the Creative Commons Attribution 4.0 License.
the Creative Commons Attribution 4.0 License.
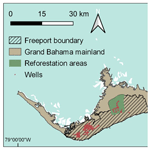
Technical assessment combined with an extended cost–benefit analysis for the restoration of groundwater and forest ecosystem services – an application for Grand Bahama
Anne Imig
Francesca Perosa
Carolina Iwane Hotta
Sophia Klausner
Kristen Welsh
Yan Zheng
A large storm surge caused by Hurricane Dorian in 2019 resulted in extensive flooding and saltwater intrusion into the aquifers of the island of Grand Bahama. This caused about 40 % of the island's water supply to become brackish with no or slow recovery to date and damage to more than 70 % of mangroves and forests on Grand Bahama. Managed aquifer recharge (MAR) and reforestation were considered nature-based solutions (NBS) to mitigate the impacts of Hurricane Dorian. First, a technical assessment of MAR investigated (hydro)geological aspects. As a result, potential locations for a MAR scheme are proposed. Further, a financial and an extended cost–benefit analysis (CBA) integrating ecosystem service (ES) assessments are conducted for proposed MAR and reforestation measures. Based on the current data availability, results indicate that the MAR scheme of rooftop rainwater harvesting is technically feasible. However, based on our first estimate with limited data, this MAR scheme will be able to provide only about 10 % of the water demand in the study area and thus would not be favorable from a financial perspective. Since MAR has a range of positive aspects (including potential reduction of desalinization efforts and improvement freshwater-dependent ecosystems), we recommend reassessment with more detailed hydrogeological data. On the other hand, reforestation measures are assessed to be financially profitable. The results of this study not only prove the technical feasibility and the added value of restoring groundwater and the forest ecosystem on Grand Bahama, but also highlight the associated high costs. The developed methods for investigating ecosystem services from an economic perspective was proven to allow for a systematic comparison of NBSs and reverse osmosis costs and benefits helping, e.g., policy- and decision-makers and to justify their implementation.
- Article
(4122 KB) - Full-text XML
-
Supplement
(582 KB) - BibTeX
- EndNote
The consequences of the Anthropocene, in particular climate change and the resulting impacts, are negatively affecting small islands and their water resources. These effects will continue to be observed in future decades (Thomas et al., 2020). Freshwater aquifers on small islands manifest as thin lenses and are sustained solely by recharge from rainfall. The freshwater lenses float atop more saline groundwater from seawater (Ault, 2016; Bedekar et al., 2019). Wave-induced overwash leads to the infiltration of saltwater into the freshwater lenses, which becomes more frequent with sea-level rise and increasing frequency and intensity of hurricanes (Emanuel, 2020; Terry and Falkland, 2010; Vecchi et al., 2021). Both island inhabitants and terrestrial ecosystems (including forests) rely heavily on these fragile and limited aquifers, making this resource finite and vulnerable (Diamond and Melesse, 2016; Morgan and Werner, 2014).
Grand Bahama (GB) is a primarily low-lying island in the archipelago of The Bahamas and in the north Atlantic hurricane belt. The island is particularly vulnerable to sea-level rise and wave-induced overwash events because approximately 80 % of the land surface elevation is lower than 1 m a.s.l. (Department of Statistics, 2012; ICF and BEST, 2001; Whitaker and Smart, 1997b).
Hurricane Dorian struck GB in September 2019. It was one of the most devastating natural disasters that The Bahamas has experienced to date, with damage amounting to a quarter of the country's gross domestic product. It stalled over GB and the neighboring Abaco Islands for more than 24 h, exerting extremely high wind speeds and covering more than half of GB under its storm surge and associated flooding (SWA and WES, 2019; UNECLAC, 2021; Zegarra et al., 2020). This resulted in widespread saltwater intrusion into the shallow freshwater aquifers. Approximately 40 % of the water supply became brackish, and 30 % of the population still lacks a supply with potable water to date. In addition, stagnant saline water and high wind caused extensive destruction of trees, mangroves, seagrass beds, and coral reefs. It was estimated that 73 % of mangrove habitats and 77 % of forests in GB were damaged (Bahamas National Trust, 2020). Al Baghdadi (2021) predicted the natural recovery of the groundwater system will take approximately 20 years.
Efforts were initiated to mitigate the devastating impacts of Hurricane Dorian on groundwater and the forest ecosystem as these provide services of immense societal and economic value. Freshwater aquifers are the only source of drinking water supply on GB that sustain the water demand of the local population and the economy, which is primarily based on tourism. Further, forest protection and restoration are critical for mitigating climate change and its impacts (van Oosterzee et al., 2020) and stabilizing groundwater recharge and quality (Ellison, 2018).
After Hurricane Dorian, the Grand Bahama Utility Company (GBUC) announced the installation of a reverse osmosis (RO) system to reduce water salinity to World Health Organization (WHO) standards and create a sustainable and resilient contingency plan in the event of another hurricane (GBUC, 2020, 2021). The RO system was fully operational from December 2021, but to date (October 2023), the water supplied to some households is not yet potable according to WHO standards. Apart from the shortcomings in the quality of supplied water for drinking water purpose, pipes and faucets are corroding in the Bahamians' households due to the water's high salinity. Further, RO is a piece of highly energy-consuming technology for drinking water treatment. Consequently, a major concern for the system is the cost dictated by energy consumption added to the membrane replacement costs (Dillon, 2005; Garfí et al., 2016).
Furthermore, hurricanes can severely damage infrastructure and cause disruptions in the energy supply, leading to damage or inoperability of the RO system. Therefore, alternative, complementary measures should be used to restore and preserve the existing freshwater resources instead of entirely depending on desalination.
Nature-based solutions (NBSs) could be an approach to maintain the drinking water supply on GB and restore the forest ecosystem sustainably and resiliently. According to Cohen-Shacham et al. (2016), NBSs are actions to manage and restore natural ecosystems that address societal challenges (e.g., climate change, water security, or natural disasters) effectively and adaptively, providing human well-being and biodiversity benefits. NBSs are gaining acceptance as a more sustainable solution to mitigate and adapt to the effects of climate change by reducing exposure to natural hazards and vulnerability to hazardous events (Sudmeier-Rieux et al., 2019, 2021). They typically are considered cost-effective and viable solutions to optimize the properties of natural ecosystems and can be integrated with technological and engineering solutions (Cohen-Shacham et al., 2016; Lupp et al., 2021).
Within this study, two NBS measures on GB, planned by different stakeholders, are assessed to mitigate the impacts of Hurricane Dorian: managed aquifer recharge and reforestation. Managed aquifer recharge (MAR) is a NBS increasingly deployed in the last few decades to tackle saltwater intrusion and climate change effects on groundwater resources. Excess water from other sources, e.g., rainfall/flooding, water treatment plants, rivers, or desalinated seawater, can infiltrate an aquifer to store and recharge groundwater (e.g., Dillon et al., 2019; Gale, 2005; Raicy et al., 2012). For small islands, reports on MAR implementation are scarce (Hejazian et al., 2017).
Cost–benefit analysis (CBA) has been applied in existing literature to assess the economic feasibility of MAR projects (e.g., Halytsia et al., 2022; Rupérez-Moreno et al., 2017) but has not included ecosystem services (ESs), as part of the highlighted benefits of NBSs. Furthermore, the CBA method, and the way it has been recommended to be conducted in the past, falls short in adequately monetizing ecosystems services (e.g., Maliva, 2014; Ruangpan et al., 2020; Network Nature, 2022; Sudmeier-Rieux et al., 2021; Wegner and Pascual, 2011). In fact, by definition, a CBA should be able to consider all benefits and costs of a measure by translating social, environmental, and economic aspects into monetary values (Clinch, 2004; Hanley, 2013). Often, however, only partial benefits of a measure are included in a CBA, especially marketed values (Clinch, 2004), thereby neglecting ethical and cultural aspects (Vojinovic et al., 2017) and implicitly setting all neglected benefits to zero (Dominati et al., 2014). Therefore, in this study, we propose a methodology that sets itself apart from already published research as it aims to combine a technical feasibility assessment and to use the results to assess them in an extended cost–benefit analysis (extended CBA) with ecosystem service analysis. Ecosystem services are modeled with the InVEST software (NCP, 2024). In other words, a standard CBA focusses on the analysis within the boundaries of the technosphere, i.e., the sphere of human-made technologies and systems, while the extended CBA encompasses studies of the measures' effects on the hydrosphere, atmosphere, and biosphere in addition to the technosphere.
In this work, (i) a technical assessment including risk assessment of MAR on small island nations is developed. (ii) Next, a methodology for an extended CBA with ES analysis is proposed. This methodology aims to explore the feasibility of NBSs (MAR and reforestation) and RO from an economic and ecosystem service perspective. (iii) The two developed methodologies are then applied to a study case on the island of Grand Bahama, The Bahamas. This study aims to show methods for investigating ecosystem services from an economic perspective. Results aim to allow for a systematic comparison of NBS and RO costs and benefits for, e.g., policy- and decision-makers and help justify their implementation.
2.1 Study site
Grand Bahama is the northernmost island of The Bahamas. The Bahamian archipelago, with approximately 700 shallow islands and 2400 cays, is scattered over a strip of approximately 1000 km from the coast of southern Florida in the north down to Cuba and Haiti in the south (Fig. 1). All islands of The Bahamas consist predominantly of limestone, leading to long and narrow shapes and low-lying lands (ICF and BEST, 2001; Whitaker and Smart, 1997b). This includes GB, an east–west striking elongated island with a maximum elevation of 20.7 m a.s.l.
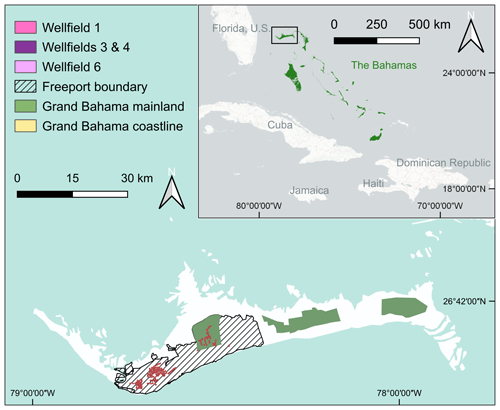
Figure 1Location of the study area and wellfields 1, 3, 4, and 6 on the island Grand Bahama, The Bahamas. Freeport boundary (black stripes) as well as Grand Bahama mainland (green) and coastline (yellow) are indicated (geographic coordinates in EPSG: 4326).
GB topography represents a gently undulating plain. The southern coast consists mainly of sand beaches with shallow reefs in front of a deep-sea basin, while the carbonate platform extends further into the northern coast, creating mangrove marshlands. The climate is classified as marine tropical, with dry winters, wet summers, and hurricane season from June to November (USACE, 2004; Whitaker and Smart, 1997). GB vegetation is typical for the northern Bahamian islands. It consists of Caribbean pine forests and palmetto palm trees in the inland, broadleaf coppice with hardwood species (especially at the windward coasts), and mangrove swamps along protected, shallow coasts. Since World War II, the primary industry on GB has been tourism, with banking, fishing, and agriculture trailing behind (ICF and BEST, 2001). The last census in 2010 revealed a total population of around 350 000 in The Bahamas, of which 51 000 (14.5 %) inhabitants live in GB, with a rising trend compared to preceding years (Department of Statistics, 2012).
GB's potable water supply is entirely provided by groundwater. Surface water is not available on the island. The average abstraction rate on GB is estimated to be 26 497 m3 d−1 (7 million gallons per day, mgd), with approximately 11 356 m3 d−1 (3 mgd) from wellfield 6 (about 43 %) and 15 141 m3 d−1 (4 mgd; about 57 %) from wellfields 1, 3, and 4 (Fig. 1) (personal communication with GBUC). Wellfield 6 is located in a low-lying rural area in the southwest of the island and was nearly fully inundated during the storm surge of Hurricane Dorian. Wellfields 1, 3, and 4 are in the city of Freeport in populated areas. The entire water supply is disinfected with chlorine.
The water from wellfield 6 has been brackish since Hurricane Dorian's storm surge. For this reason, the water has been treated with a portable reverse osmosis (RO) unit of 3 mgd capacity (equal to 43 % of the total abstracted water in GB and 30 % of water demand on the island) since the end of 2021. RO is a water treatment option for desalinization in which a partially permeable membrane separates dissolved components in water. The feed water is pressed through the membrane, removing larger dissolved components (Dos Anjos, 1998). The RO scheme is also designed to be mobile as a storm contingency plan (GBUC, 2020).
2.2 Structure of the holistic analysis
Our analysis of potential sustainability measures for GB is based on three main parts (Fig. 2). The first part addresses the technical feasibility of potential MAR measures. As the output, it provides the information about whether the tested MAR measure is technically feasible and, if so, which MAR type is the most appropriate. The second part concerns the assessment of the financial profitability of the most appropriate MAR measure compared to a portable RO scheme. The latter (MAR) and reforestation, as another sustainability measure, are assessed by means of a financial cost–benefit analysis (CBA). The third part analyzes the same measures as in the second part, but by means of an extended CBA, i.e., by including ecosystem services as additional benefits.
2.3 Sustainability measures
Managed aquifer recharge (MAR) is a nature-based solution (NBS) with the aim to quantitatively and qualitatively protect groundwater resources. Excess water from, e.g., rainfall, flooding, water treatment plants, rivers, and desalinated water can be infiltrated into an aquifer to store and recharge groundwater (e.g., Dillon et al., 2019; Gale, 2005; Raicy et al., 2012). As a result, groundwater availability is enhanced, and groundwater can be extracted in a time of need. This measure was analyzed with the technical feasibility procedure, financial CBA, and extended CBA.
Reforestation is a NBS measure that implies returning tree cover to deforested land, often intending to reinstate ecological processes at the level of climax forests (Elliott et al., 2013). Moreover, forests are essential in reducing CO2 emissions by carbon sequestration (Piyathilake et al., 2022). The reforestation measure aims at restoring the Bahamian pine trees, which are already included in an existing seedling nursery on the island (Bowen-O'Connor and Lynch, 2022). This measure was analyzed with the financial CBA and the extended CBA.
2.4 Part 1: technical feasibility assessment of managed aquifer recharge
Various guidelines exist to assess the feasibility of MAR measures in specific study areas. In this study, three independent guidelines were combined into a new procedure to assess the technical feasibility of MAR on GB (Fig. 3). These include a practical guideline for MAR in the Caribbean developed by a consortium to promote rainwater harvesting (CEHI et al., 2010), the Australian guidelines for water recycling (NRMMC, 2009a, b), and a methodology for a feasibility assessment of MAR in central Europe (DEEPWATER-CE, 2020a, b). The methodology complies with local requirements such as low-lying lands exposed to increased hurricane intensity, rising sea levels, and scarce data availability. The methodology is divided into six main steps that were organized in the form of a decision tree (Fig. 3).
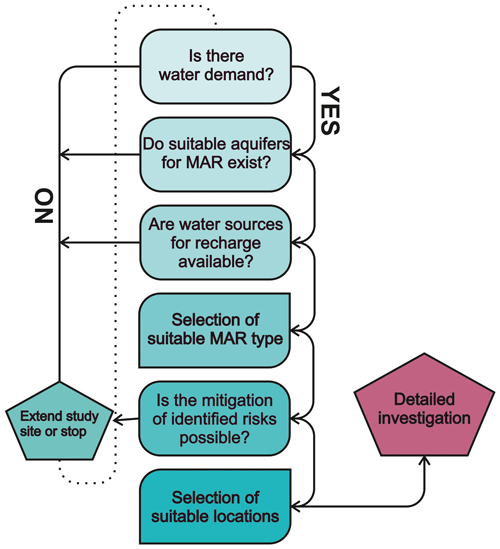
Figure 3Six-step decision tree for assessing the technical feasibility of managed aquifer recharge on the island of Grand Bahama.
As step (i), the water demand is defined as without water demand, a MAR scheme is not needed. The demand can be defined based on either technical guidelines from the country's legislation or the documented water use of the consumers. It is reasonable to predict a water demand for the design life of the MAR measure; e.g., 30 years are usually set in water supply infrastructure. We collected data from the water authorities in Grand Bahama to calculate the water demand. For step (ii), the identification of suitable aquifers, hydrogeological properties of regional aquifers were collected. Hydrogeological properties should include the lithology and location of the aquifer, storage properties, and hydraulic conductivity or transmissivity (DEEPWATER-CE, 2020a; NRMMC, 2009a). Based on the available data and the site-specific information, a suitable aquifer with sufficient storage capacity to supply the water demand shall be chosen. After defining the water demand and a suitable aquifer, in step (iii), the water source(s) for groundwater recharge should be identified, e.g., rainwater, surface water, or desalinated water. Based on the available water source, for step (iv), a suitable MAR scheme can be selected for the water demand and the available aquifers. This is necessary as, e.g., rainwater harvesting schemes have different requirements regarding groundwater levels compared to a riverbank filtration scheme (Sallwey et al., 2019). Specific criteria and data needed for their identification were determined in a literature review, which is not further summarized here but only specified for the chosen MAR type (cf. the “Results and discussion” section). For step (v), we conducted a qualitative risk assessment with a risk score matrix after Swierc et al. (2005). For the risk assessment, potential hazards relevant for a MAR scheme in Grand Bahama were chosen from a collection published in a review paper by Imig et al. (2022). For step (vi), the selection of suitable location, we developed selection criteria based on information gained from the previous steps. As in step (iv), we refrained from further specifying selection criteria and data for all possible MAR types in order to keep descriptions concise. The criteria and data for the chosen MAR scheme (in step iv) are summarized in the results section based on information from DEEPWATER-CE (2020a), CEHI et al. (2010), and NRMMC (2009a). The criteria were assessed using the geographical information system QGIS (2024) and were used in a multi-criteria decision analysis (MCDA) (Sallwey et al., 2019). The achievable recharge volume from the rainwater harvesting scheme was calculated based on recommendations by the German Institute for Standardization (DIN, 2002), where details are given in Sect. S1 in the Supplement. If steps (i)–(iii) and (v) generate a negative evaluation, we suggest extending the study area or stopping the investigation. Otherwise, if all steps can be followed and result in a positive evaluation, MAR is considered to be feasible for the study site. Input data used to conduct the technical feasibility assessment (and the other parts of the holistic analysis) are described in Table S1 in the Supplement.
2.5 Part 2: financial cost–benefit analysis (CBA)
A CBA is a decisional procedure that compares the costs and benefits of a project in monetary terms and uses these quantities to evaluate the project's effects on the well-being of people (Campos et al., 2018; Clinch, 2004; Hanley, 2013). First, the CBA approach identifies costs and benefits of a project within the system boundaries. Second, it analyses them and assigns monetary values. Third, the costs and benefits are discounted over the lifetime of the project. Lastly, the CBA compares costs and benefits with each other (Hanley, 2013; Nautiyal and Goel, 2021). In this study, the procedures followed to perform the CBA are based on the guidelines given by the European Commission (2015) for CBA of investment projects. In a CBA, the net present value (NPV) is used to compare discounted costs and benefits:
A positive NPV indicates that the tested project, measure, or scenario is profitable; otherwise, “the CBA test” failed (Hanley, 2013). Equation (1) can be expressed as follows (Hanley, 2013):
where NPV is determined as the sum of yearly contributions (t= year 1, 2,…, N, where N is the project's lifetime in years) and B and C, respectively, represent the benefits and costs of a project. Finally, Eq. (2) can be rewritten for our purposes as follows to represent the results of the financial CBA:
DWS represents the benefits of the drinking water supply, C represents the costs, and r is the discount rate. In this work, the project's lifetime was set to 30 years (European Commission, 2015).
The costs of the analyzed sustainability measures were estimated using the analogy method and expert opinion method (Angelis and Stamelos, 2000). The RO investment costs were based on the published costs (GBUC Public Relations, 2021). Project manager costs (Table S1) were based on expert-based knowledge given by the company Phoenix Engineering (Michelle Gomez, personal communication, 14 April 2022), while the required hours for each task for the MAR and the reforestation measures were based on Soža and Patekar (2022). In the financial CBA, the reference scenario represents a status without any measures applied, for which we compared the effects of the currently applied RO measure and of the potential sustainability measures.
2.6 Part 3: extended cost–benefit analysis (CBA)
An extended (or social or environmental) CBA includes environmental and other economically relevant impacts in the analysis of a project, implying the valuation of goods and services not exchanged in markets; this is done using non-market valuation methods (Brouwer and Sheremet, 2017; Clinch, 2004; Hanley, 2013; Martínez-Paz et al., 2014). This approach is more appropriate for evaluating government interventions than a financial CBA. Extended CBAs have already been applied in the past (Acuña et al., 2013; Cerulus, 2014; Grossmann, 2012; Logar et al., 2019; Ruangpan et al., 2020), but application examples are still lacking in the field of MAR. In this work, we present an extended CBA that includes five ecosystem service (ES) types to evaluate the introduced sustainability measures (cf. Sect. 2.2) in a holistic way: (i) drinking water supply and (ii) tourism were included in the extended CBA of RO and MAR scenarios, while (iii) carbon sequestration, (iv) habitat provisioning, and (v) timber provisioning were included in the extended CBA of the reforestation measure. The ES of tourism is a cultural ecosystem service that includes both benefits to visitors and income opportunities for nature tourism service providers (FAO, 2023), as also recognized by the Millennium Ecosystem Assessment (MEA, 2005). The InVEST (NCP, 2024) models Carbon Storage and Sequestration and Managed Timber Production were applied to estimate the biophysical and monetary values of carbon sequestration and timber provisioning, respectively.
The Carbon Storage and Sequestration model is based on the Tier 1 method of IPCC reports (IPCC, 2006, 2014). Biophysical carbon sequestration in plant roots and respective carbon storage in a specific region are estimated by aggregating carbon pool values assigned for each land use land cover (LULC) type (NCP, 2024). For Grand Bahama, the land cover map was reclassified based on the ecofloristic zones defined by the Food and Agriculture Organization (Ruesch and Gibbs, 2008) to differentiate the carbon pools for each zone, leading to 18 carbon classes. The value of carbon sequestration was estimated by multiplying the social cost of carbon (SCC) by the total sequestered carbon. Three different carbon prices were used to address uncertainties in the SCC (Table S1).
The Managed Timber Production model requires harvest information, including harvest frequency, harvested biomass, and market value of harvested products. As no field data were available for Grand Bahama, the input data were based on previously published literature (Table S1). As the harvesting frequency of pine trees is usually 30 years, only one harvesting revenue was considered.
It was assumed that the implementation of the sustainability measure, i.e., MAR, would provide potable water for about 30 % of the population with a connection to the public water supply (4127 of 13 755 houses connected to public piping), equal to the access to potable water after Hurricane Dorian (Department of Statistics of The Bahamas, 2012).
To estimate the ES of habitat provisioning, we used the willingness-to-pay (WTP) benefit-transfer method to conserve habitat quality, obtained from Wang et al. (2021) (Table S1). The revenue was calculated by multiplying the WTP by the total number of households in Grand Bahama (Department of Statistics of The Bahamas, 2012). To estimate the value of the tourism ES, we assume that restoring the drinking water supply could increase tourism on Grand Bahama. In fact, tourism facilities (e.g., hotels and restaurants) were also affected by the lack of water supply after Hurricane Dorian, not allowing them to conduct business at full capacity. In the following, we take into account that tourism expenditure would return to the same status as before a hurricane event. Moreover, the tourism sector is affected by a whole range of impacts, where it is complicated to attribute the contribution of the analyzed measures. Accordingly, we estimated the tourism ES of a sustainable measure as 1 % of tourism additional revenue (Soža and Patekar, 2022) based on data provided by Bahamas Ministry of Tourism (2022). Therefore, the ES of tourism, T, can be given as follows:
The description of the method applied to estimate annual average tourism expenditure data can be found in Sect. S2 in the Supplement. Finally, the NPV of the extended CBA, covering all considered ecosystem services, is estimated by the following equation (modification of Eq. 2):
where carbon is the ES of carbon sequestration, TP is the ES of timber provisioning, HP is the ES of habitat provisioning, and T is the ES of tourism (for other definitions, cf. Eq. 2). As for the financial CBA, in the extended CBA, the reference scenario represents a status without any measures applied, allowing for the comparison of the effects of the currently applied RO measure and of the potential sustainability measures. In summary, please refer to Table 1 for a schematic representation of the factors included in the different methodology steps.
3.1 Part 1: technical feasibility of managed aquifer recharge
Following the six identified steps for the technical feasibility assessment (Fig. 2), actual water demand corresponds to 11 356 m3 d−1 (3 mgd), i.e., the abstraction from wellfield 6 that currently covers water supply on the island of Grand Bahama (currently brackish groundwater). In a preliminary assessment, the recovery time of the aquifers by rainfall recharge was predicted to be 20 years (Al Baghdadi, 2021). A detailed groundwater model to predict the recharge and groundwater flow needed to mitigate the saltwater intrusion of the brackish aquifer (by dilution) could not be prepared because of limited data for the study site. A requirement is also to identify aquifers with adequate hydrogeological properties for storing and transmitting sufficient volumes of water. The entire island consists of karstified carbonates, and the latest available measurements document a porosity of 15 %–25 % and hydraulic conductivities of up to 2100 m d−1, with strong variations due to the heterogeneity of the aquifers (Whitaker and Smart, 1997a, 2000). Due to the lack of detailed investigations of the karst system (e.g., caves or conduits, porous rock facies) on the island, we assumed that, generally, the aquifers of the island could be suitable for MAR.
Rainwater was evaluated to be the most likely water source for a MAR scheme since surface water is not available on the island. Additionally, a major part of wastewater is treated locally in pit latrines and already recharges the aquifer. The analysis of rainfall data available from 2012 to 2022 revealed substantial precipitation amounts of 1594 mm yr−1 on average. Based on the limited water source and the aquifers available on the island, rainwater harvesting was identified as the most suitable MAR type. The harvesting of rainwater in the wellfields 1, 3, and 4 could be performed with rooftop rainwater harvesting and infiltration on site into the aquifer via drain trenches installed locally on the properties. An evaluation of the proposed rainwater harvesting scheme with a drain trench was conducted in a risk assessment. Hazards were identified, and a qualitative risk analysis and evaluation were conducted. The major human health risks identified were infiltration of saltwater or water with high pollutant load during storm events into the drain trenches. Further, bird feces from rooftops can infiltrate them as well, causing a microbiological contamination of the water. From a technical perspective, groundwater flooding due to an elevated groundwater table was identified as the major risk (detailed results of the risk assessment can be found in Sect. S3).
Based on prior results (including risk assessment mitigation measures; Sect. S3), the following criteria for the selection of the most suitable MAR location were defined that also allow for risk mitigation: (i) a minimum distance of the drain trench to the groundwater table to ensure sufficient natural treatment of infiltrating water (purification within the unsaturated zone) and avoid groundwater flooding; (ii) a sufficiently high elevation against high storm surges; and (iii) the use of rooftops for rainwater harvesting, where the location of MAR should be within a populated area. Furthermore, (iv), the rainwater harvesting schemes should be located at suitable areas that allow for effective groundwater recharge. Suited areas with respect to the groundwater level (depth to the groundwater table) were mapped with an MCDA-GIS approach for wellfields 1, 3, and 4 (Fig. 4).
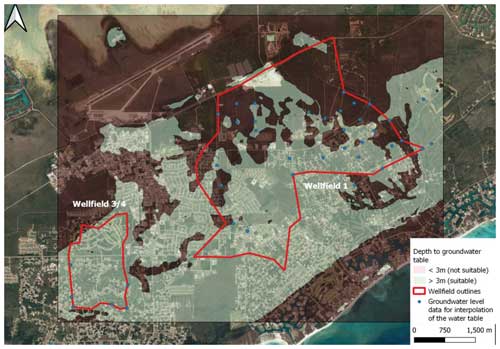
Figure 4MAR suitability map with respect to the depth to the groundwater table for the populated areas of wellfields 1, 3, and 4 (source of base map: © Google Maps).
Available recharge volume from rooftop rainwater harvesting (RRWH) was estimated according to DIN (2002) based on the roof area of the 2456 buildings and average rainfall volumes for potential infiltration in wellfield 1, wellfield 3, and 4, and all three wellfields together (Table 2). To obtain the surplus of recharge from the MAR rainwater harvesting scheme with a drain trench, the current natural estimated recharge of 25 % (Little et al., 1977; Whitaker and Smart, 1997a) was deducted from the estimated recharge with a MAR scheme.
Table 2Roof area, recharge volume from rainwater harvesting, and resulting surplus recharge for the study areas.
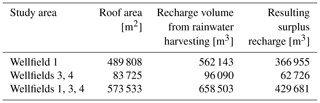
A total of 429 681 m3 yr−1 of additional recharge could be achieved with RRWH in wellfields 1, 3 and 4, corresponding to 10.4 % of the water demand for replacing the supply by brackish water. Therefore, the MAR scheme is not able to fully supply the water demand on Grand Bahama but rather contributes to a sustainable groundwater management practice. Unless an investigation is conducted to identify groundwater flow paths, a reliable prediction of enhanced groundwater recharge originating from MAR rooftop rainwater harvesting schemes with drain trenches outside of wellfields 1, 3 and 4 is not reliable. From a technical perspective, the implementation of the RRWH in the 2456 buildings in the wellfields would be possible. However, the construction of 2456 RRWH schemes would be a time-consuming task, and public acceptance would be a prerequisite to install these schemes on private terrain. Additionally, the question of who would take over the costs for the RRWH schemes would need to be discussed as part of the CBA.
3.2 Part 2: financial CBA
3.2.1 Identification of the reforestation scenario
Results of the financial CBA and the extended CBA are presented in Tables A1–A3 (Appendix A). Based on experts' opinion from the Bahamas Forestry Unit (Ingeria Miller, personal communication, 23 February 2022), the reforestation scenario takes three areas into account (Fig. 5): the first area (56.04 km2) is located in wellfield 6, where all mature pine trees were destroyed during Hurricane Dorian (Welsh et al., 2022); the second (70.30 km2) and third areas (53.63 km2) occupy public land in the east GB forest reserve, where Hurricane Dorian also affected the pine trees.
Based on the amount of fallen timber, one option for recovering costs could be for the land managers to obtain a permit to harvest. In addition, it was assumed that reforestation considers sustainable harvesting that does not affect future timber yields.
3.2.2 Net present value according to the financial CBA
The financial CBA included the supply of drinking water as a monetary benefit. The results of the estimated investment costs, operation costs, and revenues were discounted over the 30-year period from 2020 to 2050 (Table 3). The planned water capacity of the reverse osmosis (RO) system is 11 356 m3 d−1 (GBUC, 2021), and that of the RRWH measure is 1177 m3 d−1 (Table A1). With regards to RO, the investment costs are provided as a lump sum. The operation costs of RO include variable and fixed operation and maintenance costs (O&M) and annual repair and replacement (R&R) fund (see Sect. S4 in the Supplement for a detailed description of the cost estimation). The RO measure results for the financial CBA in a positive NPV of USD 51 131 907 over a 30-year period with a discount rate of 4 %.
The total investment cost of RRWH systems is estimated at USD 19.58 million based on detailed project management and administration, preparation of project, implementation of works and equipping, and promotion and viability costs (a detailed description of the cost estimation is provided in Sect. S5 in the Supplement). The implementation of the system in all 2456 buildings of wellfields 1, 3, and 4 would require at least 2 years, leading to a longer time for investment costs. As a result of the financial CBA for the RRWH measure, the NPV for a 30-year period at a discount rate of 4 % is negative and equal to USD −15 638 010.
The total investment costs of the reforestation scenario are estimated at USD 103.89 million based on detailed project management and administration, preparation of project, implementation of works and equipping, and promotion and viability costs (detailed description of the cost estimation is provided in Sect. S6 in the Supplement). As mentioned in the methodology, the reforestation measure in the financial analysis sees no estimations in terms of water supply, leading to zero revenues. As a result of the financial CBA for the reforestation measure, the NPV for a 30-year period at a discount rate of 4 % is negative and equal to USD −135 690 081.
Table 3 reports the results of the financial CBA in terms of NPV for the comparison of RO, MAR (RRWH), and reforestation measures for a set of 10 discount rates from 1 % to 10 % as a sensitivity analysis, although the value of 10 % goes beyond recommended discount rate values. When only drinking water supply is considered, RO is the best-performing measure, with a positive NPV, increasing as the discount rate values get lower. The second-best-performing measure according to the financial CBA is the RRWH system, with negative NPV values, which increase as the discount rate value decreases. The worst-performing measure in terms of water provisioning is reforestation, with negative NPV values, which increase proportionally to the discount rate.
3.3 Part 3: extended CBA
The extended CBA took into account as benefits not only the supply of drinking water, but also other ESs (Tables A1–A3). The extended CBA for RO considered as benefits the drinking water supply and tourism because these ESs are based on water capacity improvement. The RO measures results for the extended CBA in a positive NPV of USD 67 748 586 over a 30-year period with a discount rate of 4 %.
Similarly, to RO, the drinking water supply and tourism benefits were included as revenues for the potential MAR project, leading, however, to a negative NPV of USD −13 194 905 over a 30-year period with a discount rate of 4 %. Instead, for reforestation, carbon sequestration, habitat quality, and timber production benefits were included as revenues for the project, leading to a positive NPV of USD 71 879 831 for the 30-year period at a discount rate of 4 %.
Table 4 represents the results of the extended CBA for the tested measures for multiple discount rates. When additional ESs are considered, RO shows always positive NPV values, RRWH still shows negative NPV values, and the reforestation measure leads to mixed results in terms of profitability. Moreover, in comparison to a financial CBA, RO is not the best-performing measure for all discount rate values anymore: for discount rate r<4%, reforestation shows a higher NPV than RO; for , reforestation shows a lower NPV than RO but still a positive one; and for r>7%, the reforestation measure is not profitable.
It can be observed that, in all analyses, the discount rate has a big impact on the results. For five out of six measures, the lower the discount rate, the higher the NPV. This is explained by the fact that lower discount rates remunerate future benefits more than high discount rates (Martínez-Paz et al., 2014). Consequently, with low discount rates, we see that environmental measures are more profitable because these see long-term benefits. For the same principle, with high discount rates, the later the costs of a measure required to be settled, the more profitable that measure will be. However, for the case of the reforestation measure in the financial CBA (Table 3), the NPV is declining with the discount rate since only costs are considered. Researchers suggested different ways to deal with the uncertainty related to the discount rate: from using low discount rates for environmental projects (Costanza et al., 2017) to using multiple values according to the time or service (Hanley, 2013; Martínez-Paz et al., 2014).
Table 3Net present value (NPV) of the financial CBA for the sustainability measures reverse osmosis (RO), rooftop rainwater harvesting (RRWH), and reforestation. The project lifetime is 30 years.
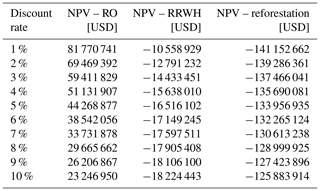
3.4 Evaluation of the methodological aspects
3.4.1 Technical feasibility of MAR
Technical feasibility studies for MAR measures are numerous and often apply common selection criteria or workflows (Sallwey et al., 2019). However, selection criteria must be adjusted based on regional or local (hydro)geology. Hejazian et al. (2017) investigated MAR implementation on an atoll in Marshall Islands but did not include selection criteria used for their evaluation. Apart from the Marshall Islands study, no methodologies were available for MAR feasibility assessments on islands with freshwater lenses (FWLs). Hence, we needed to develop a new methodology, including selection criteria. As a result, the methodology was only applied for our study case and has not been successfully applied to the small island setting with freshwater lenses.
Moreover, the methodology applied on Grand Bahama had to be tailored to an investigation with scarce data availability. Similarly, Dobhal et al. (2019) suggested a methodology for riverbank filtration with lower data availability in India. In their publication, selection criteria were not manifested with quantitative measures but rather with qualitative definitions. Further research could improve availability of hydrogeological data, and the MAR potential could be explored with a methodology following a quantitative approach.
3.4.2 Financial and extended CBA
The presented results with regards to the profitability of the measures depend on the methodological approach used for their estimation as the most appropriate methodologies to assess monetary values of ecosystem services and whether to include these estimates in a CBA are still not agreed upon. The CBA method has been widely applied in ecological restoration (Cerulus, 2014; Feuillette et al., 2016; Grossmann, 2012; Logar et al., 2019) and in water system assessments (Acuña et al., 2013; Ghafourian et al., 2021; Ruangpan et al., 2020). However, this approach shows some limitations related to the lack of a method to estimate some benefits (Ruangpan et al., 2020), the estimation of the costs, the overuse of qualitative data, and the lack of validation of the results (Network Nature, 2022; Sudmeier-Rieux et al., 2021; Wegner and Pascual, 2011).
To take uncertainty into account in our analyses, we first applied multiple discount rates. In fact, based on past research, the discount rate is amongst the most sensitive parameters and is hence an important source of uncertainty (Costanza and Daly, 1992; Perosa, 2023). A low discount rate reduces the devaluation of future effects, favoring policies with long-term benefits and low present costs, while a high discount rate does the opposite (Dominati et al., 2014; Hanley, 2013). Thus, a low rate values long-term benefits more, whereas a high rate emphasizes short-term benefits (Dominati et al., 2014). This approach allowed us to understand the effects of one of the most relevant uncertainty sources on the results. However, other sources of uncertainty can be found in our ES assessment and evaluation. As for the reproduction of natural phenomena through modeling, the methods applied for the ecosystem services estimations are affected by uncertainties, e.g., with regards to the input data used (usually not location-specific) or concerning the parameterization of the models. First, uncertainty is inherent in all techniques used for ES estimations (Dominati et al., 2014). Costanza et al. (2017) note that imperfect information affects the evaluation of ES, beginning at the process understanding level and extending through the quantification and economic valuation of ES (Dominati et al., 2014). This imperfection stems from limited biophysical and economic data availability (Dominati et al., 2014) or from relying on simplistic assumptions or expert opinion, such as the relationship between land cover, water provision, and land use (Vollmer et al., 2022). Also, the way this imperfection is included in the ES estimations depends on which models and software are chosen. Due to time resources, our analysis used one main software (InVEST) to guide the ES estimations, but others could be used. For example, a promising alternative model is the ARtificial Intelligence for Environment and Sustainability (ARIES) (Villa et al., 2014). Another recommendation would be to consider at least two benefits per ecosystem function (Boithias et al., 2016), which is a biophysical relationship that exists regardless of whether or not humans benefit from an ecosystem (Costanza et al., 2017). For example, the ecosystem function of water storage and retention can contribute to the service (benefit) of the drinking water supply. A further approach after Boithias et al. (2016) would be to use multiple valuation metrics to value each benefit, e.g., applying willingness to pay and carbon prices to evaluate the benefit of carbon sequestration.
Additionally, the lack of standards for ES modeling, assessment, and valuation along with the high time and resource demands of sophisticated methods pose some challenges (Costanza et al., 2017). For example, this can lead to double counting, where provisioning, regulating, or cultural services are counted alongside their supporting services (Costanza et al., 2017). Inappropriate classification often causes this issue (Fisher et al., 2008). In our analysis, we ensured that double counting does not happen using the MEA (2005) classification. Potential ways to address these sources of uncertainty in the future are to use the Monte Carlo approach or simpler methods such as assuming a spatially uniform error or using alternative raster inputs (Hamel and Bryant, 2017; Vining and Weimer, 2010). Additionally, as mentioned above, using multiple models to simulate the same process could help assess the effects of conceptual model uncertainty (Hamel and Bryant, 2017). For example, Wegner and Pascual (2011) suggest a pluralist framework of CBA composed of a heterogeneous set of value-articulating instruments, appropriate to the context within a specific decision. Saarikoski et al. (2016) state that multi-criteria decision analysis (MCDA) performs better than a CBA because it allows for including non-monetary ecosystem services. However, Perosa et al. (2022) showed the still existing limitations for the actual application of MCDA for river basin management. A combination of CBA and MCDA methods could be a potential solution (Saarikoski et al., 2016), although it implies higher efforts.
Besides methodological aspects related to the CBA itself, the absence of some ecosystem services in the extended CBA also represents a limitation as it implies that the value of these ecosystem services has been set to zero. A first example of omitted benefits are cultural services. InVEST provides a model (Visitation: Recreation and Tourism) to estimate the effects of land use changes to nature-based recreation and tourism. The model uses the quantity and location of photos uploaded to Flickr to understand how landscape characteristics correlate to recreation and tourism. Within the framework of this publication, this InVEST model was tested but could not provide statistically significant results. A potential alternative to estimate nature-based recreation benefits could be to use the Travel Cost Method, for which a valid approach is suggested under the Toolkit for Ecosystem Service Site-based Assessment (TESSA; Peh et al., 2017). The method involves collecting data through interviews, which can be conducted in person or online, as done by Perosa et al. (2021) through social media. Another example of ecosystem services (ESs) not included in our study is crop pollination. InVEST provides a model for this ES as well, designed to model characteristics of nesting and foraging habitats of wild bees. As other factors influence pollination on Grand Bahama, the model was not applicable for this study area. Further research may use other models that include different animal pollinators or other weather drivers (e.g., wind) as another pollination factor.
Besides missing ecosystem services, other aspects are still missing from the CBAs of the analyzed measures. First, a potential additional benefit of MAR is that its implementation would decrease the water volume filtered by the RO system, with consequent energy savings. Second, a potential negative effect of the reforestation measures is that these measures could, most likely, decrease water recharge at the local level, which could affect groundwater lenses.
3.5 Services and costs generated by MAR compared to RO
For the investigated MAR schemes, only about 10.4 % of the water demand could be supplied, whereas RO could supply 100 % of the water demand. The financial and ES assessments showed that the MAR scheme would also be less profitable from a financial and an ES-based extended CBA.
A major difference between MAR and RO lies in the investment costs (USD 5 million for RO compared to USD 22 million for MAR). The costs of the RO, which desalinates the groundwater but does not restore the aquifer, are lower than the costs of the MAR measure, which acts as an ecosystem restoration. However, this difference is not represented by the ecosystem service of water supply, which does not distinguish between water supplied from the RO plant and from the aquifer. An important improvement of this analysis would require finding a way to estimate the ecosystem service of aquifer recharge in addition to the ecosystem service of water supply, although this could potentially add more uncertainty to the results.
3.6 Reforestation
The results of the financial and extended CBA for the reforestation measure indicated profitable results. The reforestation of pine forests would increase 10 % of local stored carbon compared to current land use, and carbon sequestration would generate USD 271 million along the analysis period of 30 years. Still, as discussed in Sect. 3.4, the results are subject to limitations related to data shortage.
Nevertheless, additional benefits could be generated by the reforestation planned in wellfield 6 (Fig. 4). Positive impacts on groundwater quantity and quality of forests were identified in that area; hence, the reforestation measure could be implemented as a groundwater management strategy (Ellison, 2018). A known positive effect of pine forests is the potential of phytoremediation, where salt is taken up by the plant and removed from the groundwater. However, vegetation might also cause the decrease of freshwater lenses (FWLs). Hejazian et al. (2017) studied an atoll in the Marshall Islands that consists of two lobes of land underlain by FWLs. One lobe was cleared from tropical forest due to military use, and, consequently, the FWL grew significantly in thickness due to a reduction of evapotranspiration. We recommend studying the effect of the forest on FWLs on Grand Bahama as well. Furthermore, a potential benefit of reforestation is the increase of nature-based recreation caused by increased biodiversity, among others, through birdwatching, one of the most popular tourist attractions on Grand Bahama.
3.7 Suggestions for sustainable groundwater management on Grand Bahama
Even after the implementation of the RO scheme, the population indicates insufficient desalinated water from wellfield 6 to their households or even water outages (personal communication with population). The Grand Bahama Utility Company explains these shortcomings with problems pumping water from wellfield 6 in sufficient quantity, likely because of lacks in the supply system. In comparison to the RO system, utilizing the MAR scheme of RRWH in wellfields 1, 3, and 4 would likely not receive an additional water load and would not strain the water supply system. The existing water supply infrastructure would be able to convey 10.4 % more water to the households. Therefore, the implementation of RRWH schemes should be considered an additional option to provide a reliable water supply on the island, potentially also combining it with RO. Investigations of MAR feasibility should be reassessed after the collection of (hydro)geological information outside the wellfields. Adverse effects of the RO scheme, such as high energy consumption or brine waste, are further negative points of its application. Nevertheless, the implementation of the RRWH scheme relates to a long construction time and would require public acceptance for building such schemes on private premises.
Further measures, such as the reduction of water use or the reduction of leakage losses, which currently account for 30 %–40 % of the water demand, could be researched (CDM, 2011). It is crucial that the public is involved in the decision-making for groundwater ecosystem restoration measures to gain acceptance for their implementation (UNEP, 2021). Relying on the RO scheme as the only contingency plan for a safe water supply on GB may be shortsighted.
The Bahamas suffers from the consequences of recurring hurricanes. To mitigate these effects and restore the natural ecosystems, multiple measures have been discussed among stakeholders on Grand Bahama. Two planned sustainability measures, MAR and reforestation, were investigated for the mitigation of impacts of Hurricane Dorian on the island of Grand Bahama. A holistic analysis of the two measures was conducted: an economic assessment was performed with a financial CBA, and the ecosystem services of the measures were investigated with an extended CBA. The existing RO scheme on the island was also assessed with the financial and extended CBA, and results were compared to the planned MAR measure for the drinking water supply.
The proposed MAR scheme of rooftop rainwater harvesting with a drain trench from buildings in wellfield 1, 3, and 4 on Grand Bahama was technically evaluated and judged to be feasible. Nevertheless, the financial CBA evaluated the MAR scheme to be less profitable compared to the RO measure, which is explained by the difference in investment costs (USD 22 million compared to USD 5 million for RO). Both the financial and the extended cost–benefit analysis methods do not distinguish between the two different ways in which RO and MAR supply freshwater but account for a comparable ecosystem service: the freshwater supply. This leads to disregard the additional value of the MAR scheme of regenerating the groundwater ecosystem in comparison to a mere water supply provided by the RO system. We suggest that researchers investigate this aspect of MAR's benefits in the future. Areas for reforestation efforts were identified. The reforestation measure was assessed to be financially profitable and showed extensive potential to sustain the forest ecosystem services on the island.
The main limitation for the technical feasibility assessment of MAR on the island was a lack of hydrogeological data. We suggest further (hydro)geological data collection outside of the wellfields and to reevaluate the MAR potential based on such newly collected information. The financial CBA and extended CBA been criticized in the past with regards to how costs are estimated, how benefits are modeled and monetized, and how results are validated. Finally, results obtained within this study are subject to uncertainty due to the lack of detailed input data for the models and the assessments. The implementation of the sustainability measures on Grand Bahama is likely judged on the reforestation schemes.
The results of this work show that substantial financial and labor efforts are necessary to restore the forest and groundwater ecosystem on the island. Furthermore, this study supports that only a technical, economic, or ecological assessment of a planned human intervention in an environmental system falls short of accurately estimating its feasibility and benefit for the study area and its population. Therefore, a holistic approach considering different aspects should be pursued. The lack of data for MAR feasibility evaluation and extended CBA (financial assessment of nature-based solutions) affected the obtained results and related uncertainty. Methods for technical, economic, and ecosystem service assessments should be developed further in the future to help decision-makers in reaching the Sustainable Development Goals set by their governments.
Table A1Costs and revenues for the financial and extended cost–benefit analysis (CBA) of the RO measure along the 30-year analysis period, where the extended CBA is represented in the “Extended revenues” section of the table. O&M: operation and maintenance. R&R: repair and replacement. Benefits related to the extended cost–benefit analysis are shown in the lower part of the table (extended CBA).
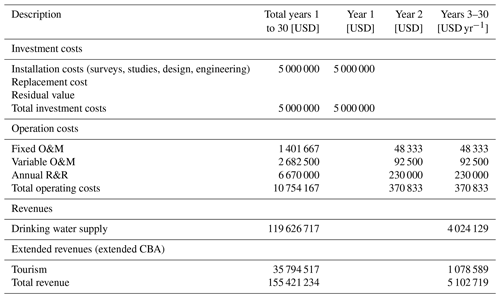
Table A2Costs and revenues for the financial and extended cost–benefit analysis (CBA) of the MAR measure along the 30-year analysis period, where the extended CBA is represented in the “Extended revenues” section of the table. Benefits related to the extended cost–benefit analysis are shown in the lower part of the table (extended CBA).
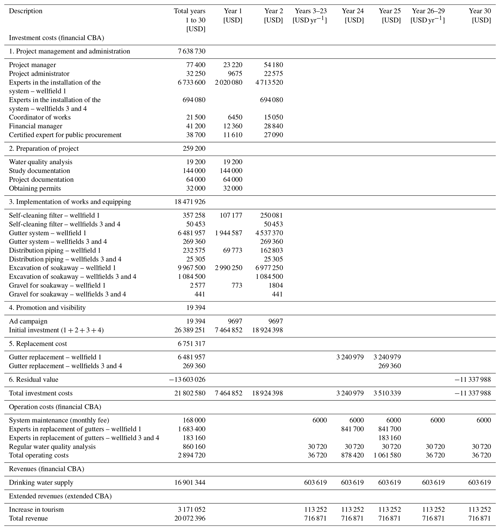
Table A3Costs and revenues for the financial and extended cost–benefit analysis (CBA) of the reforestation measure along the 30-year analysis period, where the extended CBA is represented in the “Extended revenues” section of the table. Benefits related to the extended cost–benefit analysis are shown in the lower part of the table (extended CBA).
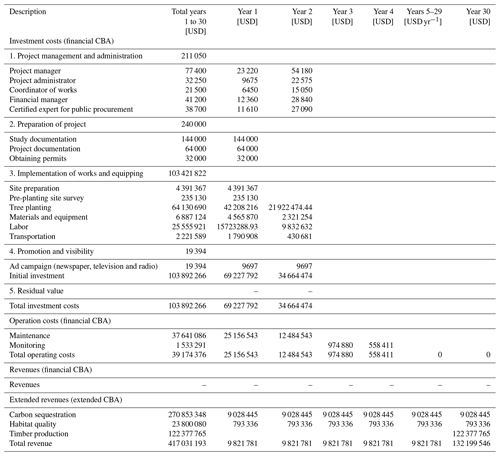
The resulting data set and procedures used to create the data set of this study are available on the mediaTUM data repository (institutional repository of the Technical University of Munich) under the DOI: https://doi.org/10.14459/2024mp1755541 (Imig et al., 2024).
The supplement related to this article is available online at: https://doi.org/10.5194/hess-28-5459-2024-supplement.
Conceptualization: AI, FP, and AR; methodology, validation, formal analysis, investigation, and writing (review and editing): all authors; software: FP, CIH, and SK; data curation: CIH and SK; writing (original draft preparation): AI and FP; visualization: AI, FP, SK, and CIH; supervision, project administration, and funding acquisition: AR, KW, FP, YZ, and AI. All authors have read and agreed to the published version of the paper
The contact author has declared that none of the authors has any competing interests.
Publisher's note: Copernicus Publications remains neutral with regard to jurisdictional claims made in the text, published maps, institutional affiliations, or any other geographical representation in this paper. While Copernicus Publications makes every effort to include appropriate place names, the final responsibility lies with the authors.
We acknowledge the support from the Grand Bahama Utility Company as well as IsraAID for this research in helping to understand the urban water supply system in The Bahamas. Funding is acknowledged as detailed in the section Financial Support.
This research was partially funded through the National Natural Science Foundation (grant no. 42321004), the Guangdong Provincial Key Laboratory of Soil and Groundwater Pollution Control (grant no. 2023B1212060002), the High-level University Special Fund (grant no. G030290001), the Bahamian Protected Areas Fund (BPAF) project SOFTGR, the German Academic Exchange Service (DAAD) PROMOS fund, and the BayIntAn fund from the Bavarian Research Alliance
This paper was edited by Yongping Wei and reviewed by four anonymous referees.
Acuña, V., Díez, J. R., Flores, L., Meleason, M., and Elosegi, A.: Does it make economic sense to restore rivers for their ecosystem services?, J. Appl. Ecol., 50, 988–997, https://doi.org/10.1111/1365-2664.12107, 2013.
Angelis, L. and Stamelos, I.: A Simulation Tool for Efficient Analogy Based Cost Estimation, Empir. Softw. Eng., 5, 35–68, https://doi.org/10.1023/A:1009897800559, 2000.
Ault, T.: Water resources: Island water stress, Nat. Clim. Chang., 6, 1062–1063, https://doi.org/10.1038/nclimate3171, 2016.
Al Baghdadi, L.: Studying the Storm-induced Salinization of the Grand Bahama Island Aquifer due to Hurricane Dorian, University of California Sacramento, 2021.
Bahamas Ministry of Tourism: Expenditure: Yearly Expenditure Comparisons By Qtr &Visitor Type, https://www.tourismtoday.com/statistics/expenditure (last access: 13 December 2024), 2022.
Bahamas National Trust: State of the environment: post hurricane Dorian report, https://bnt.bs/postdorianreport/ (last access: 13 December 2024), 2020.
Bedekar, V. S., Memari, S. S., and Clement, T. P.: Investigation of transient freshwater storage in island aquifers, J. Contam. Hydrol., 221, 98–107, https://doi.org/10.1016/j.jconhyd.2019.02.004, 2019.
Boithias, L., Terrado, M., Corominas, L., Ziv, G., Kumar, V., Marqués, M., Schuhmacher, M., and Acuña, V.: Analysis of the uncertainty in the monetary valuation of ecosystem services – A case study at the river basin scale, Sci. Total Environ., 543, 683–690, https://doi.org/10.1016/j.scitotenv.2015.11.066, 2016.
Bowen-O'Connor, C. and Lynch, E. M.: Discovering third space in citizen science and resource recovery efforts post-hurricane Dorian, American Anthropological Association Annual Meeting, 2022.
Brouwer, R. and Sheremet, O.: The economic value of river restoration, Water Resour. Econ., 17, 1–8, https://doi.org/10.1016/j.wre.2017.02.005, 2017.
Campos, I., Ng, K., Penha-Lopes, G., Pedersen, A. B., Capriolo, A., Olazabal, M., Meyer, V., Gebhardt, O., Weiland, S., Nielsen, H. Ø., Troeltzsch, J., Zandvoort, M., Lorencová, E. K., Harmáčkova, Z. V, Iglesias, P., Iglesias, A., Vizinho, A., Mäenpää, M., Rytkönen, A.-M., den Uyl, R. M., Vačkář, D., and Alves, F. M.: The Diversity of Adaptation in a Multilevel Governance Setting, in: Adapting to Climate Change in Europe, Elsevier, 49–172, https://doi.org/10.1016/B978-0-12-849887-3.00003-4, 2018.
Caribbean Environmental Health Institute (CEHI), Antigua Public Utilities Authority (APUA), The United Nations Environment Programme (UNEP), Caribbean Environmental Health Institute (CEHI), and GWP Consultants LLP: Managed Aquifer Recharge (MAR): Practical Techniques for the Caribbean, 68 pp., 2010.
CDM Camp Dresser & McKee Inc.: Groundwater Supply, Sustainability Yield and Storm Surge Vulnerability, CDM Camp Dresser & McKee Inc, 2011.
Cerulus, T.: Reflection on the Relevance and Use of Ecosystem Sevices to the LNE Department, in: Ecosystem Services: Global Issues, Local Practices, edited by: Jacobs, S., Dendoncker, N., and Keune, H., Elsevier, ISBN 9780124199644, 2014.
Clinch, J. P.: Cost–Benefit Analysis Applied to Energy, in: Encyclopedia of Energy, Elsevier, 715–725, https://doi.org/10.1016/B0-12-176480-X/00237-0, 2004a.
Cohen-Shacham, E., Walters, G., Janzen, C., and Maginnis, S.: Nature-based solutions to address global societal challenges, IUCN, Gland, Switzerland, 97 pp., https://doi.org/10.2305/IUCN.CH.2016.13.en, 2016.
Costanza, R., de Groot, R., Braat, L., Kubiszewski, I., Fioramonti, L., Sutton, P., Farber, S., and Grasso, M.: Twenty years of ecosystem services: How far have we come and how far do we still need to go?, Ecosyst. Serv., 28, 1–16, https://doi.org/10.1016/j.ecoser.2017.09.008, 2017.
Costanza, R. and Daly, H. E.: Natural Capital and Sustainable Development, Conserv. Biol., 6, 37–46, https://doi.org/10.1046/j.1523-1739.1992.610037.x, 1992.
DEEPWATER-CE: Collection of good practice and benchmark analysis on MAR solutioins in the EU – D.T1.2.1, https://www.interreg-central.eu/Content.Node/DEEPWATER-CE.html (last access: 28 November 2024), 2020a.
DEEPWATER-CE:Transnational desicion support toolbox for designating potential MAR location in Central Europe – D.T2.4.3, https://www.interreg-central.eu/Content.Node/DEEPWATER-CE.html (last access: 28 November 2024), 2020b.
Department of Statistics: The Commonwealth of The Bahamas: Cenus of Population and Housing 2010, edited by: Mackey, C., Gibson, N., Winters, C., Wilson, L., Saunders, K., Rolle, K., Conyers, C., Storr, L., and Rolle, T., 197 pp., https://www.bahamas.gov.bs/wps/wcm/connect/c0d9fae8-54df-49e3-b4b9-92e29e0b264c/2022+CENSUS+PRELIMINARY+RESULTS_FINAL+April+12+2023.pdf?MOD=AJPERES#:~:text=The Bahamas National Statistical Institute is pleased to release the,192,544 males and 206,770 females (last access: 28 November 2024), 2012.
Diamond, M. G. and Melesse, A. M.: Water Resources Assessment and Geographic Information System (GIS)-Based Stormwater Runoff Estimates for Artificial Recharge of Freshwater Aquifers in New Providence, Bahamas, in: Landscape Dynamics, Soils and Hydrological Processes in Varied Climates, ISBN 9783319187860, Springer, 1st ed. 2016 edition (August 5, 2015), 411–434, 2016.
Dillon, P.: Future management of aquifer recharge, Hydrogeol. J., 13, 313–316, https://doi.org/10.1007/s10040-004-0413-6, 2005.
Dillon, P., Stuyfzand, P., Grischek, T., Lluria, M., Pyne, R. D. G., Jain, R. C., Bear, J., Schwarz, J., Wang, W., Fernandez, E., Stefan, C., Pettenati, M., van der Gun, J., Sprenger, C., Massmann, G., Scanlon, B. R., Xanke, J., Jokela, P., Zheng, Y., Rossetto, R., Shamrukh, M., Pavelic, P., Murray, E., Ross, A., Bonilla Valverde, J. P., Palma Nava, A., Ansems, N., Posavec, K., Ha, K., Martin, R., and Sapiano, M.: Sixty years of global progress in managed aquifer recharge, Hydrogeol. J., 27, 1–30, https://doi.org/10.1007/s10040-018-1841-z, 2019.
DIN Deutsche Institut für Normung e.V.: Rainwater harvesting systems Part 1: Planning, installation, operation and maintanance DIN 1989-1:2002-04, German Institute for Standardization or Deutsches Institut für Normung e.V, https://doi.org/10.31030/9240880, 2002.
Dobhal, R., Uniyal, D. P., Gosh, N. C., Grischek, T., and Sandhu, C.: Guidelines on Bank Filtration for Water Supply in India, India and M/s Bishen Singh Mahendra Pal Singh, 23-A, New Connaught Place, Dehra Dun, India, Uttarakhand State Council for Science & Technology (UCOST) Dehra Dun, ISBN 978-81-211-0993-2, 2019
Dominati, E. J., Robinson, D. A., Marchant, S. C., Bristow, K. L., and Mackay, A. D.: Natural Capital, Ecological Infrastructure, and Ecosystem Services in Agroecosystems, in: Encyclopedia of Agriculture and Food Systems, edited by: Van Alfen, N. K., Academic Press, Oxford, 245–264, https://doi.org/10.1016/B978-0-444-52512-3.00243-6, 2014.
Dos Anjos, N. D. F. R.: Source Book of Alternative Technologies for Freshwater Augmentation in Latin America and the Caribbean, Int. J. Water Resour. D., 14, 365–398, https://doi.org/10.1080/07900629849277, 1998.
Elliott, S. D., Blakesley, D., and Hardwick, K.: Restoring Tropical Forests: A Practical Guide, The Royal Botanic Gardens, ISBN 978-1-84246-442-7, 2013.
Ellison, D.: Background Analytical Study 2 Forests and Water Background study prepared for, United nations Forum on Forests, 50, https://www.un.org/esa/forests/wp-content/uploads/2018/04/UNFF13_BkgdStudy_ForestsWater.pdf (last access: 28 November 2024), 2018.
Emanuel, K.: Evidence that hurricanes are getting stronger, P. Natl. Acad. Sci. USA, 117, 13194–13195, https://doi.org/10.1073/pnas.2007742117, 2020.
European Commission: Guide to cost-benefit analysis of investment projects: Economic appraisal tool for cohesion policy 2014–2020, European Union, Luxembourg, https://doi.org/10.2776/97516, 2015.
FAO Food and Agriculture Organization of the United Nations: Ecosystem Services & Biodiversity (ESB) [WWW Document], https://www.fao.org/ecosystem-services-biodiversity/background/cultural-services/en/ (last access: 28 November 2024), 2023.
Feuillette, S., Levrel, H., Boeuf, B., Blanquart, S., Gorin, O., Monaco, G., Penisson, B., and Robichon, S.: The use of cost–benefit analysis in environmental policies: Some issues raised by the Water Framework Directive implementation in France, Environ. Sci. Policy, 57, 79–85, https://doi.org/10.1016/j.envsci.2015.12.002, 2016.
Fisher, B., Turner, K., Zylstra, M., Brouwer, R., de Groot, R., Farber, S., Ferraro, P., Green, R., Hadley, D., Harlow, J., Jefferiss, P., Kirkby, C., Morling, P., Mowatt, S., Naidoo, R., Paavola, J., Strassburg, B., Yu, D., and Balmford, A.: Ecosystem services and economic theory: Integration for policy-relevant research, Ecol. Appl., 18, 2050–2067, 2008.
Gale, I.: Strategies for Managed Aquifer Recharge (MAR) in semi-arid areas, UNESCO's Internationa Hydrological Programme (IHP), 1–33, United Nations Educational, Scientific and Cultural Organization (UNESCO), 2005.
Garfí, M., Cadena, E., Sanchez-Ramos, D., and Ferrer, I.: Life cycle assessment of drinking water: Comparing conventional water treatment, reverse osmosis and mineral water in glass and plastic bottles, J. Clean. Prod., 137, 997–1003, https://doi.org/10.1016/j.jclepro.2016.07.218, 2016.
GBUC: Grand Bahama Utility Company announces new capital investment amidst significant progress towards island-wide potability, https://grandbahamautility.com/news/press-releases/grand-bahama-utility-company-announces-new-capital-investment-amidst-significant-progress-towardsisland-wide-potability/ (last access: 21 December 2022), 2020.
GBUC: $5 million Reverse Osmosis System for Grand Bahama completed by GBUC: https://grandbahamautility.com/news/press-releases/grand-bahama-utility-company-announces-new-capital-investment-amidst-significant-progress-towardsisland-wide-potability/ (last access: 28 November 2024), 2021.
Ghafourian, M., Stanchev, P., Mousavi, A., and Katsou, E.: Economic assessment of nature-based solutions as enablers of circularity in water systems, Sci. Total Environ., 792, 148267, https://doi.org/10.1016/j.scitotenv.2021.148267, 2021.
Grossmann, M.: Economic value of the nutrient retention function of restored floodplain wetlands in the Elbe River basin, Ecol. Econ., 83, 108–117, https://doi.org/10.1016/j.ecolecon.2012.03.008, 2012.
Hamel, P. and Bryant, B. P.: Uncertainty assessment in ecosystem services analyses: Seven challenges and practical responses, Ecosyst. Serv., 24, 1–15, https://doi.org/10.1016/j.ecoser.2016.12.008, 2017.
Hanley, N.: Environmental Cost–Benefit Analysis, in: Encyclopedia of Energy, Natural Resource, and Environmental Economics, Elsevier, 17–24, https://doi.org/10.1016/B978-0-12-375067-9.00103-0, 2013.
Halytsia, O., Vrachioli, M., Janik, K., Sitek, S., Wojtal, G., Imig, A., Rein, A. and Sauer, J.: Assessing Economic Feasibility of Managed Aquifer Recharge Schemes: Evidence from Cost-benefit Analysis in Poland, Water Resour. Manag., 36, 5241–5258, https://doi.org/10.1007/s11269-022-03303-0, 2022.
Hejazian, M., Gurdak, J. J., Swarzenski, P., Odigie, K. O., and Storlazzi, C. D.: Land-use change and managed aquifer recharge effects on the hydrogeochemistry of two contrasting atoll island aquifers, Roi-Namur Island, Republic of the Marshall Islands, Appl. Geochem., 80, 58–71, https://doi.org/10.1016/j.apgeochem.2017.03.006, 2017.
ICF Consulting and BEST Bahamas Environmental Science and Technology Commission: Integrating Management of Watersheds and Coastal Areas in Small Island Developing States of the Caribbean: The Bahamas national report, 66 pp., ICF Consulting and BEST Bahamas Environmental Science and Technology Commission, 2001.
Imig, A., Szabó, Z., Halytsia, O., Vrachioli, M., Kleinert, V. and Rein, A: A review on risk assessment in managed aquifer recharge, Integr. Environ. Asses., 18, 1513–1529, https://doi.org/10.1002/ieam.4584, 2022.
Imig, A., Perosa, F., Hotta, C. I., Klausner, S., Welsh, K., and Rein, A.: Data related to the article: Technical assessment combined with extended cost-benefit analysis for the restoration of groundwater and forest ecosystem services – An application for Grand Bahama, https://doi.org/10.14459/2024mp1755541, 2024.
IPCC: IPCC Guidelines for National Greenhouse Gas Inventories: Volume 4: Agriculture, Forestry and Other Land Use, Eggleston, ISBN 4-88788-032-4, 2006.
IPCC: 2013 Supplement to the 2006 IPCC Guidelines for National Greenhouse Gas Inventories: Wetlands, Hiraishi, IPCC, Switzerland, ISBN 978-92-9169-139-5, 2014.
Little, B. G., Buckley, D. K., Cant, R., Henry, P. W. T., Jefferiss, A., Mather, J. D., Stark, J., and Young, R. N.: Land resources of the Bahamas: a summary, Tolworth Tower, Surbition, Surrey, 1–133, 1977.
Logar, I., Brouwer, R., and Paillex, A.: Do the societal benefits of river restoration outweigh their costs? A cost-benefit analysis, J. Environ. Manage., 232, 1075–1085, https://doi.org/10.1016/j.jenvman.2018.11.098, 2019.
Lupp, G., Zingraff-Hamed, A., Huang, J. J., Oen, A., and Pauleit, S.: Living labs – a concept for co-designing nature-base solutions, Sustainability (Switzerland), 13, 1–22, https://doi.org/10.3390/su13010188, 2021.
Maliva, R. G: Economics of managed aquifer recharge, Water, 6, 1257–1279, https://doi.org/10.3390/w6051257, 2014.
Martínez-Paz, J., Pellicer-Martínez, F., and Colino, J.: A probabilistic approach for the socioeconomic assessment of urban river rehabilitation projects, Land use policy, 36, 468–477, https://doi.org/10.1016/j.landusepol.2013.09.023, 2014.
MEA Millennium Ecosystem Assessment: Ecosystems and human well-being: Synthesis; a report of the Millennium Ecosystem Assessment, Island Press, Washington, DC, 2005.
Morgan, L. K. and Werner, A. D.: Seawater intrusion vulnerability indicators for freshwater lenses in strip islands, J. Hydrol., 508, 322–327, https://doi.org/10.1016/j.jhydrol.2013.11.002, 2014.
Nautiyal, H. and Goel, V.: Sustainability assessment: Metrics and methods, in: Methods in Sustainability Science Assessment, Prioritization, Improvement, Design and Optimization, edited by: Ren, J., Elsevier, 27–46, https://doi.org/10.1016/B978-0-12-823987-2.00017-9, 2021.
Network Nature: Deliverable 3.5. Report on practical, research and innovation needs WP3 Task 3.3, 17 pp., Network Nature, https://networknature.eu/sites/default/files/uploads/networknature-d35report-practical-research-and-innovation-needs.pdf (last access: 28 November 2024), 2022.
NRMMC-EPHC-AHMC: Australia Guidelines for Water Recycling: Managing Health and Environmental Risks (Phase 1) Natural Resource Management Ministerial Council; Environment Protection and Heritage Council, Australian Health Ninisters' Conference,415, 2009a.
NRMMC-EPHC-AHMC: Australian Guidelines for Water Recycling – Managed Aquifer Recharge, J. Environ. Manage., 27, 79–88, 2009b.
Peh, K. S. H., Balmford, A. P., Bradbury, R. B., Brown, C., Butchart, S. H. M., Hughes, F. M. R., MacDonald, M. A., Stattersfield, A. J., Thomas, D. H. L., and Trevelyan, R. J.: Toolkit for Ecosystem Service Site-based Assessment (TESSA), https://www.birdlife.org/tessa-tools/ (last access: 28 November 2024), 2017.
Perosa, F., Gelhaus, M., Zwirglmaier, V., Arias-Rodriguez, L. F., Zingraff-Hamed, A., Cyffka, B., and Disse, M.: Integrated Valuation of Nature-Based Solutions Using TESSA: Three Floodplain Restoration Studies in the Danube Catchment, Sustainability, 13, 1482, https://doi.org/10.3390/su13031482, 2021.
Perosa, F., Seitz, L. F., Zingraff-Hamed, A., and Disse, M.: Flood risk management along German rivers – A review of multi-criteria analysis methods and decision-support systems, Environ. Sci. Policy, 135, 191–206, https://doi.org/10.1016/j.envsci.2022.05.004, 2022.
Perosa, F.: Decision-Making Integrating Ecosystem Services for Floodplain Management in the Danube River Basin, Dissertation, https://mediatum.ub.tum.de/1686948 (last access: 28 November 2024), 2023.
Piyathilake, I. D. U. H., Udayakumara, E. P. N., Ranaweera, L. V, and Gunatilake, S. K.: Modeling predictive assessment of carbon storage using InVEST model in Uva province, Sri Lanka, Model. Earth Syst. Environ., 8, 2213–2223, https://doi.org/10.1007/s40808-021-01207-3, 2022.
QGIS.org: QGIS Geographic Information SystemQGIS Association, http://www.qgis.org (last access: 13 December 2024), 2024.
Raicy, M. C., Renganayaki, S. P., Brindha, K., and Elango, L.: Mitigation of seawater intrusion by managed aquifer recharge, Training course material on “Managed Aquifer Recharge: Methods, Hydrogeological requirements, Post and Pre-treatment Systems”, Anna University, Chennai, India, 11 and 12 December 2012, 70–81, 2012.
Ruangpan, L., Vojinovic, Z., Di Sabatino, S., Leo, L. S., Capobianco, V., Oen, A. M. P., McClain, M. E., and Lopez-Gunn, E.: Nature-based solutions for hydro-meteorological risk reduction: a state-of-the-art review of the research area, Nat. Hazards Earth Syst. Sci., 20, 243–270, https://doi.org/10.5194/nhess-20-243-2020, 2020.
Rupérez-Moreno, C., Pérez-Sánchez, J., Senent-Aparicio, J., Flores-Asenjo, P., and Paz-Aparicio, C.: Cost-Benefit Analysis of the Managed Aquifer Recharge System for Irrigation under Climate Change Conditions in Southern Spain, Water, 9, 343, https://doi.org/10.3390/w9050343, 2017.
Ruesch, A. and Gibbs, H. K.: Global ecofloristic zones mapped by the United Nations Food and Agricultural Organization, https://databasin.org/datasets/dc4f6efd1fa84ea99df61ae9c5b3b763/ (last access: 28 November 2024), 2008.
Saarikoski, H., Mustajoki, J., Barton, D. N., Geneletti, D., Langemeyer, J., Gomez-Baggethun, E., Marttunen, M., Antunes, P., Keune, H., and Santos, R.: Multi-Criteria Decision Analysis and Cost-Benefit Analysis: Comparing alternative frameworks for integrated valuation of ecosystem services, Ecosyst. Serv., 22, 238–249, https://doi.org/10.1016/j.ecoser.2016.10.014, 2016.
Sallwey, J., Bonilla Valverde, J. P., Vásquez López, F., Junghanns, R., and Stefan, C.: Suitability maps for managed aquifer recharge: A review of multi-criteria decision analysis studies, Environ. Rev., 27, 138–150, https://doi.org/10.1139/er-2018-0069, 2019.
NCP Natural Capital Project: InVEST 3.10.2 Stanford University, University of Minnesota, Chinese Academy of Sciences, The Nature Conservancy, World Wildlife Fund, Stockholm Resilience Centre and the Royal Swedish Academy of Sciences, https://naturalcapitalproject.stanford.edu/software/invest (last access: 13 December 2024), 2024.
SWA and WES, Smart Water Analytics LLC and Water & Earth Science Inc: Fast-Track Assessment of Damages by Hurricane Dorian on the Potable Water Sources and Infrastructure of Grand Bahama Island Technical Memorandum 1 Water Quality and Hydrogeology, 42 pp., 2019.
Soža, M. and Patekar, M.: DEEPWATER-CE WORKPACKAGE T3: Pilot feasibility study for MAR schemes with integrated environmnetal approach in karst geological conditions in semiarid karst region (Croatia), DEEPWATER-CE, https://programme2014-20.interreg-central.eu/Content.Node/DEEPWATER-CE.html (last access: 24 November 2024), 2022.
Sudmeier-Rieux, K., Nehren, U., Sandholz, S., and Doswald, N.: Disasters and Ecosystems: Resilience in a Changing Climate Source Book, Geneva: UNEP and Cologne: TH Köln – University of Applied Sciences, 1–216, https://doi.org/10.5281/zenodo.3493377, 2019.
Sudmeier-Rieux, K., Arce-Mojica, T., Boehmer, H. J., Doswald, N., Emerton, L., Friess, D. A., Galvin, S., Hagenlocher, M., James, H., Laban, P., Lacambra, C., Lange, W., McAdoo, B. G., Moos, C., Mysiak, J., Narvaez, L., Nehren, U., Peduzzi, P., Renaud, F. G., Sandholz, S., Schreyers, L., Sebesvari, Z., Tom, T., Triyanti, A., van Eijk, P., van Staveren, M., Vicarelli, M., and Walz, Y.: Scientific evidence for ecosystem-based disaster risk reduction, Nat. Sustain., 4, 803–810, https://doi.org/10.1038/s41893-021-00732-4, 2021.
Swierc, J., Page, D., and Leeuwen, J. Van: Preliminary Hazard Analysis and Critical Control Points Plan (HACCP) – Salisbury Stormwater to Drinking Water Aquifer Storage Transfer and Recovery (ASTR) Project of Montana, Water, 68 pp., 2005.
Terry, J. P. and Falkland, A. C.: Responses of atoll freshwater lenses to storm-surge overwash in the Northern Cook Islands, Hydrogeol. J., 18, 749–759, https://doi.org/10.1007/s10040-009-0544-x, 2010.
Thomas, A., Baptiste, A., Martyr-Koller, R., Pringle, P., and Rhiney, K.: Climate Change and Small Island Developing States, Annu. Rev. Environ. Resour., 45, 1–27, https://doi.org/10.1146/annurev-environ-012320-083355, 2020.
UNEP United Nations Environment Programme: Progress on Integrated Water Resources Management. Tracking SDG 6 series: global indicator 6.5.1 updates and acceleration needs, ISBN 978-92-807-3878-0, 2021.
United Nations Economic Commission for Latin America and the Caribbean: Assessment of the effects and impacts of hurricane Dorian in the Bahamas, https://doi.org/10.18235/0002582, 2021.
USACE: Water Resources Assessment of the Bahamas, Water Resources Assessment of the Bahamas, 1–114, 2004.
van Oosterzee, P., Liu, H., and Preece, N. D.: Cost benefits of forest restoration in a tropical grazing landscape: Thiaki rainforest restoration project, Global Environ. Chang., 63, 102105, https://doi.org/10.1016/j.gloenvcha.2020.102105, 2020.
Vecchi, G. A., Landsea, C., Zhang, W., Villarini, G., and Knutson, T.: Changes in Atlantic major hurricane frequency since the late-19th century, Nat. Commun., 12, 1–9, https://doi.org/10.1038/s41467-021-24268-5, 2021.
Vining, A. and Weimer, D. L.: An assessment of important issues concerning theapplication of benefit-cost analysis to social policy, Journal of Benefit-Cost Analysis, 1, 1–40. https://doi.org/10.2202/2152-2812.1013, 2010.
Villa, F., Bagstad, K. J., Voigt, B., Johnson, G. W., Portela, R., Honzák, M., and Batker, D.: A methodology for adaptable and robust ecosystem services assessment, PloS One, 9, e91001, https://doi.org/10.1371/journal.pone.0091001, 2014.
Vojinovic, Z., Keerakamolchai, W., Weesakul, S., Pudar, R. S., Medina, N., and Alves, A.: Combining ecosystem services with cost-benefit analysis for selection of green and grey infrastructure for flood protection in a cultural setting, Environments – MDPI, 4, 1–16, https://doi.org/10.3390/environments4010003, 2017.
Vollmer, D., Burkhard, K., Adem Esmail, B., Guerrero, P., and Nagabhatla, N.: Incorporating ecosystem services into water resources management-tools, policies, promisingpathways, Environ. Manage., 69, 627–635, https://doi.org/10.1007/s00267-022-01640-9, 2022.
Wang, W., Mu, J. E., and Ziolkowska, J. R.: Perceived Economic Value of Ecosystem Services in the US Rio Grande Basin, Sustainability, 13, 13798, https://doi.org/10.3390/su132413798, 2021.
Wegner, G. and Pascual, U.: Cost-benefit analysis in the context of ecosystem services for human well-being: A multidisciplinary critique, Global Environ. Change, 21, 492–504, https://doi.org/10.1016/j.gloenvcha.2010.12.008, 2011.
Welsh, K., Bowen-O'Connor, C., Stephens, M., Dokou, Z., Imig, A., Mackey, T., Moxey, A., Nikolopoulos, E., Turner, A., Williams, A., Al Baghdadi, L., Bowleg, J., Chaves, H. M. L., Davis, A., Guberman, G., Hanek, D., Klausner, S., Medlev, D., Mazzoni, N., Miller, I., Williams, L., and Wilchcombe, R.: Potable Water and Terrestrial Resources on Grand Bahama Post-Hurricane Dorian: Opportunities for Climate Resilience, International Journal of Bahamian Studies, 28, 43–66, https://doi.org/10.15362/ijbs.v28i0.467, 2022.
Whitaker, F. F. and Smart, P. L.: Control of Hydraulic Conductivity of Bahamian Limestones, Groundwater, 35, 859–868, https://doi.org/10.1111/j.1745-6584.1997.tb00154.x, 1997a.
Whitaker, F. F. and Smart, P. L.: Hydrogeology of the Bahamian archipelago, in: Geology and Hydrogeology of Carbonate islands, vol. 54, edited by: Vacher, H. L. and Quinn, T., Elsevier B.V., 183–216, https://doi.org/10.1016/S0070-4571(04)80026-8, 1997b.
Whitaker, F. F. and Smart, P. L.: Characterising scale-dependence of hydraulic conductivity in carbonates: Evidence from the Bahamas, J. Geochem. Explor., 69–70, 133–137, https://doi.org/10.1016/S0375-6742(00)00016-9, 2000.
Zegarra, M. A., Schmid, J. P., Palomino, L., and Seminario, B.: Impact of Hurricane Dorian in The Bahamas: A View from the Sky, Washington D.C., 1–13, https://doi.org/10.18235/0002163, 2020.