the Creative Commons Attribution 4.0 License.
the Creative Commons Attribution 4.0 License.
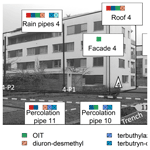
Sources and pathways of biocides and their transformation products in urban storm water infrastructure of a 2 ha urban district
Felicia Linke
Oliver Olsson
Frank Preusser
Klaus Kümmerer
Lena Schnarr
Marcus Bork
Jens Lange
Biocides used in film protection products leaching from facades are known to be a potential threat to the environment. This study identifies individual sources and entry pathways in a small-scale urban area. We investigate emissions of commonly used biocides (terbutryn, diuron, and octylisothiazolinone – OIT) and some of their transformation products (TPs; diuron-desmethyl, terbumeton, terbuthylazine-2-hydroxy, and terbutryn-desethyl) from a 2 ha residential area 13 years after construction has ended. Sampling utilizes existing urban water infrastructure representative for decentralized storm water management in central and northern Europe and applies a two-step approach to (a) determine the occurrence of biocides above water quality limits (i.e., predicted no-effect concentration, PNEC) and (b) identify source areas and characterize entry pathways into surface and groundwater. Monitoring focuses on the analysis of selected biocides and TPs by liquid chromatography–mass spectrometry/mass spectrometry (LC-MS/MS) in water samples taken from facades, rainwater pipes, drainage, and storm water infiltration systems. In standing water in a swale, we found high concentrations of diuron (174 ng L−1) and terbutryn (40 ng L−1) above PNEC for surface water. We confirmed expected sources, i.e., facades. Sampling of rain downpipes from flat roofs identified additional sources of all biocides and two TPs of terbutryn and one TP of diuron. Diuron and terbutryn were found in three drainage pipes representing different entry pathways of biocides. In one drainage pipe collecting road runoff, only diuron-desmethyl and terbutryn-desethyl were detected. In two other drainage pipes collecting infiltrated water through soil, terbuthylazine-2-hydroxy was additionally detected. One of the pipes collecting infiltrated water through soil concentration showed the highest concentrations of terbutryn and two of its TPs (terbutryn-desethyl and terbuthylazine-2-hydroxy). This suggests a high leaching potential of terbutryn. The applied two-step approach determined sources and pathways of biocide and their TPs. This study contributes to expanding knowledge on their entry and distribution and, thus, eventually towards reducing emissions.
- Article
(3487 KB) - Full-text XML
-
Supplement
(769 KB) - BibTeX
- EndNote
Biocides are bioactive substances and are regulated by Regulation (EU) no. 528/2012 (European Parliament and Council, 2012). They are, for example, used in renders and paints to inhibit the growth of microorganisms (e.g., algae and fungi) on facades (Sauer, 2017). The same active substances used as pesticides in agriculture can act as biocides in urban areas. In agriculture, the environmental fate of selected pesticides has been investigated since first usage of, e.g., diuron (Peck et al., 1980; Giacomazzi and Cochet, 2004) and terbutryn (Wu et al., 1974; Donati and Funari, 1993; Musgrave et al., 2011). Pesticides in agriculture have been intensively characterized regarding their sources (Reichenberger et al., 2007), environmental compartments for transformation (Fenner et al., 2013; Gassmann et al., 2013), and pathways to surface and underground waters (Doppler et al., 2012; Greiwe et al., 2021). However, this is not the case for biocides applied in an urban context. Diverse applications result in a variety of different sources and entry pathways (Burkhardt et al., 2011; Wieck et al., 2018; Paijens et al., 2021). Environmental impacts are relevant, since in catchments with mixed land use, overall loads of urban biocides were found to exceed those of agricultural pesticides (Wittmer et al., 2010).
Terbutryn, diuron, and octylisothiazolinone (OIT) represent commonly used biocides in coatings. Often, a combination of these three and more compounds is used against algae and fungi growth (Sauer, 2017). To date, all three biocides are transitionally authorized as film and construction materials' preservatives, i.e., product types 07 and 10, in the EU. Use of terbutryn and OIT is additionally legal for fiber, leather, rubber, and polymerized materials' preservatives (product type 09). In addition, OIT is open for use in preservatives for products during storage (product type 06), wood preservatives (product type 08), preservatives for liquid cooling and processing systems (product type 11), and working or cutting fluid preservatives (product type 13; ECHA, 2007–2020). Yet, diuron and terbutryn are regulated in the Water Framework Directive as prioritized substances (European Parliament and Council, 2013), and for final authorization as biocides, there is an ongoing risk assessment until 2024.
Transformation of biocides can principally occur directly on treated objects (e.g., by photolysis on facades; Bollmann et al., 2016, 2017b; Hensen et al., 2018) or along environmental pathways (e.g., in the soil; Bollmann et al., 2017a). Degradation time of terbutryn in soil ranges between 10 d (Lechón et al., 1997) and 231 d (Bollmann et al., 2017a), depending on, among others, temperature, pH, and organic and clay content. Terbutryn half-life in water under aerobic and anaerobic conditions were reported to be 193–644 and 266–400 d, respectively (Talja et al., 2008). Diuron is highly persistent in soil, sediment, and water. It is slowly degraded by hydrolysis and biodegradation with a half-life of a month up to a year (Giacomazzi and Cochet, 2004). Johann et al. (2018) showed an increase in sorption of diuron in the soil passage with an increase in organic matter. Bollmann et al. (2017a) estimated a half-life of diuron of more than 2500 d in soil. OIT has a reported half-life of 9.3 d (Bollmann et al., 2017b).
Diuron, terbutryn, and OIT used in facade coatings degrade to various transformation products (TPs; Hensen et al., 2020). Jirkovský et al. (1997) describe TPs of diuron formed by photolysis, and Giacomazzi and Cochet (2004) give an overview of all degradation pathways of diuron. Bollmann et al. (2016) investigate photodegradation products formed at facades of terbutryn and Bollmann et al. (2017b) of OIT. Here, we focus on four commonly investigated TPs of diuron and terbutryn originating at facades (diuron-desmethyl, terbuthylazine-2-hydroxy, terbutryn-desethyl, and terbumeton). Terbuthylazine-2-hydroxy and terbutryn-desethyl are formed by photolysis or biodegradation (Burkhardt et al., 2012; Bollmann et al., 2016, 2017a; Hensen et al., 2018). In a leaching study under natural weather conditions, Bollmann et al. (2016) found terbuthylazine-2-hydroxy, terbutryn-desethyl, and terbumeton in render and in leachate. Terbumeton is a photodegradation product that tends to remain on facades (Bollmann et al., 2017a). Terbutryn-desethyl, terbuthylazine-2-hydroxy, and terbumeton were classified as being probably toxic (Hensen et al., 2020). Diuron-desmethyl was identified as a photo TP (Burkhardt et al., 2012; Hensen et al., 2018) and is possibly also formed by microorganisms in soil (Hensen et al., 2018). Diuron-desmethyl was detected in urban runoff by various studies (Wittmer et al., 2010; Reemtsma et al., 2013; Hensen et al., 2018). In a field experiment, only 0.4 % of the diuron losses were made of diuron-desmethyl (Burkhardt et al., 2012). Moschet et al. (2014) confirmed diuron-desmethyl in rivers in Switzerland at concentrations ranging from 10 to 22 ng L−1. Diuron-desmethyl was classified as being most probably toxic or probably toxic (Hensen et al., 2020).
Biocides and their TPs are washed off from facades and enter the environment due to wind-driven rain on the facade. In urban areas, diuron, terbutryn, OIT, and their known TPs were detected in storm water (Burkhardt et al., 2011), surface water (Quednow and Püttmann, 2007), waste water treatment plants (Bollmann et al., 2014a), soil (Bollmann et al., 2017a), and groundwater (Hensen et al., 2018). This demonstrates the need to further understand sources, transformation, and pathways of these biocides applied on facades based on substance behavior. Various laboratory studies on the leaching of film preservatives of facades exist (Jungnickel et al., 2008; Schoknecht et al., 2009, 2013; Wangler et al., 2012; Bergek et al., 2014; Styszko et al., 2015; Urbanczyk et al., 2016), complemented by experimental studies under natural weather conditions (Burkhardt et al., 2012; Bollmann et al., 2016; Schoknecht et al., 2016; Bollmann et al., 2017a; Vega-Garcia et al., 2020). Release of biocides from facades is controlled by temperature, time between rain events, their extent, wind, UV exposure, biocide characteristics, and properties of paint and renders used (Paijens et al., 2019), as well as architectural design and geometry (Burkhardt et al., 2012).
Compared to experimental investigations, studies monitoring biocidal chemicals in urban storm water systems are relatively rare. They mostly focus on large heterogeneous areas where individual sources and entry pathways can hardly be identified (Wicke et al., 2015; Paijens et al., 2021). Studies have confirmed general biocide emissions from larger heterogeneous residential areas in storm water channels of separated sewer systems (Bollmann et al., 2014b; Wicke et al., 2015). In the scale of urban districts, monitoring has, so far, been concentrated on newly built areas to identify maximum biocide loads, shortly after construction was finished (Burkhardt et al., 2011; Bollmann et al., 2016). In another study, Hensen et al. (2018) investigated biocide emission from two small urban catchments with sizes of 2.95 ha and 8047 m2 but focused on the receiving parts of the water infrastructure (swale–trench system).
Much is still unknown about the emission potential and related environmental risks of biocides used in real-world urban areas. Existing studies often do not systematically follow the fate of biocides, including TPs, from source to sink. This is especially the case for urban districts with buildings aged 10 years or more. The aim of this study is, therefore, to identify sources and pathways of terbutryn, diuron, and OIT used for film protection in a 2 ha urban residential area, 13 years after initial painting, and to monitor some of their known TPs (diuron-desmethyl, terbuthylazine-2-hydroxy, terbutryn-desethyl, and terbumeton). These TPs have been already investigated in previous studies about biocide emissions from facades (e.g., Burkhardt et al., 2012; Bollmann et al., 2016, 2017a, Hensen et al., 2018), and analytical standards are available; thus, quantification is possible.
Using a stepwise approach and making use of existing urban water infrastructure, this study characterizes the environmental hazard of urban biocide use with only a small number of samples (n=60), thus limiting laboratory expenses. The main aim is to identify starting points and pathways where the release and transport of substances into adjacent aquatic systems occurs. Thereby, potential sources of biocides and TPs are identified by sampling at selected points of urban infrastructure, e.g., rain downpipes of flat roofs and by artificial experiments on facades and roof materials. After the identification of the main emission sources, pathways of biocides and TPs from the source to the catchment outlet are analyzed by sampling at selected drainage pipes or storm water canals. This also allows a comparison of the different pathways with regard to their degradation potential.
2.1 A two-step approach
We developed a two-step approach utilizing existing urban water infrastructure (Fig. 1). This was done to first check the relevance of biocide emission in the study area and then investigate sources and entry pathways. In a first step, we verified the occurrence of terbutryn, diuron, and OIT and their TPs (diuron-desmethyl, terbuthylazine-2-hydroxy, terbutryn-desethyl, and terbumeton) in urban aquatic systems, and therefore the potential of biocide leaching from building materials by sampling in a selected urban water infrastructure at the outlet of the study area. If the determined concentrations were higher than the available guidance values – e.g., measured environmental concentrations/predicted no-effect concentrations (MECs/PNECs) > 1 – then there is a risk, and thus, it is relevant to take further measures for risk mitigation. In this study, MECs/PNECs were chosen. If this criterion is fulfilled, step 2 is carried out to identify sources and pathways of selected biocides and their TPs. Finally, this knowledge may guide measures to mitigate biocide pollution.
2.2 Study area and sampling sites
The study area is located in the city of Freiburg in southwestern Germany (47∘59′ N, 7∘51′ E). It comprises a residential district of approximately 2 ha with buildings of uniform construction time, type, and structure. The modern four-story houses with thermal insulation composite systems were lastly painted in 2007, except for one small part of a roof that was repainted due to restoration in 2018. We obtained this information from a survey among residents and architects. The development plan was fixed in 2005 and construction started in 2006 (Stadt Freiburg i. Br., 2005). Roof areas are of diverse uses such as rooftop terraces and solar panels, both in combination with extensive green roofs. Houses have no roof overhang. Similar development areas exist in other parts of the city and are typical for modern architecture in central and northern Europe.
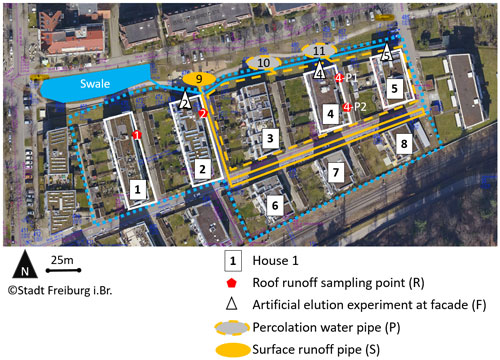
Figure 2Study area with sampling points and houses (aerial view). White lines indicate investigated houses. The blue dotted line encloses the drainage area connected to the swale. Yellow and dashed yellow lines enclose approximate areas connected to percolation water pipes and surface runoff pipe. Source: Stadt Freiburg i. Br.
The study area consists of eight houses connected to a separated sewer system that ends up in a swale that is dry during dry weather (Fig. 2). The focus of the sampling campaign was on four houses (1, 2, 4, and 5) and on surface runoff from a neighboring street that accepts water from three additional houses (6, 7, and 8). Footprints of buildings vary from 624 to 214 m2 (houses 1 to 8), with an approximate height of 13 m. Estimated facade areas covered by paints and renders are 634 to 296 m2 for houses 1 to 8 (see Table S1 in the Supplement). All houses were constructed at the same time and, thus, exposed to identical weather conditions over the years. At two houses (3 and 4), the used render contains diuron and OIT, according to inhabitants and invoices of construction work. For the other houses, used paint or renders could not be identified.
Houses 1, 2, and 4 all have flat roofs that are mostly covered by extensive greening. House 1 additionally has small rooftop terraces with an area of 44 m2. House 2 has an extensive green area with solar panels but no rooftop terrace. House 4 contains two larger rooftop terraces with approximate areas of 63 and 96 m2.
We placed our sampling sites along assumed biocide source areas and pathways (Fig. 3). Names of sampling points correspond to house numbers. Assumed sources were facades of buildings (F) and roof areas. Storm water from roof areas drains into rain downpipes. These rain downpipes were sampled at three individual houses (roof runoff sampling points; R). Water from rainwater downpipes flows via paved gutters into a small grass-covered trench along the houses. This trench leads into the swale system. Surface runoff of a neighboring street is diverted by an underground pipe and drains into the same trench. Water samples were taken at this pipe (surface runoff pipe; S).
A special feature of the study area is the possibility to collect water samples after soil passage. A drainage system above an underground parking garage located beneath houses 3 to 5 collects all water infiltrating from gardens and green areas surrounding the houses, including facade runoff. The soil consists of a 10–20 cm topsoil layer covered with grass. Below, the soil is composed of expanded clay aggregates to reduce the weight on the underground parking garage. At regular distances, there are pipe outlets of this drainage system directing water to the small trench at the northern site of the study area. The material of these pipes is polyvinyl chloride. Of these pipes, hereafter referred to as percolation water pipes (P), two were selected for sampling, i.e., P10 and P11. Both pipes represent the surrounding area of a house with all the infiltrating water.
2.3 Sampling
Water samples were collected in 1 L brown glass bottles previously washed with deionized water. All samples were immediately stored at 4 ∘C and sent to the laboratory within 24 h after sampling.
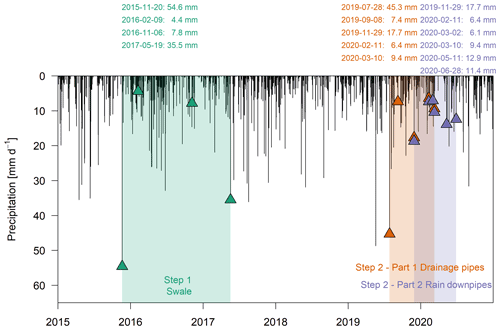
Figure 4Daily precipitation about 5 km away from study site between 2015 and 2020. Sampled events are marked according to the two-step approach. Colors correspond to the steps of the experimental procedure and sampling sites. Precipitation data are from the Deutscher Wetterdienst (Station Freiburg).
2.3.1 Event samples
From 2015 to 2017, we took samples from the swale system (step 1; Fig. 1) during four individual rain events (Table 1). Samples were taken as grab samples during the events and are not necessarily representative of the entire rain event. In 2019 to 2020, roof rainwater was sampled at rain downpipes from houses 1, 2, and 4 to account for different roof area usages (step 2; part 1). For houses 1 and 2, samples were taken at one pipe and for house 4 at two pipes. A second pipe at house 4 was additionally sampled, since the first pipe showed high biocide concentrations during initial sampling. Duplicate samples were taken at least during one event for each pipe. For step 2 (part 2), water samples were collected at two percolation water pipes (P10 and P11) and at the pipe collecting surface runoff from a neighboring street (S9). We sampled all three pipes during four events, including duplicate samples during the first two events.
Figure 4 shows daily precipitation at a climate station about 5 km away from the study area and the time of sampling in swale at drainage pipes and at rain downpipes. Daily rainfall ranged between 0 and 54.6 mm. The first swale sample was collected shortly after the highest rainfall in the 6-year monitoring period. We determined the representativeness of the sampled rain events by comparisons to a 30-year period of rainfall data.
2.3.2 Elution experiments
To determine potential biocide wash off and, thus, the significance of different sources of biocides, we conducted artificial elution experiments. We sprinkled facades with 1 L of deionized water. The water was poured within 30 s with a measuring cup across an area of approximately 0.25 m2 of the facade. We collected the water flowing down the facade using a rectangular container made out of stainless steel held against the facade. The container was cleaned with acetone prior to its use. The collected water was stored in brown glass bottles. Water samples were collected at the facades oriented northwest at houses 2, 4, and 5. Additionally, several elution experiments were conducted on the roof area of house 4. They included roof facades (n=6) and roof materials, namely wooden terraces (n=6), railings (n=2), roofing foils (n=2), roof access (n=1), roof cladding (n=1), elevator shaft foil (n=1), and grass foil (n=1). Most roof materials (i.e., roofing foils, roof access, roof cladding, elevator shaft foil, and grass foils) were tested where their orientation was vertical, for example, around vertically oriented pipes or shafts. Railings were accessible from all sides, allowing us to conduct elution experiments by setting a container underneath them. At the railings there were certain limitations regarding area poured with water so this might not be comparable to experiments at the facades. We dismantled parts of the wooden terraces to access the substructure and set a container underneath the wooden bars. In this way, leaching experiments on a horizontal surface were performed the same way as on the facades.
2.3.3 Leaching test
An additional self-fabricated leaching test was performed on the wooden terrace. The aim of this test was to determine if any leaching takes place. A wooden part of the terrace was removed and sawn into two pieces each, with a volume of 128 cm3. Each piece was put into 500 mL of deionized water and shaken for 24 h. Subsequently, the water was changed and shaken again for 24 h.
2.4 Chemical analysis
2.4.1 Chemicals and reagents
Analytical standards of diuron, diuron-desmethyl, terbutryn, terbutryn-d5, terbumeton, terbuthylazine-2-hydroxy, and terbutryn-desethyl were purchased from Neochema GmbH (Bodenheim, Germany). Diuron-d6 was received from HPC Standards GmbH (Borsdorf, Germany). OIT was purchased from LGC Standards (Teddington, Middlesex, UK). Acetonitrile (LC–MS grade; VWR International GmbH, Darmstadt, Germany) was used as organic mobile phase in chromatography and for the preparation of stock solutions.
2.4.2 Preparation and measurement of environmental samples
Environmental samples (0.9 L for surface water) were filtered with a folded filter (type 113 P cellulose ø membrane 240 mm; Carl Roth GmbH + Co. KG, Germany). The filtrate was spiked with the internal standard diuron-d6 and terbutryn-D5 (20 µL of 1 mg L−1, respectively). For solid phase extraction (SPE), cartridges (CHROMABOND® HR-X 6 mL; 200 mg−1) were conditioned with 10 mL methanol and washed with 10 mL pure water. Environmental samples were enriched on the cartridges via Teflon capillary and a vacuum extraction unit. After enrichment of the samples, cartridges were washed with 5 mL pure water and air-dried for 5–10 min. Elution was done with 10 mL of a mixture of methanol and chloroform (; 1:1). The eluted phase was dried with nitrogen and resolved in 200 µL acetonitrile. Analysis of environmental samples was performed with a Triple Quadrupole (Agilent Technologies, Inc.; 1200 Infinity LC system and 6430 Triple Quad; Waldbronn, Germany) with electrospray ionization (ESI) in positive mode. A C18 column (Nucleodur® EC 100-3 C18ec; 3 µm particle size, 125 mm length, and 2 mm diameter; Macherey–Nagel) was used as the stationary phase, and the temperature was set to 30 ∘C. Acetonitrile (A) and water with 0.1 % formic acid (B) were used as the mobile phase. Gradient was 0–1 min (75 % B), 1–7 min (40 % B), 7–12 min (15 % B), 12–15 (15 % B), 15–17 min (75 % B), and 17–20 min (75 % B). Flow was 0.4 mL min−1 and the injection volume was 5 µL.
Biocides and their TPs were identified and quantified on the basis of a precursor and two fragment ions (quantifier and qualifier). During each analysis, calibration standards covering 0.5–1000 µg L−1 were measured. For all substances except terbuthylazine-2-hydroxy, the last four calibration standards (100, 250, 500, and 1000 µg L−1) were only taken into account if enriched sample concentrations exceeded 50 µg L−1. Samples with biocides concentrations > 1000 µg L−1 (after enrichment) were diluted, and extraction and measurement were repeated. Recovery was determined by spiking water samples with 1 mg L−1 of analytical standard and was found to be 97.7 % (diuron), 88.5 % (terbutryn) and 93.5 % (OIT), 85.0 % (diuron-desmethyl), 66.2 % (terbumeton), 50 % (terbuthylazine-2-hydroxy), and 92 % (terbutryn-desethyl; Hensen et al., 2018). Terbuthylazine-2-hydroxy and terbumeton had lower recovery rates compared to the other compounds. Hence, the results of the two TPs should be treated with more caution, and values might be underestimated. If positive results are obtained despite the mediocre recovery rate, insights into the fate of both TPs can already be obtained. Concentration of terbutryn, its TPs, and OIT were calculated in reference to terbutryn-d5. Concentrations of diuron and its TP were calculated in reference to diuron-d6. Analysis was performed with the MassHunter Quantitative Analysis (QQQ) software (Agilent Technologies, Inc.). Pure water as a blank sample and a reference with 100 µg L−1 terbutryn, diuron, and OIT were carried along each analysis as quality control.
Limits of detection (LOD) and quantitation (LOQ) were calculated with DINTEST (version 2003) according to DIN 32645 (result uncertainty 33.3 %; probability of error 1 %) in a concentration range from 0.5–50 µg L−1. Due to deviations from linearity at low concentrations, different concentration ranges were used for diuron-desmethyl and terbuthylazine-2-hydroxy (5–100 and 25–1000 µg L−1). Each calibration curve was determined as the mean of three independent measurements. LOD and LOQ are given in Table 2. Table 3 gives an overview of analyzed substances.
2.5 Estimation of biocide emissions over 2 years
This section describes how total biocide emissions within a certain time period can efficiently be estimated based on a few chronological grab samples. In the study area, this was possible for a roof facade that was repainted 2 years ago according to the house owners. Since it was situated on top of a flat roof, all storm water, including biocide emissions, was collected by the rain downpipes (see Sects. 2.3.1 and 3.2.2). Since other sources were excluded by elution experiments, the net biocide emission BE (milligrams) from this single facade could be estimated multiplying average biocide concentrations C (milligrams per liter; mg L−1) in the downpipes by the volume of roof runoff. Roof runoff was estimated multiplying flat roof area A (400 m2) by recorded rainfall P from the date of painting until time of sampling (1462 mm over 2 years). To account for evaporation losses, runoff coefficients (RCs) from the literature, i.e., 70 % for the roof terrace and 30 % for extensive roof greening (DIN 1986-100; 2016), were employed as follows:
BE was related to the initial amount applied on the facade BI (milligrams) to quantify biocide leaching (BL) percentage (%) as follows:
BI was estimated multiplying the area of the newly painted facade (AF; 10 m2) by the literature values of typical amounts of paint (AP; 0.2 L m−2), including typical biocide concentrations CP (1500 mg L−1 of diuron and terbutryn; 500 mg L−1 of OIT; Sauer, 2017) as follows:
Note that BE is only a rough estimation, with various limitations discussed in Sect. 3.2.3. These include the applied literature values for initial amounts of biocides and paints and no consideration of dry and wet periods or wind-driven rain, material aging, and limited sampling. We compared the estimated BE with the literature values to determine whether estimations are feasible.
3.1 Standing water in swale (step 1)
Our data suggest that biocides emission are relevant in the investigated district, since measured concentrations of terbutryn and diuron exceeded PNEC values (Fig. 5). Terbutryn and diuron were detected during all four events when standing water in the swale was sampled. Maximum concentrations in the first event (0.04 µg L−1 terbutryn; 0.17 µg L−1 diuron) exceeded PNEC values of surface water (0.034 µg L−1 for terbutryn and 0.02 µg L−1 for diuron). OIT was not detected in the swale. These concentrations were within the range found in other studies of urban storm water. Reported concentrations of terbutryn ranged between <10 and 360 ng L−1 in storm water channels of a separated sewer system in the city of Berlin, Germany (Wicke et al., 2015). In the same study, diuron showed maximum concentrations of up to 0.6 µg L−1 and OIT up to 60 ng L−1. Gasperi et al. (2013) analyzed storm water at the outlet of three catchments in Paris, Nantes, and near Lyon. Mean concentrations were 2 µg L−1 for diuron, and OIT concentrations remained below 4 ng L−1. In another district of Freiburg, 2.8 km southwest, Hensen et al. (2018) found up to 5 ng L−1 diuron, 160 ng L−1 terbutryn, and up to 67 ng L−1 OIT in a swale–trench system.
The TPs terbuthylazine-2-hydroxy and terbutryn-desethyl were detected in samples of the first two and the fourth event, diuron-desmethyl in samples of the second and third event. Terbumeton was detected only during the third event at very low concentrations of 0.25 ng L−1. Diuron-desmethyl concentrations ranged between 0.2 and 4 ng L−1, terbuthylazine-2-hydroxy between 8 and 48 ng L−1, and terbutryn-desethyl between 43 and 335 ng L−1. Hensen et al. (2018) found concentrations of up to 23 ng L−1 for terbuthylazine-2-hydroxy, 73 ng L−1 for terbutryn-desethyl and 2 ng L−1 for diuron-desmethyl in a swale–trench system located 2.8 km southwest. In total, two of the four detected TPs (diuron-desmethyl and terbuthylazine-2-hydroxy) are described as being most probably toxic or probably toxic (Hensen et al., 2020). Differences in types and concentrations of detected substances between locations and events might be due to different sources (e.g., newly painted facades), different intensities of UV radiation, and different precipitation amounts and intensities that affect biocide emissions (Paijens et al., 2019). This may explain the highest biocide concentrations during the first rain event, which was the largest of the monitoring period (Fig. 4). It corresponded to the fifth-largest daily rainfall in the 1990–2020 period. All our sampled events were larger than 4 mm d−1, although this only applied to 39 % of the events in the 1990–2020 period. Of the four sampled events in the swale, two exceeded 30 mm d−1, which was the case for only 1 % of the 1990–2020 events. These findings suggest a bias towards larger events in our sampling. However, this analysis is limited since the weather station providing long term rainfall data is located 5 km away from the investigated site, and there might be differences in local precipitation. Due to dependencies on rain event magnitudes and, thus, high variability in detected concentrations in the swale, we did not expect new findings from a renewed sampling campaign in 2019–2020 and, thus, focused on sources of biocides in the next step.
Swale water infiltrates to groundwater, and contained pollutants can, thus, be an issue for groundwater quality (Burri et al., 2019). Hensen et al. (2018) found diuron, terbutryn, OIT, diuron-desmethyl, terbuthylazine-2-hydroxy, and terbutryn-desethyl in the shallow groundwater 2.8 km southwest. In our study, the groundwater table is significantly lower, i.e., about 5 to 7 m below the surface, which might reduce the risk of contamination. Indeed,sporadic groundwater sampling in the vicinity of our swale did not show any detectable contamination. Still, biocides and their TPs remain a risk, and there is a need for more intense monitoring of these substances in groundwater (Foster and Cogu, 2019).
3.2 Source allocation (step 2 – part 1)
3.2.1 Facades
Elution experiments at the northwestern facades of houses 2, 4, and 5 showed a different biocide composition of the wash off (Fig. 6). We conducted the elution experiment twice at different parts of the facades and found similar concentrations in the obtained duplicates. Due to our simple experimental approach and missing information about initial biocide loads, we will focus on a qualitative evaluation of the results. Terbutryn was detected at one facade (F5), OIT at two (F2 and F4), and diuron not at all. Instead, diuron-desmethyl was detected at one facade (F2), which suggests in situ photo transformation of the parent compound (Burkhardt et al., 2012). Terbuthylazine-2-hydroxy was found at two (F2 and F4) facades, terbutryn-desethyl at one (F5), and terbumeton at none. Both detected TPs of terbutryn can be formed by photo transformation (Hensen et al., 2018). Detected TPs and biocides imply the application of terbutryn at all facades, diuron only at facade F2, and OIT at facades F2 and F4. Substances below LOD might have been part of used renders or paints and may have been washed out or were not detected due to low concentrations. Biocides might still be present in deeper layers of the facade while being degraded on the surface (Uhlig et al., 2019). Although all houses were built at the same time, buildings show different color shades and sizes of protruding parts. Differences in detected TPs at the individual facades might be due to different color shades, although its influence is reported to be rather low (Bollmann et al., 2018). Urbanczyk et al. (2019) found differences in pigments contained in paints and renders influencing formation of TPs. Other factors influencing the differences in found TPs can include local wind conditions, UV exposure (Paijens et al., 2019), and, most probably, differences in the application of paint and renders. Terbutryn was only found at F5, which pointed to a different product used during construction and which is also evident by different color and by very high concentrations of the TP terbuthylazine-2-hydroxy.
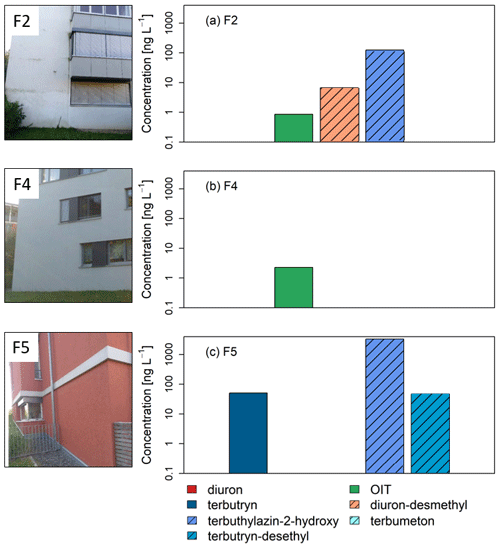
Figure 6Photos of sampled facade sides and results of facade elution experiments at houses 2, 4, and 5.
All houses were constructed 13 years before the elution experiments. Studies under natural weather conditions found that most leaching takes place within the first months after painting. Thereafter, concentrations are reported to be lower and no longer as variable as before (Burkhardt et al., 2012; Bollmann et al., 2016). Although there is no experimental study investigating facade leachate over a time period of more than a decade, it can be stated that leaching decreases significantly. Hensen et al. (2018) showed during a sprinkling experiment that leaching of biocides may occur even 15 years after initial painting. Thus, biocide leaching after 13 years should not be considered as surprise. Remarkably, diuron was found in swale samples but not in the facade elution experiments. Nevertheless, its use in paints and renders of the facades could indirectly be confirmed by the detection of its TP diuron-desmethyl. Additionally, diuron might have been used at other facades in the area which were not sampled here. Emitted OIT was very low at all facades and, thus, disappeared on its way to the swale. The elution experiments generally suggest that facades are a primary source of biocides, and their TPs in this urban catchment confirm the outcomes of the facade sprinkling experiments of Hensen et al. (2018).
3.2.2 Roofs and roof materials as source of biocides in storm water
In all rain downpipes, biocides were detected, indicating the application of biocides on the flat roofs. However, concentrations of biocides and TPs largely differed between pipes and houses (Fig. 7). Concentrations in pipe R4–P1 were high, as we did not expect roof areas as a biocide source. We sampled an additional pipe at the same building (R4–P2) to exclude potential contamination in the first pipe. Both R4 pipes showed concentrations of a similar magnitude. For comparison, we decided to sample one pipe at another house (R2). To confirm the low concentrations, we sampled at a third building (R1). We then sampled multiple events at all pipes.
Concentration differences between houses can be attributed to different products used on different roofs, as previously discussed for the investigated facades (Sect. 3.2.). Additionally, houses differ in the layout of their roofs (Sect. 2.2), which might also lead to differences in biocide use and emission. Due to the limited number of investigated houses, general conclusions, if certain roof structures pose a higher risk for biocide emission than others, cannot be drawn. Generally, biocides are used in roof sealants, treated tiles, roof paints, and bitumen roofing membranes (Paijens et al., 2019). Terbutryn and OIT may be included in paints for roof tiles (Jungnickel et al., 2008). To the best of our knowledge, we are not aware of studies that found biocides emitted from solar panels that are installed on the roof of house 2, where we detected low Terbutryn concentrations. Wicke et al. (2015) found different terbutryn concentrations in storm water channels draining areas differentiated by their construction type and related these differences to the use of roof paints. In most studies, roof runoff is characterized by samples in storm water channels of separated sewer systems (Burkhardt et al., 2011; Wicke et al., 2015). If roof areas were investigated as a source for biocide emission, mostly experiments with bituminous roof sheets were carried out (Bucheli et al., 1998; Burkhardt et al., 2007; Wicke et al., 2015). We are not aware of a study that detected terbutryn, diuron, or OIT in rainwater downpipes. In our study, diuron and diuron-desmethyl were found in high concentrations (1 µg L−1 and 320 ng L−1, respectively) in the two rain downpipes of house 4. These findings indicated the presence of an important source that had to be identified by leaching and elution experiments (Fig. 8).
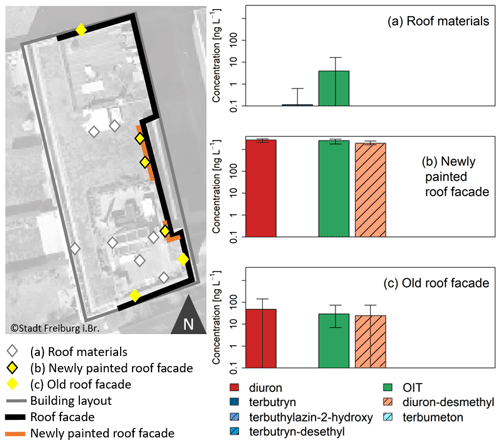
Figure 8Schematic view of roof area of house 4 with sampling spots. Results of sampling for (a) roof materials include roof balustrade, railings, roofing foil, roof access, roof cladding, elevator shaft foil and grass foil, (b) newly painted roof facade, and (c) the old roof facade. Bars show the mean; lines show minimum and maximum of the sample category.
Leaching tests of the wooden roof terrace taken from house 4 showed no biocides or TPs present in the extraction solution. Elution experiments of the wooden roof terrace showed no concentrations and, thus, confirmed findings of the self-fabricated leaching test. Elution experiments of various roof materials showed very low concentrations of terbutryn (<1 ng L−1; Fig. 8a), while OIT was found in the railing, in the roof foil, and in the roof access (max 12 ng L−1). The significance of the results of the elution experiments of roof materials is limited due to the fact that three materials were only sampled and measured once (roof access, roof cladding, and elevator shaft foil and grass foil). Still, these low concentrations did not suggest a primary source as it was indicated by the findings in the rain downpipes. However, elution experiments at parts of the inner roof facade yielded very high concentrations (2.7 µg L−1 diuron, 2.6 µg L−1 diuron-desmethyl, and 1.9 µg L−1 OIT; Fig. 8b). This inner roof facade exists at all houses, but at house 4 a 5 m2 westward-facing part (approximately 10 m long and 0.5 m high) was repainted in August 2018. Thus, high concentrations of detected biocides are likely due to the new paint. The western exposure suggests a higher emission rate of biocides due to a higher amount of wind-driven rain at the weather side (Vega-Garcia et al., 2020). Diuron-desmethyl was presumably formed as a photo TP at this facade. Additionally, diuron, diuron-desmethyl, and OIT were found on the northern side of the roof facade, at an area of about 10 m2 (Fig. 8c). Concentrations were much lower (diuron 48 ng L−1; diuron-desmethyl 29 ng L−1; OIT 25 ng L−1) than at the newly painted area. On the southern side of the roof facade, only OIT was detected in low concentrations (7 ng L−1). Terbutryn was only found in two elution experiments of the roof material and roof facade. However, terbutryn and two TPs were found at all investigated rain downpipes in low concentrations. It may, thus, be assumed that terbutryn is used in the railing and possibly also in other roof materials and is still leached. With the current test design, this could not be determined in more detail.
3.2.3 Estimation of biocide emissions over 2 years
This section evaluates the role of newly painted facades for biocide emission from house 4. Findings at the newly painted roof facade at house 4 suggested a locally limited source with a high impact. Other facades could be disregarded, since elution tests showed biocide emissions an order of magnitude lower than for the newly painted roof facade (Figs. 6 and 8c). This conforms with existing knowledge, since most leaching takes place within the first months after painting (Burkhardt et al., 2012). Table 4 shows the results of calculated 2-year biocide emissions (BEs) for diuron, terbutryn, OIT, and diuron-desmethyl. Biocide leaching percentage for diuron-desmethyl was related to its parent compound diuron. Although we did not account for factors influencing leaching (e.g., variable meteorological conditions, the unknown composition of the used paint, leaching kinetics, and other parts of the roof that might contribute to leaching), our estimated leaching percentages (BL) compared to reported values from experiments under natural weather conditions. Burkhardt et al. (2012) showed a leaching of 3.5 % for terbutryn, 13.4 % of diuron, and 3.9 % of OIT 12 months after initial painting. This matches our results, since diuron also showed the highest leaching percentage. Other studies quantified leaching 18 months after initial painting and reported 3 % for terbutryn (Bollmann et al., 2016) and 2.8 % for OIT (Bollmann et al., 2017b). The existing studies investigated artificial facades at artificial walls (Bollmann et al., 2016, 2017b) or facades at a model house (Burkhardt et al., 2012) but no real-world case. Our study, thus, confirms the realism of the artificial experiments.
Table 4Estimated 2-year biocide emission from 10 m2 rooftop facade at house 4 with BE as net biocide emissions, BI initial biocide concentration at facade, and BL biocide leaching percentage.
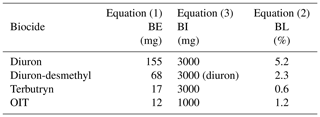
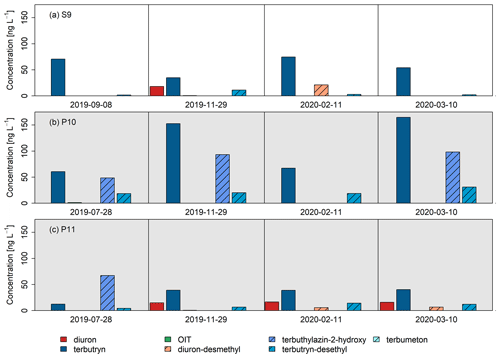
Figure 9Samples in drainage pipes during four events. (a) Pipe S9 collects street runoff. (b) Pipes P10 and (c) P11 collect infiltrated water around the houses (percolation water).
We have to admit that the accuracy of these estimations is limited due to various reasons. First, we only applied the literature values to quantify the amount of used color and its biocidal content. Second, we used precipitation data from a weather station located 5 km away. Third, we did not have information about wind-driven rain at the investigated facade, although this is an important factor regarding biocide emission (Burkhardt et al., 2012). Fourth, we disregarded variable light intensity or dry periods between rain events. Fifth, we approximated the receding biocide concentrations in rain downpipes (Fig. 7) by the mean values of four events. Sixth, we did not have information about initial biocide leaching prior to our sampling period and extrapolated mean concentrations to the entire period of 2 years. Regardless of these uncertainties, we arrived at a realistic order of magnitude, and we, hence, consider our approach promising for an initial estimation of relevant biocide sources by a limited number of samples.
3.2.4 Entry pathways of biocides from buildings into the swale (step 2 – part 2)
The three investigated drainage pipes S9 (surface runoff), P10, and P11 (percolation water) are entry pathways for emitted biocides into the swale. Terbutryn was detected in all pipes during all four sampled events, while diuron was found in percolation water pipe P11 during three events and in surface runoff pipe S9 during one event (Fig. 9). As in the swale (Fig. 5), OIT was not detected at all, probably due to fast degradation in the soil passage (Bollmann et al., 2017b). OIT concentrations at the facades were very low (Fig. 6); thus, we did not expect to find OIT in the surface runoff pipe S9.
Detected biocides in percolation water pipes P10 and P11 were different, probably due to different paints and renders used in the corresponding connected areas. The biocide concentrations of individual products may also vary (Sauer, 2017). Another reason might be a different dilution before reaching the outlet of the drainage pipe due to possible differences in the pipe system leading to the individual drainage pipe outlets. Additional sources may also affect the system since infiltrated water from gardens and terraces around the building accumulates on top of the underground parking garage and reaches the drainage pipes. This could include biocides used on terraces or garden furniture, although inhabitants denied having used biocides. Diuron and terbutryn are authorized for use in coatings, which includes terraces or furniture coating (European Parliament and Council, 2013).
Diuron-desmethyl was detected only during one event in surface runoff pipe S9 and during two events in percolation water pipe P11. Concentrations of diuron-desmethyl were lower in P11 than in S9. This might be explained by higher dilution effects in the percolation water pipe P11 or by enhanced degradation during soil passage. Diuron-desmethyl is reported to be a photo TP that can possibly be formed additionally by microorganisms in soil (Hensen et al., 2018).
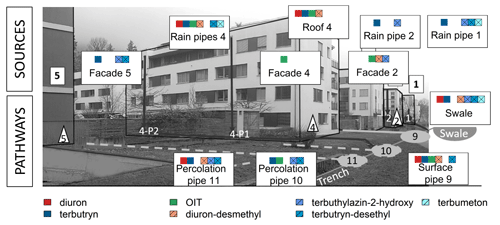
Figure 10Qualitative overview of biocides and their TPs in the investigated water infrastructures. Shown colors at sampling points represent detected substances. For the legend of the background picture, refer to Fig. 3.
TPs of terbutryn were detected in all pipes, although there was a difference between surface runoff and percolation water pipes regarding the number of detected terbutryn TPs. We detected terbutryn-desethyl in all three drainages during all events. The different appearance of the other two TPs of terbutryn implied different degradation processes in pipes S and P. Terbuthylazine-2-hydroxy was only found in percolation water pipes P10 and P11 (with soil passage) and not in S9 (without soil passage). This suggests the preferred formation of terbuthylazine-2-hydroxy is by biodegradation, although photo transformation could not be excluded, since both terbutryn TPs were already found at the sources (Figs. 5 and 6). Concentrations in the swale were in a similar range, which suggests similar input concentrations and degradation processes. Terbumeton was not found in samples in the drainage pipes, although it was detected in the swale.
For terbutryn, concentrations of both the parent compound and its TPs were highest in percolation water pipe P10. Biochemical transformation in soil is important, and TPs are obviously formed; our findings suggest that the parent compound also poses a risk to groundwater by diffuse infiltration of contaminated runoff from urban settings. Much less transformation of terbutryn takes place along surface pathways, represented by surface runoff pipe S9 where overall concentrations of TPs were smaller and terbuthylazine-2-hydroxy was not found.
3.3 Use of two-step approach for efficient monitoring
Some studies used extensive flow proportional sampling to calculate loads (Bollmann et al., 2014b; Wicke et al., 2015; Paijens et al., 2021). Concentrations of biocides can vary within an event including first flush dynamics (Bollmann et al., 2014b). To limit analytical costs, we did not assess overall biocide loads but rather stated with single samples at the outlet to document the overall relevance of biocide emission and then focused on sources and transformation along pathways. Here particularly TPs can give additional information, as shown in Fig. 10, which gives a qualitative overview of detected substances at the different sampling points. Future studies might focus on loads of biocides and TPs in order to understand the cumulated entry into the environment over longer time periods. Sampling methods, such as passive sampler, may also help to reduce the expenses and monitor more substances over a larger area (Gallé et al., 2020) or multiple catchments (Pinasseau et al., 2020).
We also show which infrastructure or area is important and should be considered for reducing the risk of biocide pollution. We identified sources both by sampling rainwater downpipes and by conducting artificial elution experiments. This might be an advantage when considering the transfer of our approach to other catchments due to an easy adaptation to existing buildings. We found additional biocide sources besides the main building facades by targeted elution experiments of various roof materials on a contaminated flat roof. This stresses the fact of considering the entire building, including roof areas, as a potential source of biocides. In our case, a small repainted roof facade was identified as a major biocide source, even 13 years after construction of the building had been completed. Therefore, existing buildings must be regarded as continuous biocide sources as they not only originate from old facades, as shown by Hensen et al. (2018), but also due to sporadic repair on restricted areas. The location of the roof facade on top of a flat roof permits a rough estimation of long-term biocide leaching with only a minimum number of samples at the rain downpipes. The obtained results are in the same order of magnitude as previous studies of artificial walls under natural weather conditions (Burkhardt et al., 2012; Bollmann et al., 2016, 2017b). Hence, we advocate the use of existing urban building infrastructure, i.e., flat roofs and rainwater downpipes, to efficiently collect long-term realistic data on biocide wash-off with only a minimum sampling effort. This approach cannot be used to close mass balances since there is not enough information on initial inputs or complete biocide loads during individual events. With more data available, such as wind-driven rain or initial biocide usage, such data could also be used to calibrate existing physically based models on potential leaching from buildings (Tietje et al., 2018) or from larger urban areas (Coutu et al., 2012).
Finally, we investigated entry pathways, and the obtained results suggest effects of the soil passage on biocide breakthrough to groundwater and on biocide transformation. We used existing urban infrastructure, in this case the collection of areal infiltration by a drainage system on top of an underground parking garage. Through this, our study adds to the few available studies that investigate the risk of biocide entry into groundwater in urban areas (Hensen et al., 2018).
Our approach can principally be adapted to other areas of commonly built modern urban districts, where separated sewer systems, thermal insulation of buildings, and modern architecture with flat roofs and limited roof overhang prevail and promote biocide emission. With a limited number of samples and analyzed substances especially small-scale districts can be characterized regarding their potential risk of biocide emissions. This shows new possibilities for reducing the pollutant entry in a targeted manner and for taking measures at the source (Kümmerer et al., 2018).
Following the introduced method, we first confirmed the relevance of biocide emission in the investigated urban system by spot sampling during four events in a swale. Receiving urban infiltration systems (e.g., swales, swale–trench systems, and retention ponds) at the outlet of a catchment generally provide an integrated signal of the aquatic system of a larger area. Thereafter, source areas were identified, again with a limited number of samples. Artificial elution experiments confirmed expected sources, i.e., facades. Some facades showed only TPs but no biocides. TPs may help to identify previously used substances and can, thus, complete the picture of biocide use on facades. Besides facades, we found additional sources through sampling of rain downpipes from flat roofs. In our case, high concentrations in one downpipe helped to identify a small recently painted roof facade as being a primary biocide source. We, therefore, advocate the sampling of rain downpipes as this can help to identify additional sources and also facilitate estimations of emitted biocide loads, since volumes of roof runoff can easily be estimated when rainfall is known. Monitored drainage pipes characterized entry pathways from buildings to the swale and suggested differences in biocide transformation due to soil passage. Yet, all pipes showed concentrations of terbutryn, regardless of whether there is a pathway through soil or not. This shows the risk that biocides are not necessarily degraded on their way to groundwater. For surface water, our study confirmed the potential environmental risk of biocide use, since concentrations at the outlet of our urban catchment exceeded PNEC values at one event. This means that biocides were emitted into the urban environment more than a decade after construction had ended. Hence, biocide pollution is not limited to newly built areas but is a continuous and omnipresent problem relevant for all urban areas. We expect that our parsimonious approach can easily be adopted to other cities to evaluate the risk of biocide pollution.
We produced the figures with R (http://www.rstudio.com/, last access: 16 August 2021) (RStudio Team, 2020).
Sampling data generated during this study are included in this article. Measured concentrations of substances can be found in Table S2 in the Supplement. GIS data for Freiburg were kindly provided by Stadt Freiburg i. Br. Weather data for Freiburg are available from the Deutsche Wetterdienst.
The supplement related to this article is available online at: https://doi.org/10.5194/hess-25-4495-2021-supplement.
FL sampled and visualized the measured data and prepared most of the paper in cooperation with JL and OO. JL, FP, FL, and MB were responsible for the conceptualization. LS, OO, and KK facilitated the sample analysis, and LS wrote Sect. 2.4. All authors were involved in the editing and revision of the paper.
The authors declare that they have no conflict of interest.
Publisher's note: Copernicus Publications remains neutral with regard to jurisdictional claims in published maps and institutional affiliations.
This research was funded by the EU within the European Regional Development Fund (ERDF), support measure INTERREG V in the Upper Rhine as part of the NAVEBGO project 5.3 (sustainable reduction of biocide inputs to groundwater in the Upper Rhine region).
This open-access publication was funded by the University of Freiburg.
This paper was edited by Christian Stamm and reviewed by Ute Schoknecht, Adèle Bressy, and one anonymous referee.
Bergek, J., Andersson Trojer, M., Mok, A., and Nordstierna, L.: Controlled release of microencapsulated 2-n-octyl-4-isothiazolin-3-one from coatings: Effect of microscopic and macroscopic pores, Colloid. Surf. A, 458, 155–167, https://doi.org/10.1016/j.colsurfa.2014.02.057, 2014.
Bollmann, U. E., Tang, C., Eriksson, E., Jönsson, K., Vollertsen, J., and Bester, K.: Biocides in urban wastewater treatment plant influent at dry and wet weather: concentrations, mass flows and possible sources, Water Res., 60, 64–74, https://doi.org/10.1016/j.watres.2014.04.014, 2014a.
Bollmann, U. E., Vollertsen, J., Carmeliet, J., and Bester, K.: Dynamics of biocide emissions from buildings in a suburban stormwater catchment – concentrations, mass loads and emission processes, Water Res., 56, 66–76, https://doi.org/10.1016/j.watres.2014.02.033, 2014b.
Bollmann, U. E., Minelgaite, G., Schlüsener, M., Ternes, T., Vollertsen, J., and Bester, K.: Leaching of Terbutryn and Its Photodegradation Products from Artificial Walls under Natural Weather Conditions, Environ. Sci. Technol., 50, 4289–4295, https://doi.org/10.1021/acs.est.5b05825, 2016.
Bollmann, U. E., Fernández-Calviño, D., Brandt, K. K., Storgaard, M. S., Sanderson, H., and Bester, K.: Biocide Runoff from Building Facades: Degradation Kinetics in Soil, Environ. Sci. Technol., 51, 3694–3702, https://doi.org/10.1021/acs.est.6b05512, 2017a.
Bollmann, U. E., Minelgaite, G., Schlüsener, M., Ternes, T. A., Vollertsen, J., and Bester, K.: Photodegradation of octylisothiazolinone and semi-field emissions from facade coatings, Scient. Rep., 7, 41501, https://doi.org/10.1038/srep41501, 2017b.
Bollmann, U. E., Bester, K., Vollertsen, J., Urbanczyk, M. M., and Rudelle, E. A.: Transport and transformation of biocides in construction materials: Factors controlling release and emissions, Pesticide Research, 177, Ministry of Environment and Food of Denmark, Environmental Protection Agency, Copenhagen, 110 pp., 2018.
Bucheli, T. D., Müller, S. R., Voegelin, A., and Schwarzenbach, R. P.: Bituminous Roof Sealing Membranes as Major Sources of the Herbicide (R S)-Mecoprop in Roof Runoff Waters: Potential Contamination of Groundwater and Surface Waters, Environ. Sci. Technol., 32, 3465–3471, https://doi.org/10.1021/es980318f, 1998.
Burkhardt, M., Kupper, T., Hean, S., Haag, R., Schmid, P., Kohler, M., and Boller, M.: Biocides used in building materials and their leaching behavior to sewer systems, Water Sci. Technol., 56, 63–67, https://doi.org/10.2166/wst.2007.807, 2007.
Burkhardt, M., Zuleeg, S., Vonbank, R., Schmid, P., Hean, S., Lamani, X., Bester, K., and Boller, M.: Leaching of additives from construction materials to urban storm water runoff, Water Sci. Technol., 63, 1974–1982, https://doi.org/10.2166/wst.2011.128, 2011.
Burkhardt, M., Zuleeg, S., Vonbank, R., Bester, K., Carmeliet, J., Boller, M., and Wangler, T.: Leaching of biocides from façades under natural weather conditions, Environ. Sci. Technol., 46, 5497–5503, https://doi.org/10.1021/es2040009, 2012.
Burri, N. M., Weatherl, R., Moeck, C., and Schirmer, M.: A review of threats to groundwater quality in the anthropocene, Sci. Total Environ., 684, 136–154, https://doi.org/10.1016/j.scitotenv.2019.05.236, 2019.
Coutu, S., Rota, C., Rossi, L., and Barry, D. A.: Modelling city-scale facade leaching of biocide by rainfall, Water Res., 46, 3525–3534, https://doi.org/10.1016/j.watres.2012.03.064, 2012.
DIN 1986-100: Drainage systems on private ground – Part 100: Specifications in relation to DIN EN 752 and DIN EN 12056: DIN 1986-100, 2016.
Donati, L. and Funari, E.: Review of leaching characteristics of triazines and their degradation products, Ann. 1st Super. Sanita, 29, 225–241, 1993.
Doppler, T., Camenzuli, L., Hirzel, G., Krauss, M., Lück, A., and Stamm, C.: Spatial variability of herbicide mobilisation and transport at catchment scale: insights from a field experiment, Hydrol. Earth Syst. Sci., 16, 1947–1967, https://doi.org/10.5194/hess-16-1947-2012, 2012.
ECHA: List of biocidal active substances, available at: https://echa.europa.eu/de/information-on-chemicals/biocidal-active-substances (last access: 9 March 2021), 2007–2020.
European Parliament and Council: Regulation (EU) No. 528/2012 of the European Parliament and of the Council of 22 May 2012 concerning the making available on the market and use of biocidal products, Text with EEA relevance: EU No. 528/2012, Luxembourg, 123 pp., 2012.
European Parliament and Council: Directive 2013/39/EU of the European Parliament and of the Council of 12 August 2013 amending Directives 2000/60/EC and 2008/105/EC as regards priority substances in the field of water policyText with EEA relevance, Luxembourg, 17 pp., 2013.
Fenner, K., Canonica, S., Wackett, L. P., and Elsner, M.: Evaluating pesticide degradation in the environment: blind spots and emerging opportunities, Science, 341, 752–758, https://doi.org/10.1126/science.1236281, 2013.
Foster, S. and Cogu, R. C.: Urban Groundwater – mobilising stakeholders to improve monitoring, The Source, available at: https://www.thesourcemagazine.org/urban-groundwater-mobilising-stakeholders-to-improve-monitoring/ (last access: 9 March 2021), 2019.
Gallé, T., Bayerle, M., Pittois, D., and Huck, V.: Allocating biocide sources and flow paths to surface waters using passive samplers and flood wave chemographs, Water Res., 173, 115533, https://doi.org/10.1016/j.watres.2020.115533, 2020.
Gasperi, J., Sebastian, C., Ruban, V., Delamain, M., Percot, S., Wiest, L., Mirande, C., Caupos, E., Demare, D., Kessoo, M. D. K., Saad, M., Schwartz, J. J., Dubois, P., Fratta, C., Wolff, H., Moilleron, R., Chebbo, G., Cren, C., Millet, M., Barraud, S., and Gromaire, M. C.: Micropollutants in urban stormwater: occurrence, concentrations, and atmospheric contributions for a wide range of contaminants in three French catchments, Environ. Sci. Pollut. Res. Int., 21, 5267–5281, https://doi.org/10.1007/s11356-013-2396-0, 2013.
Gassmann, M., Stamm, C., Olsson, O., Lange, J., Kümmerer, K., and Weiler, M.: Model-based estimation of pesticides and transformation products and their export pathways in a headwater catchment, Hydrol. Earth Syst. Sci., 17, 5213–5228, https://doi.org/10.5194/hess-17-5213-2013, 2013.
Giacomazzi, S. and Cochet, N.: Environmental impact of diuron transformation: a review, Chemosphere, 56, 1021–1032, https://doi.org/10.1016/j.chemosphere.2004.04.061, 2004.
Greiwe, J., Olsson, O., Kümmerer, K., and Lange, J.: Pesticide peak concentration reduction in a small vegetated treatment system controlled by chemograph shape, Hydrol. Earth Syst. Sci., 25, 497–509, https://doi.org/10.5194/hess-25-497-2021, 2021.
Hensen, B., Lange, J., Jackisch, N., Zieger, F., Olsson, O., and Kümmerer, K.: Entry of biocides and their transformation products into groundwater via urban stormwater infiltration systems, Water Res., 144, 413–423, https://doi.org/10.1016/j.watres.2018.07.046, 2018.
Hensen, B., Olsson, O., and Kümmerer, K.: A strategy for an initial assessment of the ecotoxicological effects of transformation products of pesticides in aquatic systems following a tiered approach, Environ. Int., 137, 105533, https://doi.org/10.1016/j.envint.2020.105533, 2020.
Jirkovský, J., Faure, V., and Boule, P.: Photolysis of Diuron, Pestic. Sci., 50, 42–52, https://doi.org/10.1002/(SICI)1096-9063(199705)50:1<42::AID-PS557>3.0.CO;2-W, 1997.
Johann, S., Schwerd, R., and Scherer, C. R.: Verhalten von aus Baustoffen ausgelaugten Bioziden bei der Bodenpassage, Bauphysik, 40, 369–378, https://doi.org/10.1002/bapi.201800006, 2018.
Jungnickel, C., Stock, F., Brandsch, T., and Ranke, J.: Risk assessment of biocides in roof paint, Environ. Sci. Pollut. Res., 15, 258–265, https://doi.org/10.1065/espr2007.12.465, 2008.
Kümmerer, K., Dionysiou, D. D., Olsson, O., and Fatta-Kassinos, D.: A path to clean water, Science, 361, 222–224, https://doi.org/10.1126/science.aau2405, 2018.
Lechón, Y., Sánchez-Brunete, C., and Tadeo, J. L.: Influence of the Laboratory Incubation Method on Chlorotoluron and Terbutryn Degradation in Soil, J. Agric. Food Chem., 45, 951–954, 1997.
Moschet, C., Wittmer, I., Simovic, J., Junghans, M., Piazzoli, A., Singer, H., Stamm, C., Leu, C., and Hollender, J.: How a complete pesticide screening changes the assessment of surface water quality, Environ. Sci. Technol., 48, 5423–5432, https://doi.org/10.1021/es500371t, 2014.
Musgrave, H., Tattersall, C., Clarke, S., and Comber, S.: Technical Support for the Impact Assessment of the Review of Priority Substances under Directive 2000/60/EC: Substance Assessment: Terbutryn, European Commission (DG Environment), London, 59 pp., 2011.
Paijens, C., Bressy, A., Frère, B., and Moilleron, R.: Biocide emissions from building materials during wet weather: identification of substances, mechanism of release and transfer to the aquatic environment, Environ. Sci. Pollut. Res. Int., 27, 3768–3791, https://doi.org/10.1007/s11356-019-06608-7, 2019.
Paijens, C., Bressy, A., Frère, B., Tedoldi, D., Mailler, R., Rocher, V., Neveu, P., and Moilleron, R.: Urban pathways of biocides towards surface waters during dry and wet weathers: Assessment at the Paris conurbation scale, J. Hazard. Mater., 402, 123765, https://doi.org/10.1016/j.jhazmat.2020.123765, 2021.
Peck, D. E., Corwin, D. L., and Farmer, W. J.: Adsorption-Desorption of Diuron by Freshwater Sediments, J. Environ. Qual., 9, 101–106, 1980.
Pinasseau, L., Wiest, L., Volatier, L., Mermillod-Blondin, F., and Vulliet, E.: Emerging polar pollutants in groundwater: Potential impact of urban stormwater infiltration practices, Environ. Pollut., 266, 115387, https://doi.org/10.1016/j.envpol.2020.115387, 2020.
Quednow, K. and Püttmann, W.: Monitoring terbutryn pollution in small rivers of Hesse, Germany, J. Environ. Monit., 9, 1337–1343, https://doi.org/10.1039/b711854f, 2007.
Reemtsma, T., Alder, L., and Banasiak, U.: Emerging pesticide metabolites in groundwater and surface water as determined by the application of a multimethod for 150 pesticide metabolites, Water Res., 47, 5535–5545, https://doi.org/10.1016/j.watres.2013.06.031, 2013.
Reichenberger, S., Bach, M., Skitschak, A., and Frede, H.-G.: Mitigation strategies to reduce pesticide inputs into ground- and surface water and their effectiveness; a review, Sci. Total Environ., 384, 1–35, https://doi.org/10.1016/j.scitotenv.2007.04.046, 2007.
RStudio Team: Integrated Development for R, RStudio, PBC, Boston, MA, available at: http://www.rstudio.com/, last access: 16 August 2021.
Sauer, F.: Microbicides in Coatings, Vincentz Network, Hanover, 143 pp., 2017.
Schoknecht, U., Gruycheva, J., Mathies, H., Bergmann, H., and Burkhardt, M.: Leaching of biocides used in façade coatings under laboratory test conditions, Environ. Sci. Technol., 43, 9321–9328, https://doi.org/10.1021/es9019832, 2009.
Schoknecht, U., Sommerfeld, T., Borho, N., and Bagda, E.: Interlaboratory comparison for a laboratory leaching test procedure with façade coatings, Prog. Organ. Coat., 76, 351–359, https://doi.org/10.1016/j.porgcoat.2012.10.001, 2013.
Schoknecht, U., Mathies, H., and Wegner, R.: Biocide leaching during field experiments on treated articles, Environ. Sci. Eur., 28, 2850, https://doi.org/10.1186/s12302-016-0074-9, 2016.
Stadt Freiburg i. Br.: Satzung über den Bebauungsplan Wohngebiet östlich Wiehre-Bahnhof/ Ausgleichsflächen am Tuniberg – Plan-Nr. 4-62: Plan-Nr. 4–62, Freiburg i. Br., 3 pp., 2005.
Styszko, K., Bollmann, U. E., and Bester, K.: Leaching of biocides from polymer renders under wet/dry cycles–Rates and mechanisms, Chemosphere, 138, 609–615, https://doi.org/10.1016/j.chemosphere.2015.07.029, 2015.
Talja, K. M., Kaukonen, S., Kilpi-Koski, J., Malin, I., Kairesalo, T., Romantschuk, M., Tuominen, J., and Kontro, M. H.: Atrazine and terbutryn degradation in deposits from groundwater environment within the boreal region in Lahti, Finland, J. Agricult. Food Chem., 56, 11962–11968, https://doi.org/10.1021/jf802528a, 2008.
Tietje, O., Burkhardt, M., Rohr, M., Borho, N., and Schoknecht, U.: Emissions- und Übertragungsfunktionen für die Modellierung der Auslaugung von Bauprodukten, 28, Umweltbundesamt – UBA, Dessau-Roßlau, 59 pp., 2018.
Uhlig, S., Colson, B., and Schoknecht, U.: A mathematical approach for the analysis of data obtained from the monitoring of biocides leached from treated materials exposed to outdoor conditions, Chemosphere, 228, 271–277, https://doi.org/10.1016/j.chemosphere.2019.04.102, 2019.
Urbanczyk, M. M., Bollmann, U. E., and Bester, K.: Partition of biocides between water and inorganic phases of renders with organic binder, Sci. Total Environ., 573, 639–644, https://doi.org/10.1016/j.scitotenv.2016.08.129, 2016.
Urbanczyk, M. M., Bester, K., Borho, N., Schoknecht, U., and Bollmann, U. E.: Influence of pigments on phototransformation of biocides in paints, J. Hazard. Mater., 364, 125–133, https://doi.org/10.1016/j.jhazmat.2018.10.018, 2019.
Vega-Garcia, P., Schwerd, R., Scherer, C., Schwitalla, C., Johann, S., Rommel, S. H., and Helmreich, B.: Influence of façade orientation on the leaching of biocides from building façades covered with mortars and plasters, Sci. Total Environ., 734, 139465, https://doi.org/10.1016/j.scitotenv.2020.139465, 2020.
Wangler, T. P., Zuleeg, S., Vonbank, R., Bester, K., Boller, M., Carmeliet, J., and Burkhardt, M.: Laboratory scale studies of biocide leaching from façade coatings, Build. Environ., 54, 168–173, https://doi.org/10.1016/j.buildenv.2012.02.021, 2012.
Wicke, D., Matzinger, A., and Rouault, P.: Relevanz organischer Spurenstoffe im Regenwasserabfluss Berlins, Kompetenzzentrum Wasser, Berlin, 109 pp., 2015.
Wieck, S., Olsson, O., and Kümmerer, K.: Not only biocidal products: Washing and cleaning agents and personal care products can act as further sources of biocidal active substances in wastewater, Environ. Int., 115, 247–256, https://doi.org/10.1016/j.envint.2018.03.040, 2018.
Wittmer, I. K., Bader, H.-P., Scheidegger, R., Singer, H., Lück, A., Hanke, I., Carlsson, C., and Stamm, C.: Significance of urban and agricultural land use for biocide and pesticide dynamics in surface waters, Water Res., 44, 2850–2862, https://doi.org/10.1016/j.watres.2010.01.030, 2010.
Wu, C.-H., Santelmann, P. W., and Davidson, J. M.: Influence of Soil Temperature and Moisture on Terbutryn Activity and Persistence, Weed Sci., 22, 571–574, 1974.