and hosts roughly 40 % of the region's hydropower dams (Fig. 1; Table 1; International Commission on Large Dams, 2018).
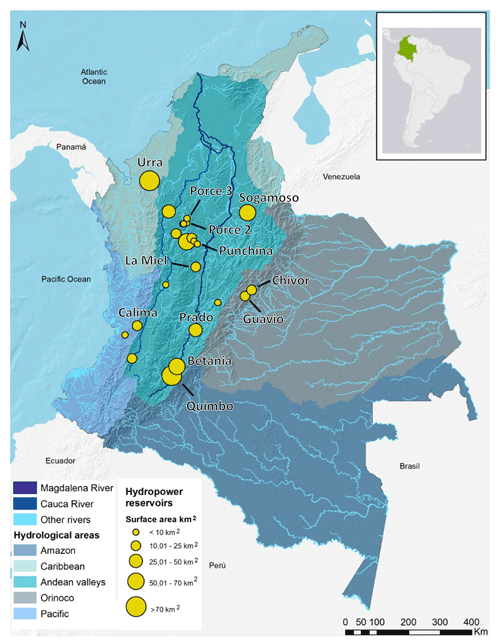
Figure 1A map of 22 reservoir-forming hydropower stations in Colombia regulated by the Autoridad Nacional de Licencias Ambientales (ANLA). The labeled reservoirs are those analyzed in this study. The topographic base map is publicly available from the Sistema de Información Ambiental de Colombia, and the locations of the hydropower projects are provided by Sistema de Información Geográfica, maintained by Autoridad Nacional de Licencias Ambientales.
Table 1Summary of the data available (in samples per year) for the assessment of reservoir stratification (no. of profiles), changes in river temperature, dissolved oxygen (DO) and total suspended solids (TSS) caused by dams for all hydropower stations licensed by the Autoridad Nacional de Licencias Ambientales (ANLA) in Colombia. Power plants with data for upstream vs. downstream comparisons are listed first and sorted by surface area.
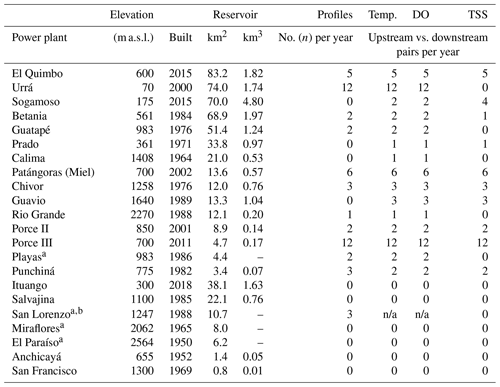
a Reservoir volume not known. b Turbinated discharge goes directly into Playas Reservoir, so there is no downstream river for the comparison of inflow conditions. n/a stands for not applicable.
We limit our focus to alterations of physicochemical parameters: temperature, dissolved oxygen and total suspended solids. These parameters are included in most aquatic environmental monitoring programs and define the critical oxic living conditions for aquatic fauna and the dynamic reconstruction of riparian habitat. Thermal shocks or regime changes are known to disrupt the life cycles of tropical biota (Caissie, 2006; Ward and Stanford, 1982), with cold-water discharges from dams frequently cited as negatively impacting fish (King et al., 1998; Todd et al., 2005; Preece and Jones, 2002; van Vliet et al., 2013; Cooper et al., 2019). Changes to the availability of dissolved oxygen impose physiological constraints on the potential metabolic activity of aquatic animals (Ekau et al., 2010), with all but the most adapted of macroscopic fauna unable to tolerate hypoxia (Agostinho et al., 2021; Chapman et al., 2002; Kramer and McClure, 1982). Disruptions to sediment transport rob floodplains and deltas of a critical lifeline (Giosan et al., 2014; Constantine et al., 2014) and can even restructure fish communities (Granzotti et al., 2018).
Our goal is to systemically assess Colombian dams with respect to their impacts on temperature, dissolved oxygen and total suspended sediments via the analysis of available monitoring data. As it is the mixing behavior of reservoirs that largely governs thermal and oxygen dynamics surrounding dams, we assess the Colombian dam portfolio for evidence of thermal stratification. Regarding the aforementioned parameters, we compare downstream monitoring stations to those upstream, which serve as a reference condition for the state of the river. We ask the following research questions:
-
Do Colombian reservoirs stratify?
-
Does the stratification lead to alteration of river thermal regimes?
-
Does stratification lead to hypoxia in downstream waters?
-
How effectively do dams trap sediment and reduce the concentration of suspended matter?
The answers to these questions have important implications for planning the future of hydropower development in Colombia and throughout the Tropical Andes biodiversity hotspot. We assess which characteristics of dams appear to be associated with the most problematic outcomes and provide recommendations to decision-makers and regulators regarding how to minimize harm moving forward.
2.1 Colombian dams and monitoring data
Colombia's Autoridad Nacional de Licencias Ambientales (ANLA) maintains environmental monitoring databases for all of Colombia's hydropower projects generating > 100 MW (26 power stations). Hydropower installations are distributed throughout the country's three branches of the Andes, but they are concentrated in the Magdalena–Cauca Basin, which is home to roughly 70 % of the total national population of 50 million people. Colombian hydropower installations span a wide range of elevations – from 70 m in the foothills to more than 2200 m in the high Andes, with a correspondingly high range of mean ambient temperatures. Because they are distributed across the many slopes of the Colombian Andes including within relatively dry inter-Andean valleys, they experience highly divergent precipitation regimes, including monomodal and bimodal rainfall patterns. The western slope of the western Andes is one of the Earth's wettest regions, with some municipalities reporting annual rainfall of more than 10 000 mm yr−1; in contrast, the Sogamoso Reservoir near Bucaramanga receives roughly 1/10 this amount of annual rainfall. The climate settings of Colombian dams are enormously diverse. Of the hydropower projects under ANLA jurisdiction, 22 are associated with a dam and reservoir. The remaining four are micro-hydro systems that divert a portion of river discharge into turbine intakes but do not completely impound their associated rivers; thus, we exclude them from analysis.
As part of the environmental licensing process, the companies that operate the hydropower projects are required (by ANLA) to monitor the environmental impacts of their operations and submit annual reports and monitoring data sets. The companies (which may be public or private) contract environmental consulting firms to collect field samples and analyze them in their laboratories. The data are assimilated into a central georeferenced database maintained by ANLA, which screens the data for quality. In a legal sense, these data are public, and ANLA works to guarantee access; however, to date, plans to build an online portal to facilitate direct data acquisition have not yet been implemented. Currently, data are available to the public via direct request to ANLA. For this study, we requested and were granted access to systematized hydropower monitoring data from the years 2017 and 2018, which were the most recent years of fully quality-controlled data (Table 1). ANLA is working to incorporate historical data into its database; however, as our primary goal was to examine changes across the portfolio of hydropower systems, rather than to examine the evolution of dammed rivers over time, we did not request data from previous years. Data are stored in .gdb files, which require graphical information systems software (i.e., Esri ArcMap) to read them. We screened all data for statistical outliers and for chemical and physical plausibility and did not find the need to discard any data from our focal parameters.
Data collection frequency (summarized in Table 1) is highly heterogeneous across sites, with two dams (Urrá and Porce III) being measured monthly, whereas most dams provide just one to three data points per year. Sampling frequency is generally higher for more recently constructed reservoirs, reflecting an update to regulations in 1993. Older reservoirs (pre-2000) are normally monitored only once or twice per year. Each dam's monitoring approach is uniquely tailored to its geographic circumstances, but it generally includes surface water sampling from one or more stations upstream of the reservoir and downstream of the dam as well as samples from various parts of the reservoir itself, often including depth profiles. Adding to the data heterogeneity is a lack of standardization, leaving key fields missing in some entries. For example, Colombia's largest dam by volume, Sogamoso Reservoir, has been extensively profiled, but the depth and time information has been omitted, limiting interpretability.
2.2 Analytical approach
As stratification is a fundamental driver of thermal and oxygen dynamics within and downstream of hydropower reservoirs (Winton et al., 2019), we first qualitatively assessed depth profiles (where available) for stratification strength. Because the profile data availability is quite variable, including the number of depth points sampled and seasonal coverage, we focus on deepwater oxygen levels (below the thermocline) and the magnitude of the oxygen concentration difference between surface and deep waters to sort each reservoir into coarse categories of strongly stratifying, weakly stratifying or non-stratifying. We classified reservoirs as strongly stratifying if they showed oxygen concentrations of < 2 mg O2 L−1 at depth and a difference between the surface of at least 3 mg O2 L−1. We classified all other reservoirs as weakly stratifying, as all showed differences in temperature and oxygen between the surface and deep waters of at least 2 ∘C and 1 mg O2 L−1 (for a summary, see Table S1 in the Supplement). Secondarily we use the densiometric Froude number (Parker et al., 1975), which compares the inertial force of reservoir water, based on mean flow-through velocity, with the gravitational force tending to maintain densiometric stability (Orlob, 1983; Deas and Lowney, 2000). The Froude number can be approximated using the following simplified formula including reservoir length (L), depth (D), discharge (Q) and volume (V): F= 320()() (Parker et al., 1975). This formula has been applied as a metric for stratification behavior (Ledec and Quintero, 2003).
To understand dam impacts, we rely on contemporaneous paired measurements of upstream vs. downstream conditions, and we interpret the differences to be attributable to dam effects, which is a widely used approach (Fovet et al., 2020). Colombian regulations stipulate that industrial or commercial activities should not alter water temperatures by more than 5 ∘C, which is a temperature difference that has been associated with acute responses from tropical biota, such as fish mortality from warm water (Cooper et al., 2019) or disruption to fish reproductive cycles from cold water (King et al., 1998). A more conservative thermal change threshold of ±2 ∘C may be warranted given that the community composition of macroinvertebrates is highly sensitive to subtle changes in the thermal regime (Eady et al., 2013; Preece and Jones, 2002) and assessments of global climate change effects on fish delimit a 2 ∘C threshold for impacts (van Vliet et al., 2013). Furthermore, as we are limited (by data availability) to just a few random comparisons for most sites for Colombia, we are unlikely to be capturing the most extreme moments of thermal impact; thus, the precautionary principle would dictate a stricter approach. Therefore, we consider a change of +2 or −2 ∘C to be evidence of warm-water or cold-water pollution, respectively. For changes in dissolved oxygen, we found that a loss of > 2 mg L−1 imposed by dams always corresponded to a downstream concentration of < 5 mg L−1, which is the regulatory minimum concentration for cold freshwaters in Colombia. Oxygen availability imposes a fundamental constraint on many aquatic species (Coble, 1982; Spoor, 1990; Ekau et al., 2010); therefore, we assess impact along a change threshold of 2 mg L−1 to distinguish between “minor” and “severe” oxygen loss. For sediment trapping, although the literature is clear about the potential consequences of dam-induced sediment loss from rivers, choosing an appropriate threshold demarcating what constitutes a severe loss is highly subjective, and there are no regulatory guidelines for total suspended solids. We report the gradient of responses, noting how many dams trapped > 50 % and > 99 % of inbound suspended sediments.
We note that, in some cases, dams can be oriented in a cascade whereby the outflow from one reservoir rapidly (or immediately) enters the reservoir for the next power station. In such a configuration, the inflowing water does not necessarily represent a neutral reference condition, as it has likely already been altered by the previous dam. This may bias us to underestimate the potential of lower-chain dams to alter water quality; however, without access to data on pre-dam river conditions, there is no alternative metric for reference conditions beyond upstream waters. As only 1 year of recent data is available for most projects, we focus our analyses on just the most recent year (2017 or 2018) under the assumption that covering an annual climate cycle is more important for answering our research questions than studying variation between years.
3.1 Stratification
Our analyses of reservoir mixing behavior indicate that most, if not all, Colombian reservoirs stratify strongly. Of the 22 reservoirs evaluated, 12 had available depth profile information; of these 12 reservoirs, 8 exhibited anoxia (dissolved oxygen (DO) concentration < 1.5 mg O2 L−1) in deep waters, indicating that they stratify sufficiently to prevent consistent reoxygenation from the surface (Table S1). The remaining four reservoirs lack evidence of acutely hypoxic deep water; however, with just two or three depth profiles per year, it is possible that this limited sampling did not coincide with periods of stronger stratification, which can develop rapidly in tropical lakes (Lewis, 1996). From the few reservoirs that have been profiled several times per year, it appears that Colombian reservoirs are rarely, if ever, well mixed (Figs. S1, S2 and S3 in the Supplement). This result is supported by our calculations of the densiometric Froude number (Fr) (Parker et al., 1975; Orlob, 1983), which indicate that, of the 12 dams with discharge data available (necessary for calculating Fr), all reservoirs except for Guatapé fall into the strongly stratifying domain of Fr < 0.3, regardless of whether mean depth or maximum depth is used for the calculation (Fig. 2). In Colombia, large reservoirs tend to stratify strongly, and this limnological reality creates the potential for thermal and biogeochemical disruptions to downstream aquatic ecosystems.
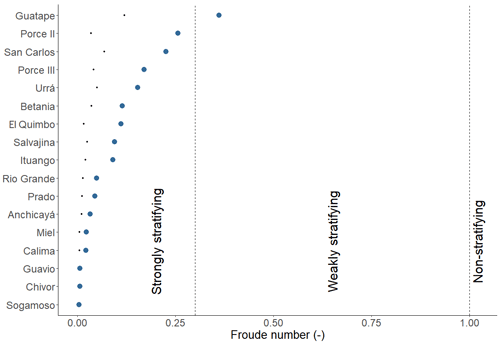
Figure 2Colombian reservoirs sorted by the densiometric Froude number (Fr), which is a function of reservoir depth, length, volume and discharge (Parker et al., 1975). The vertical lines at Fr = 0.3 and Fr = 1.0 indicate the expected boundaries between strongly stratifying, weakly stratifying and non-stratifying waterbodies (Orlob, 1983). Small dots represent Fr if the maximum depth (height of dam wall) is used instead of the mean depth, as recommended by Ledec and Quintero (2003). Underlying data were sourced from Autoridad Nacional de Licencias Ambientales and the International Commission on Large Dams (International Commission on Large Dams, 2018) (https://www.icold-cigb.org/, last access: 17 November 2020). Five reservoirs from Table 1 are excluded because of missing discharge data.
3.2 Thermal regime change
Consistent with the expectation that stratifying reservoirs will alter thermal regimes, we find that 9 of the 12 (75 %) Colombian reservoirs assessed generated temperature anomalies in the river of at least 2 ∘C (Fig. 3). Four reservoirs create cold-water pollution of at least −2 ∘C downstream, and seven reservoirs create warm-water pollution of at least +2 ∘C downstream. Two reservoirs – Urrá and El Quimbo – generate both cold- and warm-water pollution at different times of the year, illustrating the importance of temporal dynamics. Seasonal climate cycles that govern stratification, fluctuations in inflowing discharge associated with droughts/floods and dam operation (i.e., hydropeaking) may all influence the direction and magnitude of downstream thermal effects over timescales of months to minutes. Our ability to more broadly assess thermal changes is severely limited by the lack of frequent (seasonal or monthly) and detailed (with depth profiles) monitoring for most projects.
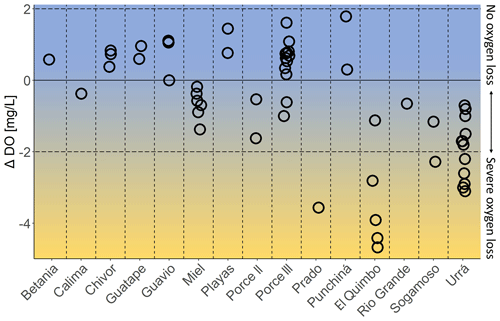
Figure 3The temperature differences between the upstream and downstream river surfaces of 15 hydropower dams in Colombia. Each point represents one pair of contemporaneous measurements from the most recent year of available data (either 2018 or 2017).
3.3 Hypoxia
We find that minor loss of oxygen (change in DO < 2 mg L−1) in waters downstream of Colombian dams was common (evident in 9 of 15 reservoirs), but severe oxygen loss relative to upstream (change in DO > 2 mg L−1) was associated with only 4 reservoirs (Fig. 4). These hydropower schemes (Urrá, El Quimbo, Sogamoso and Prado) are the same reservoirs that exhibited cold-water pollution (Fig. 3) – a coincidence that supports our assumption that both effects are driven by thermal stratification (Hutchinson and Loffler, 1956; Lewis, 1987). As with temperature, we observe high seasonal variation in upstream–downstream oxygen dynamics, which, again, supports the notion that hypoxic effects are sensitive to the seasonality of climate, stratification and dam operations.
3.4 Sediment trapping
Loss of total suspended sediments (TSS) associated with Colombian dams is pervasive; however, for several reservoirs, loss of TSS is observed to be extreme (Fig. 5). A total of 6 out of the 10 reservoirs that we assessed showed TSS decreases of more than 50 %, and two dams – El Quimbo and Sogamoso – logged losses of more than 99 %. Some reservoirs exhibited an increase in TSS downstream of the dam relative to upstream conditions, which may reflect local erosion processes or activities, while other reservoirs exhibited upstream sediment losses. For example, the Porce River has been dammed by several dams in a cascade system and, as a result, carries a relatively low sediment load; however, the river reach immediately below Porce III dam is turbid because of local illegal mining activity. Beyond such local artifacts, turbid inflow and clear outflow are a typical modality of Colombian dams, especially during rainy periods.
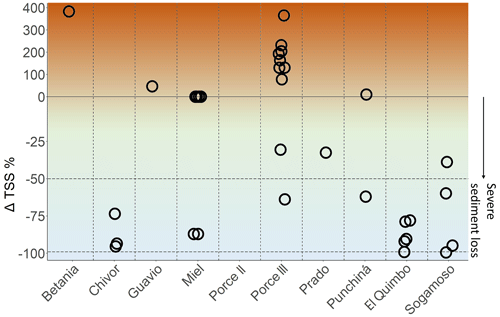
Figure 5Proportional change in mass of total suspended solids (TSS) in river water downstream of 10 Colombian hydropower dams relative to upstream values. Negative values indicate a loss of TSS, whereas positive values indicate an increase in TSS. Each point represents one pair of contemporaneous measurements from the most recent year of available data (either 2018 or 2017).
4.1 Drivers
In contrast to sediment loss and warm-water discharge, which appear to be ubiquitous, cold-water pollution and hypoxic effects appear to both afflict the same subset of four Colombian reservoirs (Urrá, El Quimbo, Prado and Sogamoso). Examining the characteristics of these reservoirs reveals some important commonalities: all are very deep (at least 70 m) and have long mean residence times, sufficient for anoxia to develop in the hypolimnion (as is evident at Urrá, which has depth profile data; Fig. S1). As all of the power plants have fixed depth intakes to run their turbines, there is no opportunity for operators to avoid discharging cold and hypoxic water when the thermocline/oxycline is shallower than the depth of intakes. In the case of El Quimbo, hydropower authorities inject liquid oxygen into discharge waters to meet the minimum dissolved oxygen requirement of 4 mg L−1, which is an expensive measure that they hope will be phased out by 2023 as oxygen demand in the reservoir lessens, as typically happens as reservoirs age. For Urrá, authorities have found a policy solution, passing a rule relaxing the minimum oxygen requirement to 2 mg L−1 for the first 5 km downstream of the dam, which is good for hydroelectric generators but does not reduce impacts on aquatic biodiversity and its associated ecosystems services. Both Prado and Sogamoso are out of compliance with the 4 mg L−1 threshold, and authorities are evaluating management options (unpublished documents from Autoridad Nacional de Licencias Ambientales).
The development of hypoxia in lakes and reservoirs can be exacerbated by long hydraulic residence times and elevated oxygen demand from organic matter inputs. Colombian dam data illustrate the importance of these reservoir and catchment characteristics for determining the risk of hypoxia developing in downstream rivers. Patángoras (Miel), in some important respects, appears to be like El Quimbo – they have similar depths, hydrologic residence times and fixed-depth intakes – but, in contrast to El Quimbo, Miel shows no sign of downstream hypoxia. Miel also relies on a reoxygenation system – an air bubbler in its oscillation cavern – but this is a much less intensive and less costly intervention than El Quimbo's need for liquid oxygen injection. Part of Miel's behavior may be attributable to the fact that its inflowing water is near saturation and has a very low biochemical oxygen demand (BOD; Table S2), meaning that severe hypoxia only appears rarely and typically well below 50 m depth (Fig. S2). This is likely a function of the well-preserved state of the Miel catchment, which includes robust riparian buffers that buffer the reservoir against extreme hydrologic fluctuations. In contrast, Porce II, which lies downstream of the Medellín metropolis (population of 4 million people), receives inflows that are already hypoxic (< 5 mg L−1) and have an elevated BOD (Table S2). Therefore, anoxia downstream of Porce II is not surprising. Further downstream, the Porce III Reservoir receives river water with substantial oxygen deficits, but its short residence time of just over 8 d prevents the dam from discharging water with significantly less oxygen than its inflows. Although the evidence from Colombian dams is anecdotal, it is consistent with the conventional logic that reservoirs loaded with high levels of organic matter from inflows (or left behind by inundated terrestrial ecosystems during reservoir filling) and with long residence times have greater potential to develop hypolimnetic anoxia for discharge downstream.
Dam design features, rather than environmental factors such as oxygen demand, offer an alternative explanation for why some dams avoid discharging hypoxic water downstream. A tower system with multiple intakes spanning 46 vertical meters in the Chivor Reservoir allows for discharged waters to be sourced from different depths as water levels fluctuate seasonally. Although we see no evidence of hypolimnetic hypoxia in this reservoir (Table S2), its selective withdrawal system could ensure oxic surface waters are passed downstream, as has been proposed as a solution for tailwater hypoxia in other tropical dams (Kunz et al., 2013). As previously stated, an air blower in the oscillation cavern at Miel dam provides reaeration of seasonally hypoxic (2 to 5 mg L−1) turbinated waters, such that discharged waters typically maintain DO concentrations of at least 6 mg L−1 (Table S2). A low BOD of inflows, as described above, may be an important mitigating factor, but evidence of the effectiveness of an engineered reoxygenation system for avoiding downstream hypoxia cannot be ignored.
4.2 Implications for river ecology
Many tropical freshwater aquatic species are highly susceptible to thermal regime changes (Olden and Naiman, 2010), and a temperature change of a few degrees (Fig. 3) may very well cause disruptions to the life cycles of sensitive species (King et al., 1998; Clarkson and Childs, 2000). In the Andes, surface-releasing reservoirs that discharge warm waters might hypothetically favor lowland species over the cooler-water species that we would normally expect to be present at a given altitude. Several potamodromous fish in Colombia's Magdalena River, such as bocachico (Prochilodus magdalenae) and pimelodids (Pseudoplatystoma magdaleniatum and Pimelodus yuma) migrate seasonally from lowlands to up to 1200 or 500 m elevation, respectively, to spawn, historically transiting river reaches that have been dammed in recent decades (López-casas et al., 2014, 2016). Therefore, these species may avoid spawning in rivers altered by upstream dams, effectively reducing the available reproductive habitat (López-Casas, 2015). Alternatively, if the fish do spawn, thermal changes may disrupt the timing of embryo development; this can be lethal, as has been documented in Colombian fish farms (Harvey and Hoar, 1980) and the Mekong River of tropical Southeast Asia (Li et al., 2021). Researchers have found that hydropower generation is associated with changes in the production of a hormone driving oocyte maturation in P. magdalenae, thus disrupting its spawning cycle in dammed rivers (de Fex-wolf et al., 2019). For Andean fish species, the ranges of thermal tolerance are poorly known; therefore, more research would be needed to test such a hypothesis.
Hypoxic conditions in the tailwaters of dams impose even more dramatic ecological constraints than temperature. Dissolved oxygen concentrations from below 3.5 to 5 mg L−1 trigger escape behavior in most macroscopic organisms (Spoor, 1990), and monitoring data reveal concentrations of less than 5 mg L−1 below seven Colombian hydropower dams. Because the data come from sparse grab samples, it is not clear how persistent hypoxia is in these river reaches, but, at minimum, they indicate a loss of viable habitat for hypoxia-sensitive species for at least some parts of the year. The fish communities downstream of Colombia's Porce III dam – one of the projects with hypoxic tailwater – have shifted, with a loss of some native species and replacement by invasive exotic species (Valencia-Rodríguez et al., 2022). The authors of this study attribute these shifts to habitat fragmentation, but anoxia/hypoxia could be an important factor, as many other studies have commented that the multiple changes and stressors imposed by dams are difficult to disentangle (van Puijenbroek et al., 2021; Young et al., 1976). A review from Brazil indicates that poor oxygen management associated with dams is likely to be a major driver of fish mortality in South American rivers, accounting for roughly 40 % of fish kills covered by the media (Agostinho et al., 2021). As with temperature, a limited understanding of species-specific fish tolerance thresholds makes it impossible to fully grasp the impact of hypoxia/anoxia on fish communities.
Sediment trapping in dams, which can reach 99 % efficiency in some Colombian cases, exerts ecological impacts at different spatial scales. Unnaturally clear river water below dams may favor a different pool of top predators (those adapted for visual hunting), which may displace species adapted to turbid conditions, setting off trophic cascades rippling down the food chain. This effect has been documented at a large dam in Brazil (Granzotti et al., 2018), but it has not yet been reported in the Tropical Andes. If the sediment load is not replenished through additional erosion in excess of deposition downstream of the dam, the floodplain and delta ecosystems, which depend on riverine sediment delivery, will ultimately be starved, disrupting the cycles of nutrient retention and transport (Kondolf et al., 2014). A modeling exercise estimates that up to 40 % of sediments in the heavily dammed Magdalena River basin are currently being trapped behind dams and that this figure could increase to up to 68 %, threatening the ecological functioning of the Colombia's Mompós Depression, one of South America's largest wetland complexes (Angarita et al., 2018). Scientists have sounded the alarm that, globally, sediment trapping at dams in concert with sea level rise may lead to a massive loss of coastal deltaic wetlands (Giosan et al., 2014; Dunn et al., 2019). Colombian river sediments support mangroves on the Pacific and Caribbean coasts, although the extent to which dams may be impacting floodplain lakes, coastal mangroves and deltaic processes within Colombia's coastal zones is unclear.
It is a challenge to pinpoint the exact mechanism by which dams alter their associated aquatic ecosystems, as they impose so many changes, spanning physical, hydrological, chemical and biological dimensions, simultaneously (Young et al., 1976). Many studies of dammed river ecology focus on hydromorphological changes in habitat structure or availability, loss of connectivity, and alterations to flow regimes (Bratrich et al., 2004; García et al., 2011). In this study, we focus on oxygen, temperature and suspended sediments and find that these are just as plausible mechanistic pathways for reductions in habitat quality and availability, thereby driving shifts in ecological communities in dam-adjacent ecosystems. Environmental assessments of dams should take care not to ignore the changes dams impose on the physicochemical condition of downstream waters nor to underestimate temperature, oxygen and suspended sediments as modes of ecological change.
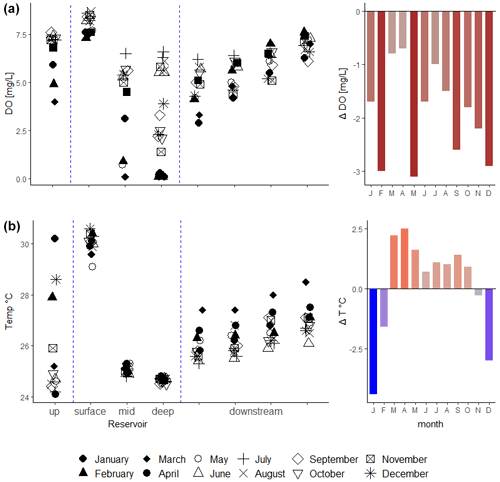
Figure 6Monthly monitoring data from the Urrá Reservoir in Cordoba, Colombia, in 2018 showing the upstream, reservoir and downstream (a) dissolved oxygen concentration and (b) temperature; the upstream–downstream difference is also shown for the two abovementioned parameters. Data were sourced from Autoridad Nacional de Licencias Ambientales, and data accessibility is described in Sect. 2.
4.3 Implications for management
4.3.1 Regulators
The frequency of problematic changes to river temperature, oxygen and sediment associated with dams suggests that regulators should consider risk assessments specific to these parameters and their associated ecological side effects in environmental impact assessments of new dam projects. It may even be worth assessing some older existing dams for which impacts have been underappreciated because of sparse monitoring requirements. Our analyses of monitoring data show that measurements frequent enough to capture seasonal variability are highly valuable. Just six Colombian reservoirs had three or more sampling dates per year, making it difficult to assess the effects of seasonality on physicochemical properties for most Colombian hydropower projects. For reservoirs with few measurements, we may be missing important seasons, and it is probable that hypoxia downstream of Colombian dams is more prevalent than what happens to be captured by these sparse observational “snapshots”. From sites with more frequent measurements, it is possible to glean a deeper understanding of the interactions between reservoir limnology and downstream conditions relative to the associated upstream reference. For example, the monthly monitoring scheme at Urrá Reservoir allowed us to observe that downstream oxygen concentrations were always depleted relative to the upstream values, even though the reservoir hypolimnion was only hypoxic for part of the year (Fig. 6a). Monthly monitoring at Urrá also gives a much more complete picture of thermal outcomes. It is evident that the Urrá Reservoir remains thermally stratified for 12 months of the year; moreover, while upstream river temperatures fluctuated some 6 ∘C seasonally, downstream temperatures were more homogenous and varied by less than 3 ∘C throughout the year (Fig. 6b). For 8 of the 12 months of the year, Urrá exerted only minor (< 2 ∘C) thermal effects on downstream waters. Thus, if we were to randomly select 3 months of data for Urrá, there would be a 42 % chance of not detecting one of the more extreme outcomes. Hence, for reservoirs with one (e.g., Betania) or a few measurements per year (e.g., Porce II), we are undoubtedly missing most of the range of their potential thermal impacts. It is likely that downstream thermal regime changes are even more prevalent than our analysis of available data seems to reveal. Because Urrá also employs many monitoring stations extending downstream of the dam, reoxygenation and warming can be tracked longitudinally as the river flows. This makes it possible to assess, for example, the length of the river reach for which dissolved oxygen is below a certain target threshold. It would be much easier to detect and understand impacts if more hydropower dams had such high-frequency and spatially rich monitoring programs.
Of course, increasing monitoring effort will increase costs; therefore, regulators will have to decide whether the utility of higher-frequency and higher-quality information justifies the expense. In addition, monitoring is not practical for detecting fluctuations in water conditions that occur over timescales of weeks, days or hours. Automated sensors placed strategically below reservoirs may be a cost-effective method for generating high-frequency measurements of dissolved oxygen, temperature, turbidity and other parameters. Although sensors cannot replace manual grab samples and laboratory analyses, which cover a much broader suite of parameters, they do provide a window into sub-daily fluctuations in water properties and may be effective for some classes of solutes (Pesántez et al., 2021). Hourly measurements can give insights into how hydropower operations (i.e., hydropeaking) interact with water conditions (Calamita et al., 2021), or they can capture the effects of episodic events such as a reservoir drawdown for maintenance. Remote monitoring via satellite is another potential low-cost solution. Remote sensing would not work for dissolved oxygen, but it may be viable for tracking changes in temperature and chlorophyll a and has been effectively applied for monitoring big shifts in river turbidity (Rudorff et al., 2018). We caution that satellite data alone, even in the most ideal of scenarios, still require ground-truthing; hence, they cannot completely replace ground-based monitoring.
We note that our analyses are only possible because Colombia has a centralized and public repository for environmental monitoring data – a system uncommon in the tropics and which puts it ahead of its peers. Neighboring countries along the Tropical Andes – Venezuela, Ecuador, Peru, Bolivia – all lack a national-scale public data repository for hydropower monitoring data, making systematic assessment of national portfolios difficult. Colombia's database, mandated and curated by ANLA, probably provides the best opportunity to understand river responses to hydropower for the Tropical Andes region.
4.3.2 Planners
For siting and designing future hydropower dams in the Tropical Andes, Colombia provides important lessons. First, loss of river sediments may be unavoidable, but the impact could be minimized by targeting catchments with low sediment loads or implementing sediment bypass systems where feasible. Second, downstream warm-water effects are probably unavoidable unless intake systems can mix surficial and deep waters to match upstream temperature. Finally, cold, anoxic discharges may be avoidable through siting/design choices. High-elevation reservoirs (e.g., Chivor and Guavio) and those in well-preserved catchments with low BOD (Miel) will be less prone to developing anoxia. Short residence times may prevent reservoirs from discharging hypoxic waters downstream (Porce III). Multiple intakes spanning a range of depths (Chivor) may help avoid downstream hypoxia. Failure to design hydropower schemes for effective oxygen management may later necessitate costly interventions, such as liquid oxygen injection (El Quimbo), or exemptions from environmental protection policies with considerable impacts (Urrá).
The energy sectors across the Tropical Andes would be wise to consider the ecological successes and failures of Colombia's existing hydropower portfolio as they decide which of the many hundreds of potential hydropower projects to prioritize. Our findings on the pervasiveness of challenges with respect to sediment loss, thermal regime change and hypoxia could be incorporated in existing basin-scale planning frameworks that already use multiple indices to avoid and minimize the anticipated environmental and social impacts of hydropower expansion (Opperman et al., 2015, 2017). The environmental impacts that we document in Colombia may also be useful for evaluating specific hydropower project plans. Due diligence during dam planning will not only help industry comply with environmental regulations but will also potentially optimize the net value that hydropower can deliver to society by avoiding and minimizing external environmental costs.
R. Scott Winton wrote R scripts to visualize the monitoring data .csv files extracted from the ANLA .gdb files by Daniel Valencia-Rodríguez. The R scripts and the .csv files are available upon request from the corresponding author (R. Scott Winton).
The data used in this work are public and may be requested directly from ANLA (which has an open data policy): https://datosabiertos-anla.hub.arcgis.com/ (ANLA, 2023). The .csv files analyzed in this paper may be requested directly from the corresponding author (R. Scott Winton).
The supplement related to this article is available online at: https://doi.org/10.5194/hess-27-1493-2023-supplement.
RSW, SLC, JD and LJS conceptualized the experiments. DVR and CBF curated the data. RSW analyzed the data. RSW, BW and LJS secured funding. All authors participated in the investigation. BW and LJS administered the project. RSW wrote R scripts to analyze and visualize the data. LJS and BW provided supervision. RSW wrote the original draft. All authors participated in the editing and review of the manuscript.
The contact author has declared that none of the authors has any competing interests.
Publisher's note: Copernicus Publications remains neutral with regard to jurisdictional claims in published maps and institutional affiliations.
This work was supported by a grant from the Leading House for the Latin American Region via the Centro Latino Americano-Suizo of the University of St. Gallen (CLS HSG), mandated by the Swiss State Secretariat for Education, Research and Innovation (SERI). The authors acknowledge additional in-kind support from the University of Antioquia and The Nature Conservancy. We thank the Autoridad Nacional de Licencias Ambientales for making their data publicly available and Silvia Vanegas Pinzón for facilitating access. The authors are also grateful to Juan Sebastian Hernandez Suarez for helpful suggestions that improved the manuscript.
This research has been supported by the Staatssekretariat für Bildung, Forschung und Innovation (grant no. SMG1924).
This paper was edited by Wouter Buytaert and reviewed by three anonymous referees.
Agostinho, A. A., Alves, D. C., Gomes, L. C., Dias, R. M., Petrere Jr., M., and Pelicice, F. M.: Fish die-off in river and reservoir: A review on anoxia and gas supersaturation, Neotrop. Ichthyol., 19, 1–28, 2021.
Ailly, D. A. B., Assemiro, F. E. A. S. C., Gostinho, C. A. S. A., and Arques, E. L. E. M.: The metabolic theory of ecology convincingly explains the latitudinal diversity gradient of Neotropical freshwater fish, Ecology, 95, 553–562, 2014.
Almeida, R. M., Shi, Q., Gomes-selman, J. M., Wu, X., Xue, Y., Angarita, H., Barros, N., Forsberg, B. R., García-villacorta, R., Hamilton, S. K., Melack, J. M., Montoya, M., Perez, G., Sethi, S. A., Gomes, C. P., and Flecker, A. S.: Reducing greenhouse gas emissions of Amazon hydropower with strategic dam planning, Nat. Commun, 10, 4281, https://doi.org/10.1038/s41467-019-12179-5, 2019.
Anderson, E. P., Jenkins, C. N., Heilpern, S., Maldonado-ocampo, J. A., Carvajal-vallejos, F. M., Encalada, A. C., and Rivadeneira, J. F.: Fragmentation of Andes-to-Amazon connectivity by hydropower dams, Science Advances, 4, 1–8, https://doi.org/10.1126/sciadv.aao1642, 2018.
Angarita, H., Wickel, A. J., Sieber, J., Chavarro, J., Maldonado-Ocampo, J. A., Herrera-R., G. A., Delgado, J., and Purkey, D.: Basin-scale impacts of hydropower development on the Mompós Depression wetlands, Colombia, Hydrol. Earth Syst. Sci., 22, 2839–2865, https://doi.org/10.5194/hess-22-2839-2018, 2018.
ANLA: Portal de Datos Abiertos ANLA, https://datosabiertos-anla.hub.arcgis.com/, last access: 4 April 2023.
Bratrich, C., Truffer, B., Jorde, K., Markard, J., Meier, W., Peter, A., Schneider, M., and Wehrli, B.: Green hydropower: a new assessment procedure for river management, River Res. Appl., 20, 865–882, https://doi.org/10.1002/rra.788, 2004.
Caissie, D.: The thermal regime of rivers: A review, Freshwater Biol., 51, 1389–1406, https://doi.org/10.1111/j.1365-2427.2006.01597.x, 2006.
Calamita, E., Siviglia, A., Gettel, G. M., Franca, J., Winton, R. S., Teodoru, C. R., Schmid, M., and Wehrli, B.: Unaccounted CO2 leaks downstream of a large tropical hydroelectric reservoir, P. Natl. Acad. Sci. USA, 118, e2026004118, https://doi.org/10.1073/pnas.2026004118, 2021.
Carvajal-Quintero, J. D., Januchowski-Hartley, S. R., Maldonado-Ocampo, J. A., Jézéquel, C., Delgado, J., and Tedesco, P. A.: Damming Fragments Species' Ranges and Heightens Extinction Risk, Conserv. Lett., 10, 708–716, https://doi.org/10.1111/conl.12336, 2017.
Chapman, L. J., Chapman, C. A., Nordlie, F. G., and Rosenberger, A. E.: Physiological refugia: Swamps, hypoxia tolerance and maintenance of fish diversity in the Lake Victoria region, Comp. Biochem. Phys. A, 133, 421–437, https://doi.org/10.1016/S1095-6433(02)00195-2, 2002.
Clarkson, R. W. and Childs, M. R.: Temperature Effects of Hypolimnial-Release Dams on Early Life Stages of Colorado River Basin Big-River Fishes, Copeia, 2000, 402–412, https://doi.org/10.1643/0045-8511(2000)000[0402:TEOHRD]2.0.CO;2, 2000.
Coble, D. W.: Fish Populations in Relation to Dissolved Oxygen in the Wisconsin River, T. Am. Fish. Soc., 111, 612–623, https://doi.org/10.1577/1548-8659(1982)111<612:FPIRTD>2.0.CO;2, 1982.
Constantine, J. A., Dunne, T., Ahmed, J., Legleiter, C., and Lazarus, E. D.: Sediment supply as a driver of river meandering and floodplain evolution in the Amazon Basin, Nat. Geosci., 7, 899–903, https://doi.org/10.1038/ngeo2282, 2014.
Cooper, C. J., Mueller, C. A., and Eme, J.: Temperature tolerance and oxygen consumption of two South American tetras, Paracheirodon innessi and Hyphessobrycon herbertaxelrodi, J. Therm. Biol., 86, 102434, https://doi.org/10.1016/j.jtherbio.2019.102434, 2019.
Deines, A. M., Bee, C. A., Katongo, C., Jensen, R., and Lodge, D. M.: The potential trade-off between artisanal fisheries production and hydroelectricity generation on the Kafue River, Zambia, Freshwater Biol., 58, 640–654, https://doi.org/10.1111/fwb.12055, 2013.
Dunn, F. E., Darby, S. E., Nicholls, R. J., Cohen, S., Zarfl, C., and Fekete, B. M.: Projections of declining fluvial sediment delivery to major deltas worldwide in response to climate change and anthropogenic stress, Environ. Res. Lett., 14, 084034, https://doi.org/10.1088/1748-9326/ab304e, 2019.
Eady, B. R., Rivers-Moore, N. A., and Hill, T. R.: Relationship between water temperature predictability and aquatic macroinvertebrate assemblages in two South African streams, Afr. J. Aquat. Sci., 38, 163–174, https://doi.org/10.2989/16085914.2012.763110, 2013.
Ekau, W., Auel, H., Pörtner, H.-O., and Gilbert, D.: Impacts of hypoxia on the structure and processes in pelagic communities (zooplankton, macro-invertebrates and fish), Biogeosciences, 7, 1669–1699, https://doi.org/10.5194/bg-7-1669-2010, 2010.
Deas, M. L. and Lowney, C. L.: Water Temperature Modelling Review, California Water Modelling Forum, Central Valley, California, https://www.waterboards.ca.gov/waterrights/water_issues/programs/bay_delta/california_waterfix/exhibits/docs/petitioners_exhibit/dwr/part2/DWR-1045 Bay Delta Modeling Forum 2000.pdf (last access: 2 April 2023), 2000.
de Fex-wolf, D., López-Casas, S., and Jiménez-Segura, L. F.: Hydropower effects on Prochilodus magdalenae (Prochilodontidae) reproduction: evidence from endocrine response, Rev. MVZ Cordoba, 24, 7180–7187, https://doi.org/10.21897/rmvz.1606, 2019.
Flecker, A. S., Shi, Q., Almeida, R. M., Angarita, H., Gomes-Selman, J. M., García-Villacorta, R., Sethi, S. A., Thomas, S. A., LeRoy Poff, N., Forsberg, B. R., Heilpern, S. A., Hamilton, S. K., Abad, J. D., Anderson, E. P., Barros, N., Bernal, I. C., Bernstein, R., Cañas, C. M., Dangles, O., Encalada, A. C., Fleischmann, A. S., Goulding, M., Higgins, J., Jézéquel, C., Larson, E. I., McIntyre, P. B., Melack, J. M., Montoya, M., Oberdorff, T., Paiva, R., Perez, G., Rappazzo, B. H., Steinschneider, S., Torres, S., Varese, M., Walter, M. T., Wu, X., Xue, Y., Zapata-Ríos, X. E., and Gomes, C. P.: Reducing adverse impacts of Amazon hydropower expansion, Science, 375, 753–760, https://doi.org/10.1126/science.abj4017, 2022.
Fovet, O., Ndom, M., Crave, A., and Pannard, A.: Influence of dams on river water-quality signatures at event and seasonal scales: The Sélune River (France) case study, River Res. Appl., 36, 1267–1278, https://doi.org/10.1002/rra.3618, 2020.
García, A., Jorde, K., Habit, E., Caamaño, D., and Parra, O.: Downstream environmental effects of dam operations: changes in habitat quality for native fish species, River Res. Appl., 27, 312–327, https://doi.org/10.1002/rra.1358, 2011.
Giosan, L., Syvitski, J., Constantinescu, S., and Day, J.: Climate change: Protect the world's deltas, Nature, 516, 31–33, https://doi.org/10.1038/516031a, 2014.
Granzotti, R. V., Miranda, L. E., Agostinho, A. A., and Gomes, L. C.: Downstream impacts of dams: shifts in benthic invertivorous fish assemblages, Aquat. Sci., 80, 1–14, https://doi.org/10.1007/s00027-018-0579-y, 2018.
Grill, G., Lehner, B., Lumsdon, A. E., MacDonald, G. K., Zarfl, C., and Liermann, C. R.: An index-based framework for assessing patterns and trends in river fragmentation and flow regulation by global dams at multiple scales, Environ. Res. Lett., 10, 015001, https://doi.org/10.1088/1748-9326/10/1/015001, 2015.
Harrison, J. A., Prairie, Y. T., Mercier-blais, S., and Soued, C.: Year – 2020 Global Distribution and Pathways of Reservoir Methane and Carbon Dioxide Emissions According to the Greenhouse Gas From Reservoirs (G-res) Model, Global Biogeochem. Cy., 35, e2020GB006888, https://doi.org/10.1029/2020GB006888, 2021.
Harvey, B. J. and Hoar, W. S.: Teoría y Práctica de la Reproducción Inducida en los Peces, Centro Internacional de Investigaciones para el Desarrollo, Ottawa, 50 pp., ISBN 0-88936-253-X, 1980.
He, F., Zarfl, C., Bremerich, V., David, J. N. W., Hogan, Z., Kalinkat, G., Tockner, K., and Jähnig, S. C.: The global decline of freshwater megafauna, Glob. Change Biol., 25, 3883–3892, https://doi.org/10.1111/gcb.14753, 2019.
Hutchinson, G. E. and Loffler, H.: The thermal stratification of lakes, P. Natl. Acad. Sci. USA, 42, 84–86, https://doi.org/10.1073/pnas.42.2.84, 1956.
International Commission on Large Dams: World Register of Dams, https://www.icold-cigb.org/GB/world_register/world_register_of_dams.asp, last access: 1 August 2018.
King, J., Cambray, J. A., and Impson, N. D.: Linked effects of dam-released floods and water temperature on spawning of the Clanwilliam yellowfish Barbus capensis, Hydrobiologia, 384, 245–265, https://doi.org/10.1023/A:1003481524320, 1998.
Kirby, M., Krittasudthacheewa, C., Mainuddin, M., Kemp-Benedict, E., Swartz, C., and de la Rosa, E.: The mekong: A diverse basin facing the tensions of development, Water Int., 35, 573–593, https://doi.org/10.1080/02508060.2010.514094, 2010.
Kondolf, G. M., Gao, Y., Annandale, G. W., Morris, G. L., Jiang, E., Zhang, J., Cao, Y., Carling, P., Fu, K., Guo, Q., Hotchkiss, R., Peteuil, C., Sumi, T., Wang, H.-W., Wang, Z., Wei, Z., Wu, B., Wu, C., and Yang, C. T.: Sustainable sediment management in reservoirs and regulated rivers: Experiences from five continents, Earth's Future, 2, 256–280, https://doi.org/10.1002/2013EF000184, 2014.
Kramer, D. L. and McClure, M.: Aquatic surface respiration, a widespread adaptation to hypoxia in tropical freshwater fishes, Environ. Biol. Fish., 7, 47–55, https://doi.org/10.1007/BF00011822, 1982.
Kummu, M., Lu, X. X., Wang, J. J., and Varis, O.: Basin-wide sediment trapping efficiency of emerging reservoirs along the Mekong, Geomorphology, 119, 181–197, https://doi.org/10.1016/j.geomorph.2010.03.018, 2010.
Kunz, M. J., Senn, D. B., Wehrli, B., Mwelwa, E. M., and Wüest, A.: Optimizing turbine withdrawal from a tropical reservoir for improved water quality in downstream wetlands, Water Resour. Res., 49, 5570–5584, https://doi.org/10.1002/wrcr.20358, 2013.
Ledec, G. and Quintero, J. D.: Good dams and bad dams: environmental criteria for site selection of hydroelectric projects, Latin America and the Caribbean Region: Sustainable Development Working Paper 16, vi + 21 pp., https://documents1.worldbank.org/curated/en/224701468332373651/pdf/303600NWP0Good000010Box18600PUBLIC0.pdf (ast access: 28 March 2023), 2003.
Lewis, W. M. J.: Tropical limnology, Annu. Rev. Ecol. Syst., 18, 159–184, 1987.
Lewis, W. M. J.: Perspectives in Tropical Limnology, edited by: Schiemer, F. and Boland, K. T., SPB Academic Publishing, Amsterdam, 43–64, ISBN 9051031130, 1996.
Li, T., Mo, K., Wang, J., Chen, Q., Zhang, J., and Zeng, C.: Science of the Total Environment Mismatch between critical and accumulated temperature following river damming impacts fi sh spawning, Sci. Total Environ., 756, 144052, https://doi.org/10.1016/j.scitotenv.2020.144052, 2021.
López-Casas, S.: Magdalena potamodromous migrations: effects of regulated and natural hydrological regimes, Universidad de Antioquia, 1–135, https://bibliotecadigital.udea.edu.co/dspace/bitstream/10495/5651/1/SilviaLopezCasas_2015_MagdalenaPotadromousMigrations.pdf (last access: 28 March 2023), 2015.
López-casas, S., Jiménez-segura, L. F., and Pérez-Gallego, C. M.: Peces migratorios al interior de una central hidroeléctrica: caso Miel I, cuenca del río Magdalena (Caldas-Antioquia), Colombia, Biota Colombiana, 15, 26–39, 2014.
López-Casas, S., Jiménez-Segura, L. F., Agostinho, A. A., and Pérez, C. M.: Potamodromous migrations in the Magdalena River basin: bimodal reproductive patterns in neotropical rivers, J. Fish Biol., 89, 157–171, https://doi.org/10.1111/jfb.12941, 2016.
Maavara, T., Parsons, C. T., Ridenour, C., Stojanovic, S., Dürr, H. H., Powley, H. R., and Van Cappellen, P.: Global phosphorus retention by river damming, P. Natl. Acad. Sci. USA, 112, 15603–15608, https://doi.org/10.1073/pnas.1511797112, 2015.
Moran, E. F., Claudia, M., Moore, N., Müller, N., and Hyndman, D. W.: Sustainable hydropower in the 21st century, P. Natl. Acad. Sci. USA, 115, 11981–11898, https://doi.org/10.1073/pnas.1809426115, 2018.
Olden, J. D. and Naiman, R. J.: Incorporating thermal regimes into environmental flows assessments: Modifying dam operations to restore freshwater ecosystem integrity, Freshwater Biol., 55, 86–107, https://doi.org/10.1111/j.1365-2427.2009.02179.x, 2010.
Opperman, J., Grill, G., and Hartmann, J.: The Power of Rivers: Finding balance between energy and conservation in hydropower development, Washington, D.C., 52 pp., https://www.nature.org/media/freshwater/power-of-rivers-report.pdf (last access: 28 March 2023), 2015.
Opperman, J., Hartmann, J., Raepple, J., Angarita, H., Beames, P., Chapin, E., Geressu, R., Grill, G., Harou, J., Hurford, A., D, K., Kelman, R., Martin, E., Martins, T., Peters, R., Rogéliz, C., and Shirley, R.: The Power of Rivers A Business Case, The Nature Conservancy, Washington, D.C., 1–88, https://www.nature.org/content/dam/tnc/nature/en/documents/powerofriversreport_final3.pdf (last access: 28 March 2023), 2017.
Orlob, G. T.: Mathematical Modeling of Water Quality: Streams, Lakes and Reservoirs, John Wiley & Sons, Chichester, UK, ISBN 978-0471100317, 1983.
Parker, F. L., Benedict, B. A., and Tsai, C.: Evaluation of mathematical models for temperature prediction in deep reservoirs, National Envrionmental research center, US Environmental Protection Agency, Corvallis, Oregon, 1975.
Pesántez, J., Birkel, C., Mosquera, G. M., Peña, P., Arízaga-Idrovo, V., Mora, E., McDowell, W. H., and Crespo, P.: High-frequency multi-solute calibration using an in situ UV–visible sensor, Hydrol. Process., 35, e14357, https://doi.org/10.1002/hyp.14357, 2021.
Preece, R. M. and Jones, H. A.: The effect of Keepit Dam on the temperature regime of the Namoi River, Australia, River Res. Appl., 18, 397–414, https://doi.org/10.1002/rra.686, 2002.
Rudorff, N., Rudorff, C. M., Kampel, M., and Ortiz, G.: Remote sensing monitoring of the impact of a major mining wastewater disaster on the turbidity of the Doce River plume off the eastern Brazilian coast, ISPRS Journal of Photogrammetry and Remote Sensing, 145, 349–361, https://doi.org/10.1016/j.isprsjprs.2018.02.013, 2018.
Spoor, W. A.: Distribution of fingerling brook trout, Salvelinus fontinalis (Mitchill), in dissolved oxygen concentration gradients, J. Fish Biol., 36, 363–373, 1990.
Stone, R.: Dam-building threatens Mekong fisheries, Science, 354, 1084–1085, https://doi.org/10.1126/science.354.6316.1084, 2016.
Todd, C. R., Ryan, T., Nicol, S. J., and Bearlin, A. R.: The impact of cold water releases on the critical period of post-spawning survival and its implications for Murray cod (Maccullochella peelii peelii): A case study of the Mitta Mitta River, southeastern Australia, River Res. Appl., 21, 1035–1052, https://doi.org/10.1002/rra.873, 2005.
Tognelli, M. F., Lasso, C. A., Bota-Sierra, C. A., Jiménez-Segura, L. F., and Cox, N. A.: Estado de conservación y distribución de la biodiversidad de agua dulce en los Andes tropicales, edited by: Tognelli, M. F., Lasso, C. A., Bota-Sierra, C. A., Jiménez-Segura, L. F., and Cox, N. A., IUCN, Gland, Switzerland, Cambridge, UK and Arlington, USA, 214 pp., ISBN 978-2-8317-1791-3, 2016.
Valencia-Rodríguez, D., Herrera-Pérez, J., Restrepo-Santamaría, D., Galeano, A., Winton, R. S., and Jiménez-Segura, L.: Fish community turnover in a dammed Andean River over time, Neotrop. Ichthyol., 20, 1–19, https://doi.org/10.1590/1982-0224-2021-0091, 2022.
van Puijenbroek, P. J. T. M., Buijse, A. D., Kraak, M. H. S., and Verdonschot, P. F. M.: Through the dam into troubled waters: Combined effects of stream fragmentation, habitat deterioration, and poor water quality on lowland stream fish distribution, River Res. Appl., 37, 1016–1024, https://doi.org/10.1002/rra.3829, 2021.
van Vliet, M. T. H., Ludwig, F., and Kabat, P.: Global streamflow and thermal habitats of freshwater fishes under climate change, Climatic Change, 121, 739–754, https://doi.org/10.1007/s10584-013-0976-0, 2013.
Vörösmarty, C. J., Meybeck, M., Fekete, B., Sharma, K., Green, P., and Syvitski, J. P. M.: Anthropogenic sediment retention: Major global impact from registered river impoundments, Global Planet. Change, 39, 169–190, https://doi.org/10.1016/S0921-8181(03)00023-7, 2003.
Ward, J. V and Stanford, J. A.: Evolutionary Ecology of Aquatic Insects, Annu. Rev. Entomol., 27, 97–117, 1982.
Winemiller, K. O., McIntyre, P. B., Castello, L., Fluet-Chouinard, E., Giarrizzo, T., Nam, S., Baird, I. G., Darwall, W., Lujan, N. K., Harrison, I., Stiassny, M. L. J., Silvano, R. A. M., Fitzgerald, D. B., Pelicice, F. M., Agostinho, A. A., Gomes, L. C., Albert, J. S., Baran, E., Petrere, M., Zarfl, C., Mulligan, M., Sullivan, J. P., Arantes, C. C., Sousa, L. M., Koning, A. A., Hoeinghaus, D. J., Sabaj, M., Lundberg, J. G., Armbruster, J., Thieme, M. L., Petry, P., Zuanon, J., Vilara, G. T., Snoeks, J., Ou, C., Rainboth, W., Pavanelli, C. S., Akama, A., van Soesbergen, A., and Saenz, L.: Balancing hydropower and biodiversity in the Amazon, Congo, and Mekong, Science, 351, 128–129, https://doi.org/10.1126/science.aac7082, 2016.
Winton, R. S., Calamita, E., and Wehrli, B.: Reviews and syntheses: Dams, water quality and tropical reservoir stratification, Biogeosciences, 16, 1657–1671, https://doi.org/10.5194/bg-16-1657-2019, 2019.
Young, W. C., Kent, D. H., and Whiteside, B. G.: The influence of a deep storage reservoir on the species diversity of benthic macroinvertebrate communities of the Guadalupe River, Texas J. Sci., 27, 213–224, 1976.
Zarfl, C., Lumsdon, A. E., Berlekamp, J., Tydecks, L., and Tockner, K.: A global boom in hydropower dam construction, Aquat. Sci., 77, 161–170, https://doi.org/10.1007/s00027-014-0377-0, 2014.
The requested paper has a corresponding corrigendum published. Please read the corrigendum first before downloading the article.
- Article
(3327 KB) - Full-text XML
- Corrigendum
- Spanish version
-
Supplement
(486 KB) - BibTeX
- EndNote