the Creative Commons Attribution 4.0 License.
the Creative Commons Attribution 4.0 License.
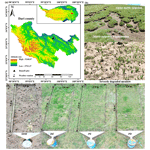
Mixed-cultivation grasslands enhance runoff generation and reduce soil loss in the restoration of degraded alpine hillsides
Yulei Ma
Yifan Liu
Jesús Rodrigo-Comino
Manuel López-Vicente
Gao-Lin Wu
Vegetation restoration is among the most effective measures for controlling runoff and soil erosion resulting from human activities. Nevertheless, few studies have been undertaken to analyze the effects of grassland restoration on maintaining local runoff, especially on alpine degraded hillsides where mixed-cultivation grasslands predominate. In this research, runoff plots were established to investigate the impact of three mixed-cultivation grasslands, each sowing two grass species per plot on a 20° slope: Deschampsia cespitosa and Elymus nutans (DE), Poa pratensis L. cv. Qinghai and Elymus nutans (PE), and Poa pratensis L. cv. Qinghai and Deschampsia cespitosa (PD). The activation and volume of surface runoff and the magnitude of soil loss on alpine degraded hillsides over 3 years (2019, 2020, and 2022) were assessed. A severely degraded meadow (SDM) plot was used as a control. The findings indicated that mixed-cultivation grasslands can effectively maintain runoff and reduce soil loss as planting age increases. Between 2019 and 2022, the values of the average runoff depth for DE, PE, PD, and SDM were 0.47, 0.55, 0.45, and 0.27 mm, respectively. Despite the increase in runoff, grassland restoration favored soil conservation: the net soil losses per unit area of SDM were 1.4, 1.3, and 1.9 times greater than those in DE, PE, and PD, respectively. The key factors affecting soil loss and runoff were rainfall amount, duration, and intensity (60 min intensity). We conclude that the results of this study can serve as scientific guides to formulate efficient policy decisions for planning the most effective vegetation restoration in severely degraded hillside alpine meadows. To improve the effectiveness of grassland restoration, we suggest that protective measures should be prioritized during the initial planting stage of cultivated grasslands.
- Article
(1648 KB) - Full-text XML
- BibTeX
- EndNote
Grasslands are an essential component of terrestrial ecosystems and habitats for the development of animal livestock (O'Mara, 2012). They make significant contributions to biodiversity conservation, climate mitigation, carbon sequestration, and water supply and regulation (Bardgett et al., 2021). Despite the importance of grasslands, about half of them are degraded globally, with 5 % of them undergoing severe degradation (based on net primary productivity), and this issue has become a major concern for landscape conservation (Gang et al., 2014; Török et al., 2021). Global grassland net primary productivity (NPP) has declined by 58.84 Tg C yr−1. Grassland degradation causes a loss of up to 90 % of the soil structure, which facilitates water movement (infiltration) and retention (water-holding capacity) in soils (Wick et al., 2016), reduces carbon storage potential (Liebig et al., 2013), and impedes soil functioning. Moreover, degraded grasslands are prone to severe soil erosion, especially in mountainous areas. For example, in the Swiss Alpine uplands, water erosion ranges from 0.14 to 1.25 t ha−1 per month according to the phenological stage of the grasses (Schmidt et al., 2019), and in the gully slope of the Loess Plateau the average amount of soil erosion was 306.7 t ha−1 per month during the 2018–2020 grass growing season (Zhu et al., 2021).
Precipitation is the main water source of soil moisture supply in semiarid areas, and the conversion of precipitation to runoff is one of the major contributors to river streamflow (Leung et al., 2015; Li et al., 2024). In some previous studies, it was observed that vegetation restoration reduced surface runoff and decreased sediment production, which led to lower river levels and threatened the health of river ecosystems (Dijk and Keenan, 2007). A recent study conducted by Wu et al. (2020) proposed sustainable management strategies for semiarid areas, with a positive tradeoff between surface runoff maintenance and erosion control. However, to date very few studies have addressed the effects of restored grasslands in maintaining surface runoff and preventing soil erosion (Minea et al., 2022). This topic is particularly important for alpine grasslands, which play a vital role in the supply of fresh water and the development of livestock husbandry (Cui et al., 2022). Therefore, it is necessary to assess the impacts of grassland restoration on runoff generation and soil protection.
Vegetation restoration is widely considered one of the most effective methods for controlling runoff and soil erosion worldwide (Anache et al., 2018; Vanacker et al., 2022). The effects of vegetation cover properties on runoff and soil loss reduction are strongly connected to plant species, leaf and branch cover, aboveground biomass, litter biomass, and root systems (Liu et al., 2022a; Freschet and Roumet, 2017; Gyssels et al., 2005; Zhu et al., 2021). Furthermore, the processes of runoff and soil loss are significantly influenced by the improvement of soil characteristics with vegetation restoration (Schwarz et al., 2015; Gyssels et al., 2005). The interaction between vegetation and soil could stabilize the topsoil and alter soil properties (Saxton and Rawls, 2006; Ma et al., 2023). Vegetation restoration promotes the formation of soil aggregates, decreases soil bulk density, enhances organic matter and nutrients, and improves soil porosity, resulting in high soil hydraulic conductivity and field capacity (Qiu et al., 2022; Saxton and Rawls, 2006). The soil properties interlinked above alter soil hydrological properties and ultimately influence hillslope and watershed hydrology, such as runoff and soil erosion (Lu et al., 2020; Qiu et al., 2022). While vegetation restoration has the potential to be a key method of environmental restoration under human management, inappropriate selection of species can negatively impact the sustainability of local economic and environmental development (Huang et al., 2017, 2019). For example, cultivated grasslands have already been advocated as a sensible solution for the conservation of soil and water as well as the regrowth of vegetation in semiarid mountain areas (Liu et al., 2022a; Wu et al., 2010). Grass communities with multiple stratified structures are better at maintaining surface runoff and decreasing soil loss than those with a single composition and structure (Mohammad and Adam, 2010).
Surface runoff – also known as storm water runoff or overland flow – reaches the stream in the forms of sheet, rill, and gully flows (Rumynin, 2015). The conversion of rainfall to overland flow depends on the rainfall intensity, the soil hydrological properties (e.g., (non)saturated hydraulic conductivity, matrix flux potential, and field capacity), and the initial soil water content (López-Vicente and Navas, 2012; Gyssels et al., 2005; De Baets et al., 2007). Because runoff is the primary driver of water erosion on hillslopes and serves as the main agent for sediment transport, reducing the conversion of rainfall to runoff is regarded as an effective way of controlling water erosion through vegetation restoration (Zhou et al., 2016; Zhu et al., 2021). On the other hand, in arid and semiarid regions, surface runoff is the major water supply source to the river streamflow, so it is vital for ensuring the sustainability of ecosystems and human activities (Liu et al., 2020; Robinson et al., 2003). Therefore, restoration efforts in areas with low rainfall should be oriented to maintain runoff while reducing its level of sediment concentration.
Soil erosion can be reduced by various factors, including the aboveground and belowground biomasses of grasses, litter cover, and root systems (De Baets et al., 2007; Durán Zuazo and Rodríguez Pleguezuelo, 2008; Gyssels and Poesen, 2003; Wen et al., 2024). Grasslands can control water erosion reliance on the roles of the aboveground biomass in dissipating flow energy (Bochet and García-Fayos, 2004), living roots in a decreasing soil detachment capacity (Zhang et al., 2013), grass plant cover in intercepting rainfall (Liu et al., 2019), and litter cover in enhancing rainwater infiltration (Liu et al., 2022b). Moreover, the interweaving of plant roots can remarkably alter the physical properties of the topsoil, enhancing its resistance to erosion (Schwarz et al., 2015; Wang et al., 2018). The impacts of grass roots on the soil characteristics can be summarized as follows: (i) increasing the stability of soil aggregates by aggregating fine soil particles into macroaggregates; (ii) enhancing soil cohesion by interweaving with the soil; and (iii) decreasing soil bulk density by increasing soil porosity (Wu et al., 2019; Gyssels et al., 2005). For example, numerous recent studies have confirmed that a grass with a shallow yet dense fibrous root system appears to be more effective at controlling water erosion than grass with good ground cover but low root density (De Baets et al., 2007; Bochet et al., 2006).
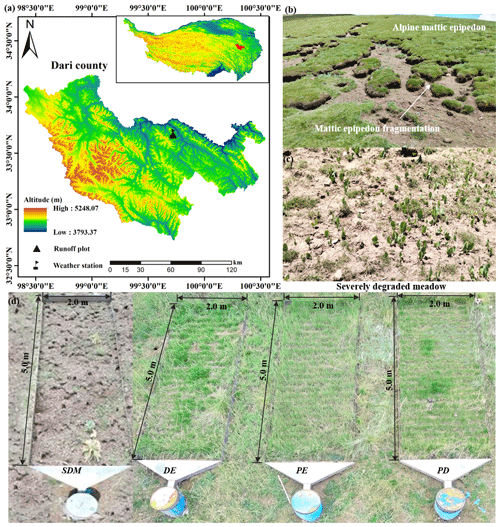
Figure 1The location of the study area on the Qinghai–Tibetan Plateau and the locations of runoff plots in the study area. (a) The location of the study area, (b) the fragmenting mattic epipedon on the alpine hillslope, (c) severely degraded meadow (SDM) formed by the disappearance of the mattic epipedon, and (d) four runoff plots of SDM and mixed-cultivation grasslands. A typical severely degraded meadow with a slope of 20° was selected to plant mixed grasses. Runoff plots were photographed with a drone in the early stages of the 2022 growing season: DE – Deschampsia cespitosa and Elymus nutans; PE – Poa pratensis L. cv. Qinghai and Elymus nutans; and PD – Poa pratensis L. cv. Qinghai and Deschampsia cespitosa.
Alpine meadows, especially on the Qinghai–Tibetan Plateau, constitute the predominant ecosystem in China and the world, accounting for 44 % and 6 % of total grassland areas, respectively (Wang et al., 2016). Over 50 % of alpine meadows have been subject to an increasing degree of degradation (Bardgett et al., 2021), with the extent of degradation depending on the meadow patch cover resulting from the fragmentation of alpine meadows (Fig. 1b). Severely degraded meadows (also known as “black beach” and “black soil-type degraded meadow”) formed after the mattic epipedon, typically 10 to 15 cm deep, was fully removed by overgrazing and rodent activities exposing the subsoil (Fig. 1c; Ma et al., 2023; Shang et al., 2008). Severely degraded meadows amounted to about 30 % of the total area of alpine meadows on the Qinghai–Tibetan Plateau (Shang et al., 2008). Recent studies by Niu et al. (2021) and Ma et al. (2024) observed that fragmentation of alpine meadows and severely degraded meadows could reduce surface runoff and enhance soil erosion.
The Qinghai–Tibetan Plateau serves as the headwater for many of Asia's major rivers (Xu, 2018). The eastern and southern parts of the Qinghai–Tibetan Plateau are influenced by the monsoon, and rainfall is the primary source of streamflow (Cuo et al., 2014). The long-term and widespread degradation of hillside alpine meadows has disrupted the soil water balance, reducing surface runoff (Niu et al., 2021; Ma et al., 2024). This, in turn, diminishes river streamflow, ultimately constraining the sustainable development of both local and downstream regions. The importance of artificial grassland in restoring alpine degraded meadows is widely accepted (Li et al., 2018; Wu et al., 2010). Artificial grassland – also known as tamed grassland, sowed grassland, and cultivated grassland – refers to fields that have been broken up and replanted with exotic grasses and forbs and utilized for hay crop production or cattle grazing (Fisher et al., 2018). The establishment of artificial grassland in severely degraded areas provides a dual benefit by boosting productivity and improving the ecological environment of alpine grasslands (Shang et al., 2008; Liu et al., 2022a).
While previous studies have often focused on the carbon sequestration capacity, vegetation characteristics, soil quality, and productivity of cultivated grasslands (Wang et al., 2013a; Bai and Cotrufo, 2022), there has been limited examination of the impacts of mixed-cultivation grasslands on the provision of runoff and prevention of soil erosion on alpine hillsides. Recently, Liu et al. (2022a) evaluated the effects of plant morphological characteristics on runoff and soil erosion in different mixed-cultivation grasslands during natural rainfall events. Here, we presented novel research to examine the ability of cultivated grasslands to regulate runoff and soil loss and to evaluate the effect of three different mixed-cultivation grasslands: Deschampsia cespitosa and Elymus nutans (DE), Poa pratensis L. cv. Qinghai and Elymus nutans (PE), and Poa pratensis L. cv. Qinghai and Deschampsia cespitosa (PD). These are compared to a severely degraded meadow (SDM) using a 3-year field experiment. In particular, this study aimed (1) to assess the temporal variations in soil and water loss of DE, PE, and PD grasslands during the growing season and under natural rainfall and (2) to determine the key factors influencing the mixed-cultivation grasslands in controlling runoff and soil erosion. This study has realistic implications for understanding the contribution of mixed-cultivation grassland restoration to soil erosion control on degraded alpine hillsides.
2.1 Study area
This study was carried out in the representative area of Zhique Village (33°40′01′′ N and 99°43′06′′ E; elevation over 4200 ), Dari County, Qinghai Province, which served as a field experimental site and model area for the restoration of severely degraded alpine meadow on the Qinghai–Tibetan Plateau (Fig. 1a). The climate conditions correspond to a typical highland with low temperatures throughout the year, i.e., not showing the typical four-season pattern (spring, summer, fall, and winter) but rather just two main seasons: cold and warm. In the study region, the average annual temperature is −3.1 °C, with monthly variations from −14.7 °C in January to 7.5 °C in July (values corresponded to the period 1981–2018; data source: European Centre for Medium-Range Weather Forecasts). The average annual precipitation is 416 mm, with the majority of it falling from July to September, based on Climate Hazards Group InfraRed Precipitation with Station (CHIRPS) data. The majority of the precipitation in the warm season falls during the vegetation growth period (from May to September), favoring optimal conditions for the development of plants. The soil type in the study area is classified as Mat Cryi-gelic Cambisols (IUSS-WRB, 2015). Currently, the remnant vegetation at this site is composed of alpine shrub (Salix cupularis and Potentilla fruticose), alpine meadow (Kobresia pygmaea, Kobresia humilis, and Kobresia capillifoli), and swamp meadow (Carex atrofusca, Poa annua, and Carex parva).
Soil erosion in the degraded alpine meadows is severe, having become the primary source of sediment delivered to streams in the study area (Liu et al., 2022a). The mattic epipedon of alpine meadow has experienced fragmentation and even disappearance (Fig. 1b), eventually forming a severely degraded meadow (Fig. 1c). Before the implementation of the grassland restoration project, i.e., the Subsidy and Incentive System for Grassland Conservation, the average soil erosion rate and the total erosion in the study area were 13.63 and 323.58 × 106 t yr−1, respectively (Zhao et al., 2021). Severely degraded meadows were restored via mixed-cultivation grasslands – fields were plowed and replanted with two grass species – and moderately degraded meadows were restored by broadcast sowing on the hillslopes during the implementation of the grassland restoration project. The grass species used for the projects have excellent characteristics like strong trampling tolerance, good palatability, abundant leaf quantity, and developed rhizomes, e.g., Poa pratensis L. cv. Qinghai, Deschampsia cespitosa, and Elymus nutans (Shang et al., 2008).
2.2 Experimental design and measurement
The degraded hillslopes are the main components of runoff generation and confluence areas on the Qinghai–Tibetan Plateau. Hence, the grass species chosen for mixed-cultivation grasslands must not only be grazing-tolerant and have good forage, but they must also prevent soil loss and maintain surface runoff. Potential grass species should also be fully acclimated to harsh alpine climates and have complementary morphological characteristics and living habits (Liu et al., 2022a). The community established by matching of grass morphological characteristics and habits has a hierarchical vertical cover structure and little interspecific or intraspecific competition. Following the abovementioned guidelines for choosing grass species, we ultimately decided on three species (Deschampsia cespitosa, Poa pratensis L. cv. Qinghai, and Elymus nutans) from the most widely utilized grass species. Deschampsia cespitosa is a cool-season bunching grass native to alpine environments. It typically forms a low, dense tussock (up to 30–50 cm tall) of very thin (0.5 cm wide), arching, flat to inrolled, dark-green grass blades (up to 5 cm long). Deschampsia cespitosa, a common bottom grass, has 70 % of its stems growing between 0 and 30 cm. Elymus nutans is a common and important plant species in the alpine meadows of the Qinghai–Tibetan Plateau (Chen et al., 2009). It is a valuable fodder grass in alpine locations that has been extensively employed for animal production, disturbed grassland restoration, and artificial grassland construction due to its resilience to cold, drought, and pests (Ren et al., 2010). Elymus nutans is a herbaceous perennial species with sparsely tufted culms that can grow to heights of 70 to 100 cm (Liu et al., 2022a). Poa pratensis L. cv. Qinghai is the common and dominant species native to the Qinghai–Tibetan Plateau. It is an excellent species that has been selected and cultivated to restore degraded alpine meadows. Also, Poa pratensis L. cv. Qinghai is a herbaceous perennial species with erect or geniculate base culms that grow 20–60 cm tall.
To reveal the effects of mixed-cultivation grasslands in controlling runoff and soil loss on hillsides, field observation of mixed grass plots designed by us was conducted for the 2019 to 2022 growing seasons. Therefore, one plot with SDM as a control and three plots with two mixed grass seeds per plot (Deschampsia cespitosa and Elymus nutans – DE; Poa pratensis L. cv. Qinghai and Elymus nutans – PE; Poa pratensis L. cv. Qinghai and Deschampsia cespitosa – PD) were selected as the testing sites (Fig. 1d). All four runoff plots were spaced 1 m apart and were located on the same hillside with the same elevation and soil texture. All the plots were bounded by steel plates (30 cm high and 2 mm thick sheet) and built during May 2019, with an area of 10 m2 (2 m wide and 5 m long parallel to the maximum slope gradient). To collect only runoff and soil loss from the runoff plot, the steel plate was placed vertically into the soil to a depth of about 10 cm, with the remainder sticking out from the soil surface. At the outlet of each plot, a steel runoff collection and a calibrated tank (75 L) were set up to gather sediment and runoff. To prevent the collected runoff from being lost to evaporation, the calibrated tank was set inside a sealed vat (Fig. 1d).
In addition, the grass seeding for each runoff plot was completed in May 2019. For the runoff plots, grass seeds were distributed to a depth of less than 1 cm in strips at 20 cm intervals following plowing. The seeding rate was set at 6.0 g m−2 for Poa pratensis L. cv. Qinghai and Deschampsia cespitosa and at 4.5 g m−2 for Elymus nutans to ensure a constant number of plants based on germination and seedling emergence rates. None of the runoff plots experienced any human disturbance during the observation period (2019–2022), including grazing, harvesting, and excavation.
2.3 Rainfall, runoff, and soil loss measurement
A Vantage pro 2™ weather station (Davis Instruments Corp., USA) with a measurement accuracy of 4 % was positioned next to the experimental plots to monitor precipitation intensity and duration (Fig. 1). Precipitation events were defined by the occurrence of a no-rain interval lasting more than 3 h between them. A total of 42 precipitation events were recorded from 2019 to 2022 throughout the growing seasons. Snow was not collected, and only rainfall was recorded during the growing seasons (from 15 June to 25 August). Precipitation characteristics of each event, including amount (P), duration (RD), and maximum intensities of 60 min (RI60), were recorded. The average rainfall intensity (ARI) was calculated by dividing the total rainfall amount by the duration of the rainfall event. After each rainfall-runoff event, both runoff and sediment were collected right away. The water level in the calibrated tank was measured first to calculate the runoff volume. Then, the runoff was fully mixed inside the calibrated tank using a stirring bar to thoroughly whirl it, and two 500 mL bottles were used to obtain mixture samples of sediment and runoff. When the calibrated tank had less than 1000 mL of runoff sample, all runoff was collected. Lastly, the calibrated tank was cleaned in order to collect sediment and runoff for the subsequent rainfall-runoff event. The mixture samples in the bottle were transported back to the lab to be filtered on filter paper with a pore size of 30–50 µm. The filter paper with sediment was oven-dried to a consistent weight at 105 °C. The ratio of the soil loss amount to the runoff volume in the mixed samples was applied to calculate the sediment concentration. Finally, the runoff volume and sediment concentration were multiplied to calculate the soil loss in each plot.
We collected runoff and soil erosion data during the growing season for the years 2019 to 2022. Data for 2021 could not be collected due to the prevention and control strategies for coronavirus (COVID-19). Soil erosion and runoff were portrayed in this work by soil erosion per unit area (g m−2) and runoff depth (mm). The runoff depth (R) and soil erosion per unit area (S) can be calculated using the following formulas:
where VR is the volume of runoff from runoff plots (m3), St is the total amount of soil erosion from runoff plots (g), and A is the area of the runoff plots (m2).
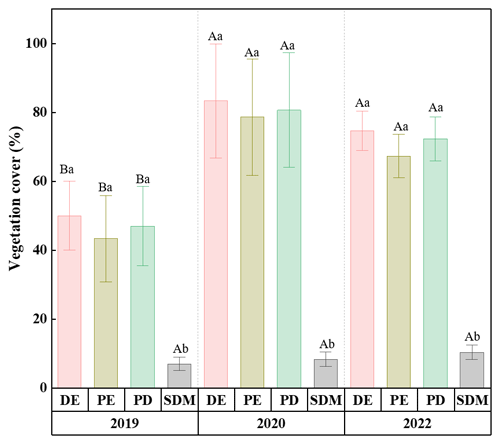
Figure 2Changes in vegetation cover in various mixed-cultivation grasslands from 2019 to 2022. Different capital letters mean that differences were significant in different years for the same grassland community, and different lowercase letters mean that differences were significant between different communities in the same year.
2.4 Vegetation and soil property measurement
Vegetation cover (VC), including dead (standing litter) and living vegetation, was measured monthly from the 2019 to 2022 growing seasons using a steel-wire frame (50 cm × 50 cm) subdivided into 25 plots of 10 cm × 10 cm. Figure 2 shows the changes in vegetation cover for all runoff plots from 2019 to 2022. After collecting runoff samples each year, the quadrants (50 cm × 50 cm) were positioned in the upslope, mid-slope, and downslope areas. Litter in each quadrant was collected and oven-dried to determine litter biomass (LB) (Zhu et al., 2021). The litter collection for 2019 was not completed due to the seeding of mixed-cultivation grasslands in May 2019, and the litter collection for 2020 and 2021 was done at the end of the runoff collection for the current year. Undisturbed soil samples were taken in the 0–10 cm soil layers using steel rings in 2022. All soil samples were saturated and then weighed (Wsat). The saturated soil samples were placed on the dry sand layer to drain water for about 2 and 8 h and then weighed (W2 h and W8 h). Finally, the soil samples were dried in an oven at 105 °C for 24 h and then weighed (Wdr). Based on the above measurements, soil bulk density (BD, g cm−3), total porosity (TP, %), capillary porosity (CP, %), non-capillary porosity (NCP, %), and soil water content at field capacity (FC, %) were determined as follows:
where Wsr is the weight of the steel ring (g), ds is the soil particle density (generally 2.65 g cm−3), and V is the volume of the ring (100 cm3).
In addition, root mass density (RMD) was obtained using a root drill, followed by washing with water and drying in the oven. Four undisturbed samples were collected in each quadrant using a steel ring (6.18 cm diameter and 2.0 cm height), and they were applied to a direct shear (ZJ type). The soil cohesion was obtained by Mohr–Coulomb theory (Labuz and Zang, 2012).
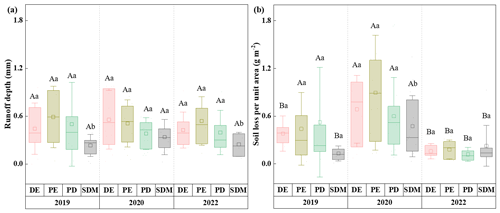
Figure 3Changes in soil erosion and runoff in various mixed-cultivation grasslands from 2019 to 2022. (a) Runoff depth and (b) soil loss per unit area. Note: for the four treatment runoff plots, runoff and sediment were measured 14, 18, and 10 times, respectively, during the growing seasons of 2019, 2020, and 2022. Different capital letters mean that differences were significant in different years for the same grassland community, and different lowercase letters mean that differences were significant between different communities in the same year for SDM, DE, PE, and PD. The lines in the middle of the box represent the median values. The squares in the box represent the average values.
2.5 Calculating the reduction effect of runoff and soil loss
Four metrics were employed to assess the efficiencies of the mixed-cultivation grasslands in regulating runoff and soil loss, i.e., the runoff reduction benefit (RRB, %), sediment concentration reduction benefit (CRB, %), soil erosion reduction benefit (SRB, %), and percentage of runoff reduction to soil loss reduction ratio (RRSR) (Zhao et al., 2014). High values of RRB, SRB, or CRB indicated that vegetation was able to reduce runoff, soil erosion, or sediment concentration compared to the rates observed in the control plot (severely degraded meadow). In addition, a low RRSR implied that vegetation was more beneficial in minimizing soil erosion than in minimizing runoff (Liu et al., 2020). These indices were calculated as follows:
where Rc and Rv are the runoff depths of the degraded meadow plot and plots covered by mixed-cultivation grasslands, Sc and Sv are the soil loss per unit area of the degraded meadow plot and plots covered by mixed-cultivation grasslands, and Cc and Cv are the sediment concentrations of the degraded meadow plot and plots covered by mixed-cultivation grasslands.
2.6 Statistical analyses
All data were analyzed using SPSS statistics software (IBM, USA, version 26.0). The Kolmogorov–Smirnov test was used to test the normality of the data. Duncan's multi-range tests of one-way analysis of variance (ANOVA) were applied to test for significant differences between soil and vegetation characteristics, runoff depth, soil erosion amount, and the runoff and soil loss reduction ratio in various mixed-cultivation grasslands at 0.05 significance levels. Also, path analysis is a form of multi-regression statistical analysis that is used to evaluate causal models by examining the relationships between runoff, soil loss, and soil and vegetation properties. By using this method, one can identify the major factors influencing runoff and soil loss and determine the direct and indirect effects of soil and vegetation properties on runoff and soil loss.
3.1 Mixed-cultivation grasslands modified runoff amounts and soil losses
Mixed-cultivation grasslands increased runoff and reduced soil erosion. One-way ANOVA revealed that runoff significantly (p < 0.05) increased after the severely degraded alpine hillside was restored by the mixed-cultivation grassland (Fig. 3). During the three evaluated growing seasons (2019, 2020, and 2022), the average runoff depths for DE, PE, PD, and SDM were 0.47, 0.55, 0.45, and 0.27 mm, respectively. The average runoff depths of SDM in 2019, 2020, and 2022 were 0.23, 0.34, and 0.25 mm, respectively, all significantly (p < 0.05) lower than (except for 2020) the average runoff of mixed-cultivation grassland DE, PE, and PD, which measured 0.44, 0.59, and 0.50 mm in 2019; 0.55, 0.51, and 0.38 mm in 2020; and 0.43, 0.54, and 0.40 mm in 2022 (Fig. 3a). Regarding soil conservation, the amount of soil loss in grasslands was significantly influenced by the planting age. As depicted in Fig. 3b, soil losses in DE, PE, and PD (except for DE in 2019) were significantly (p < 0.05) higher in 2019 and 2020 (the first and second years of planting) than those in the fourth year of planting (2022). In 2020, soil losses produced by DE, PE, and PD were significantly higher (p < 0.05) than those of SDM. Satisfactorily, the three mixed-cultivation grasslands did exhibit a clear reduction in soil loss compared to SDM in 2022 (albeit not significantly), with soil losses per unit area for SDM being 1.4, 1.3, and 1.9 times higher than those for DE, PE, and PD, respectively. No significant difference (p > 0.05) was observed in runoff depth and soil loss between DE, PE, and PD in 2019, 2020, and 2022. The results showed that any of the three mixed-cultivation grasslands (DE, PE, and PD) could be effective in controlling soil loss and maintaining runoff.
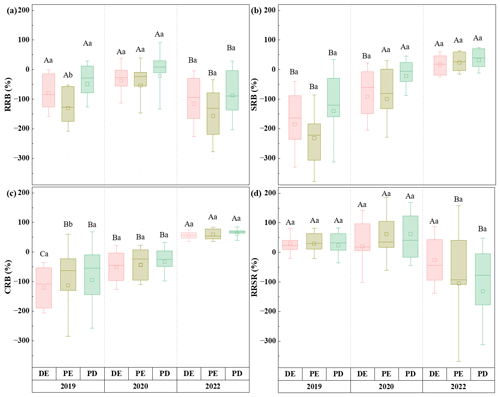
Figure 4Runoff, soil loss, and sediment concentration reduction ratio in different mixed-cultivation grasslands from 2019 to 2022. (a) Runoff reduction ratio (RRB), (b) soil loss reduction ratio (SRB), (c) sediment concentration reduction ratio (CRB), and (d) percent of runoff reduction ratio to soil loss reduction ratio (RRSR). Note: different capital letters mean that differences were significant in different years for the same grassland community, and different lowercase letters mean that differences were significant between different communities in the same year. The lines in the middle of the box represent the median values. The squares in the box represent the average values.
3.2 Specific runoff and soil loss reduction ratios of the cultivated grasslands
Figure 4 illustrates the runoff, soil loss, and sediment concentration reduction ratio after planting various mixed-cultivation grasslands. Lower RRB values indicated a better ability to maintain runoff for mixed-cultivation grasslands, while higher SRB and CRB values indicated better effectiveness of grasslands in soil loss reduction. The mean RRB values of the grassland communities DE, PE, and PD were −79.3 %, −130.4 %, and −48.5 % in 2019; −36.9 %, −53.5 %, and −21.5 % in 2020; and −115.4 %, −156.1 %, and −87.6 % in 2022, respectively (Fig. 4a). Regardless of the combination of the abovementioned grass species, the average increase ratio of runoff in 2022 (the fourth year of planting) was significantly (p < 0.05) higher than those in 2019 and 2020 (the first and second years of planting). The SRB of the three mixed-cultivation grasslands (DE, PE, and PD) increased with increasing planting age. It is worth noting that the average SRB values in the grassland communities of DE, PE, and PD were 18.0 %, 24.3 %, and 31.9 %, respectively, in 2022 (Fig. 4b). The SRB values of DE, PE, and PD in 2022 were significantly (p < 0.05) higher than those of 2019, whereas the SRE values between 2020 and 2022 were significant (p < 0.05) for DE but not (p > 0.05) for PE and PD. Additionally, the CRB for all mixed-cultivation grasslands in 2022 was significantly (p < 0.05) higher than those in 2019 and 2020. The mean CRB values of the cultivated-grassland communities DE, PE, and PD increased from −120.9 % to 55.8 %, from −112.4 % to 59.7 %, and from −94.3 % to 62.1 % from 2019 to 2022, respectively (Fig. 4c). Regardless of the age of the grasslands, the value of RRSR was less than 1, suggesting that the soil erosion reduction effect of the grasslands was higher than its runoff reduction effect (Fig. 4d). No significant differences (p > 0.05) appeared in RRB, SRB, CRB, and RRSR between DE, PE, and PD in 2019, 2020, and 2022.
Table 1Results of path analysis of the factors affecting runoff depth.
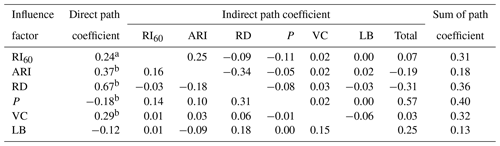
Note: RI60 is the maximum 60 min intensity (mm h−1), ARI is the average rainfall intensity (mm h−1), RD is the rainfall duration (h), P is the rainfall amount (mm), VC is the vegetation cover (%), and LB is the litter biomass (g m−2). a means the correlation is significant at the 0.05 significance level, and b means the correlation is significant at the 0.01 significance level.
Table 2Results of path analysis of the factors affecting soil loss per unit area.
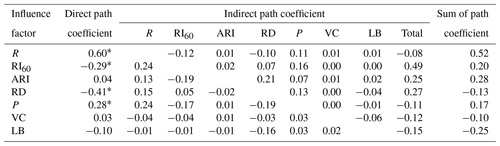
Note: R is the surface runoff (mm), RI60 is the maximum 60 min intensity (mm h−1), ARI is the average rainfall intensity (mm h−1), RD is the rainfall duration (h), P is the rainfall amount (mm), VC is the vegetation cover (%), and LB is the litter biomass (g m−2). ∗ means the correlation is significant at the 0.01 significance level.
3.3 Key factors affecting runoff and soil loss
Precipitation characteristics and vegetation features played a significant role in influencing the hydrological response of the soil. In this study, path analysis was applied to identify the key factors affecting soil loss. The results of this analysis indicated that the path coefficients of RI60, RD, P, and VC were 0.31, 0.36, 0.40, and 0.32, respectively (Table 1). This suggests that P, RD, VC, and RI60 had positive effects on the runoff amount, with P being the most influential factor. Direct influences on runoff were primarily attributed to the ARI and RD, with direct path coefficients of 0.37 and 0.67, respectively. Meanwhile, the influences of P and LB on runoff were mainly indirect, with indirect path coefficients of 0.57 and 0.25, respectively. For instance, P, in combination with other factors, particularly RI60 and RD, contributed significantly to runoff.
Soil loss was significantly influenced by R, RI60, ARI, and LB. The path coefficients of R, RI60, ARI, and LB were 0.52, 0.20, 0.28, and −0.25, respectively (Table 2). These results show that R, RI60, and ARI had a promotional effect on soil loss, whereas LB had an inhibitory effect on soil loss. Meanwhile, R and P had a direct positive influence on soil erosion, with direct path coefficients of 0.60 and 0.28, whereas RI60 and RD had a direct negative influence on soil erosion, with direct path coefficients of −0.29 and −0.41 (Table 2). In addition, the direct and indirect path coefficients both indicated that LB had an inhibitory influence on the soil loss per unit area, with values of −0.10 and −0.25, respectively.
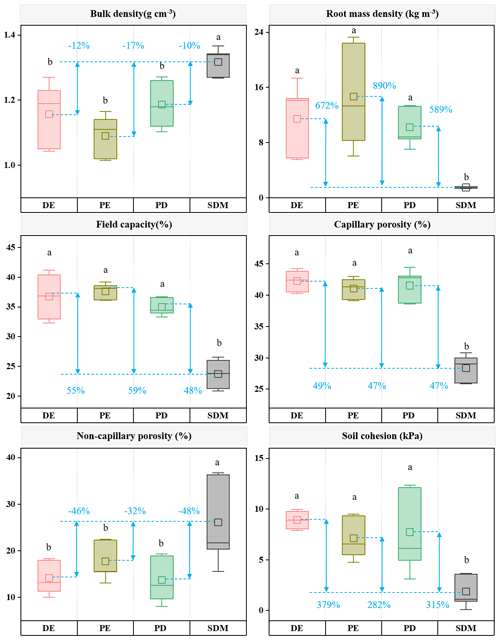
Figure 5Changes in bulk density, root mass density, field capacity, capillary capacity, non-capillary porosity, and soil cohesion in the 0–10 cm soil layer when SDM was restored to mixed-cultivation grassland for 4 years for DE, PE, and PD. Percentages represent the increased rate of soil properties (increased rate = (VDE or VPE or ), where VSDM, VDE, VPE, and VPD are the mean values of soil characteristics of SDM, DE, PE, and PD. Different lowercase letters mean that differences were significant between different communities. The lines in the middle of the box represent the median values. The squares in the box represent the average values.
4.1 Benefits of mixed-cultivation grasslands for soil conservation and runoff maintenance
The mixed-cultivation grasslands (DE, PE, and PD) effectively maintained runoff and minimized soil loss (Fig. 4). This finding is similar to those of some previous studies (Liu et al., 2019, 2022a). In this study, the mixed-cultivation grasslands significantly increased surface runoff compared to the SDM. The difference in runoff between mixed-cultivation grasslands and SDM may be attributed to the soil infiltration rate. Mixed-cultivation grasslands had more abundance of fibrous roots in the topsoil compared with SDM (Fig. 5), and those fine roots reduced infiltration by occupying the soil pores (Leung et al., 2015). In comparison to SDM, the soil NCP and FC of DE, PE, and PD significantly decreased by 46 %, 32 %, and 48 % and increased by 55 %, 59 %, and 48 %, respectively (Fig. 5). This implied that SDM was restored to mixed-cultivation grasslands with lower permeability and better water retention.
Soil loss in all three mixed-cultivation grassland communities (DE, PE, and PD) was higher than that in the SDM during the first and second years following planting. However, by the fourth year, the SDM exhibited higher soil loss than the three mixed-cultivation grasslands (Fig. 3). These changes in soil erosion were predominantly attributed to the development of the root system and the improvement of the soil structure (Zhu et al., 2021). The seeding method of plowing led to a disruption of the soil structure, resulting in increased soil loss in the three mixed-cultivation grasslands during the initial stages of planting. We confirmed that the mixed-cultivation grassland age was a key factor in understanding the interannual changes in soil erosion. This idea was also demonstrated in other types of primary land uses, e.g., woody crops or young forests (Rodrigo-Comino et al., 2018). Nevertheless, we hypothesize that grassland topsoil demonstrated a stronger resilience to erosion as its root system grew, which had a reinforcement impact on the soil and led to lower soil loss in the fourth year of planting than that of the SDM. The topsoil (0–10 cm) of the grasslands had significantly different soil properties from the SDM in the fourth year after planting, as detailed in Fig. 5. In comparison to the SDM, the root mass density and soil cohesion of grasslands DE, PE, and PD increased by 672 %, 890 %, and 589 % and by 379 %, 282 %, and 315 %, respectively.
4.2 Effects of rainfall and vegetation characteristics on runoff and soil loss
Surface runoff and erosion processes are influenced and constrained by rainfall depth, intensity, and duration as well as by VC (Mohamadi and Kavian, 2015; Bochet et al., 2006). In this study, VC had a directly posiitive effect on surface runoff. Moreover, this result was in line with the finding of Niu et al. (2021), who reported that the surface runoff increased with the grassland cover. Our results also indicated that rainfall amount (P) could have an indirect effect on surface runoff through RD and RI60. This implies that heavier and longer-lasting rainfall events were more likely to lead to surface runoff generation (Dos Santos et al., 2017). The findings demonstrated that R and ARI were the most and second most influential factors in promoting soil erosion (Table 2). The primary causes of this are runoff velocity increases with higher precipitation intensities (Wang et al., 2013b), which likely enhances the capacity of soil detachment and transport by surface runoff (Zhu et al., 2021). Furthermore, LB had a direct and negative impact on soil loss (Table 2), indicating that the effectiveness of grasslands in reducing soil loss increased as LB increased. Liu et al. (2022b) found that the soil loss rate decreased with increasing litter biomass in grasslands. Plant litter can intercept precipitation, reducing rainfall kinetic energy and splash erosion while also increasing surface roughness (Liu et al., 2017; Xia et al., 2019). All these processes favor a reduction in runoff yield and soil loss rates.
Grass combinations with different morphological characteristics can effectively reduce soil loss (Liu et al., 2022a). In this study, the reduction in soil loss in the early stages of mixed-cultivation grassland planting (2019 and 2020) was attributed to grassland cover and plant morphological characteristics. Deschampsia cespitosa, Poa pratensis L. cv. Qinghai, and Elymus nutans are dense clump, rhizomatic sparse clump, and sparse clump perennial grasses, respectively. In addition, Deschampsia cespitosa and Poa pratensis L. cv. Qinghai are bottom grasses, while Elymus nutans is a top grass. The mix of dense and sparse grasses (DE and PD) and the mix of top and bottom grasses (DE and PE) can complement each other morphologically and structurally, thereby more effectively reducing the kinetic energy of raindrops (Liu et al., 2022a). Poa pratensis L. cv. Qinghai, a rhizomatic grass, also has abundant root systems intertwined with the soil, increasing soil cohesion and consequently reducing soil detachment capacity (Wang et al., 2018). Overall, in this study, the morphological and root characteristics of mixed-cultivation grasslands reduced the runoff velocity, influenced the water infiltration process, and decreased the soil erodibility.
4.3 Implications for grassland restoration on degraded alpine hillsides
Our findings demonstrated that mixed-cultivation grasslands with complementary morphological features and habits can be effective in maintaining runoff and reducing soil erosion. Three mixed-cultivation grasslands (DE, PE, and PD) exhibited an effective role in controlling soil loss on the degraded alpine hillside. However, at the start of planting, the mixed planted grassland had greater soil erosion than the severely degraded meadow, whereas soil loss was reduced in the fourth year of planting (Figs. 2 and 3). This suggested that protection measures, such as mesh covering and anti-trampling, may be taken into account to reduce soil loss in the initial planting stage of cultivated grassland on alpine hillsides (Liu et al., 2022a). Moreover, grass may also be planted with a no-till system to avoid the initial increase in soil erosion in the initial phases of cultivated grassland by destroying soil structure (Karayel and Sarauskis, 2019). In addition, spring meltwater is the main driver of soil erosion in degraded alpine meadows in alpine regions, which greatly increases the turbidity of rivers (Shi et al., 2020). The restoration of severely degraded hillslope meadows increased vegetation cover and soil ability, both of which could have an inhibitory impact on meltwater erosion (Liu et al., 2022a). To better understand the effects of cultivated grassland on meltwater erosion, future experiments under natural freezing and thawing conditions need to be conducted and monitored.
Cultivated grasslands, considered a crucial component of vegetation restoration, have been extensively utilized in the rehabilitation of degraded alpine hillsides (Shang et al., 2008). Nevertheless, plant restoration is not necessarily beneficial to the long-term viability of on- and off-site ecosystems' functions, including natural succession and river ecosystems. Therefore, the selected vegetation types ought to be advantageous for ecosystems' sustainability, both on- and off-site, such as maintaining river streamflow and unrestricted natural succession. The seed prices of cultivated grass communities of Deschampsia cespitosa and Elymus nutans, Poa pratensis L. cv. Qinghai and Elymus nutans, and Poa pratensis L. cv. Qinghai and Deschampsia cespitosa were about USD 690, USD 750, and USD 480 ha−1, respectively, in Xining, Qinghai Province, in 2019. Planting proper mixed-cultivation grassland on alpine degraded hillsides can achieve both environmental and economic benefits. This study proved that mixed-cultivation grasslands can maintain runoff and decrease soil loss.
Based on the measured data during the 2019, 2020, and 2022 growing seasons, the planting of mixed-cultivation grasslands in severely degraded hillside alpine meadows effectively maintained surface runoff and decreased soil loss, especially after the mixed-cultivation grassland played a positive role in consolidating the soil surface. The benefits were statistically significant compared with the control plot, but differences between the three types of cultivated grasslands were not significant. Planting the mixed-cultivation grasslands after plowing loosened the soil structure and thus increased the sediment concentration in runoff during the first stage after planting. Subsequently, sediment concentration decreased with the growth of the root system of the mixed-cultivation grasslands, improving root–soil cohesion due to the root architecture. To guarantee that mixed-cultivation grasslands can perform the aforementioned functions, protective measures should be implemented during the initial planting stage to support their healthy growth. Our results also suggested that mixed-cultivation grasslands with different but complementary morphologies and structures and abundant fine-root systems were effective in maintaining surface runoff and reducing soil erosion. Precipitation amount, duration, maximum 60 min intensity, vegetation cover, and composition were the predominant factors affecting surface runoff and soil loss. The erosion resistance contribution of the aboveground community characteristics and belowground roots during the cultivation time could maintain a relatively high surface runoff and decrease the sediment concentration. These findings have potential implications for understanding the contribution of mixed-cultivation grassland restoration to soil erosion control on the degraded hillsides of alpine areas.
The data that support the findings of this study are available on request from the corresponding author.
YM: investigation; formal analysis; methodology; software; writing – original draft. YL: investigation; formal analysis; writing – review and editing. JRC: interpretation of data; writing – review and editing. MLV: interpretation of data; writing – review and editing. GLW: conceptualization; funding acquisition; supervision; writing – original draft, review, and editing.
The contact author has declared that none of the authors has any competing interests.
Publisher's note: Copernicus Publications remains neutral with regard to jurisdictional claims made in the text, published maps, institutional affiliations, or any other geographical representation in this paper. While Copernicus Publications makes every effort to include appropriate place names, the final responsibility lies with the authors.
We thank Nunzio Romano, Gall Corinna, Vanacker Veerle, and Qianjin Liu for their constructive comments and suggestions for this paper.
This research has been supported by the National Natural Science Foundation of China (grant nos. NSFC41930755 and NSFC32230068), the Chinese Academy of Sciences (grant no. XDB40000000), and the Qinghai Provincial Department of Science and Technology (grant no. 2019-S-1).
This paper was edited by Nunzio Romano and reviewed by Veerle Vanacker and Corinna Gall.
Anache, J. A. A., Flanagan, D. C., Srivastava, A., and Wendland, E. C.: Land use and climate change impacts on runoff and soil erosion at the hillslope scale in the Brazilian Cerrado, Sci. Total Environ., 622–623, 140–151, https://doi.org/10.1016/j.scitotenv.2017.11.257, 2018.
Bai, Y. and Cotrufo, M. F.: Grassland soil carbon sequestration: Current understanding, challenges, and solutions, Science, 377, 603–608, https://doi.org/10.1126/science.abo2380, 2022.
Bardgett, R. D., Bullock, J. M., Lavorel, S., Manning, P., Schaffner, U., Ostle, N., Chomel, M., Durigan, G., Fry, E. L., Johnson, D., Lavallee, J. M., Le Provost, G., Luo, S., Png, K., Sankaran, M., Hou, X. Y., Zhou, H. K., Ma, L., Ren, W. B., Li, X. L., Ding, Y., Li, Y. H., and Shi, H. X.: Combatting global grassland degradation, Nat. Rev. Earth Env., 2, 720–735, https://doi.org/10.1038/s43017-021-00207-2, 2021.
Bochet, E. and García-Fayos, P.: Factors controlling vegetation establishment and water erosion on motorway slopes in Valencia, Spain, Restor. Ecol., 12, 166–174, https://doi.org/10.1111/j.1061-2971.2004.0325.x, 2004.
Bochet, E., Poesen, J., and Rubio, J. L.: Runoff and soil loss under individual plants of a semi-arid Mediterranean shrubland: influence of plant morphology and rainfall intensity, Earth Surf. Proc. Land., 31, 536–549, https://doi.org/10.1002/esp.1351, 2006.
Chen, S., Ma, X., Zhang, X., and Chen, Z.: Genetic variation and geographical divergence in Elymus nutans Griseb. (Poaceae: Triticeae) from West China, Biochem. Syst. Ecol., 37, 716–722, https://doi.org/10.1016/j.bse.2009.12.005, 2009.
Cui, Z., Liu, Y. F., Liu, Y., Leite, P. A. M., Shi, J. J., Shi, Z. H., and Wu, G. L.: Fragmentation alters the soil water conservation capacity of hillside alpine meadows on the Qinghai-Tibetan Plateau, Geoderma, 428, 116133, https://doi.org/10.1016/j.geoderma.2022.116133, 2022.
Cuo, L., Zhang, Y. X., Zhu, F. X., and Liang, L. Q.: Characteristics and changes of streamflow on the Tibetan Plateau: A review, J. Hydrol.-Reg. Stud., 2, 2014, 49–68, https://doi.org/10.1016/j.ejrh.2014.08.004, 2014.
De Baets, S., Poesen, J., Knapen, A., Barberá, G. G., and Navarro, J. A.: Root characteristics of representative Mediterranean plant species and their erosion-reducing potential during concentrated runoff, Plant Soil, 294, 169–183, https://doi.org/10.1007/s11104-007-9244-2, 2007.
Dijk, A. I. J. M. and Keenan, R. J.: Planted forests and water in perspective, Forest Ecol. Manag., 251, 1–9, https://doi.org/10.1016/j.foreco.2007.06.010, 2007.
Dos Santos, J. C. N., de Andrade, E. M., Medeiros, P. H. A., Guerreiro, M. J. S., and de Queiroz Palácio, H. A.: Effect of Rainfall Characteristics on Runoff and Water Erosion for Different Land Uses in a Tropical Semiarid Region, Water Resour. Manag., 31, 173–185, https://doi.org/10.1007/s11269-016-1517-1, 2017.
Durán Zuazo, V. H. and Rodríguez Pleguezuelo, C. R.: Soil-erosion and runoff prevention by plant covers. A review, Agron. Sustain. Dev., 28, 65–86, https://doi.org/10.1051/agro:2007062, 2008.
Fisher, R. J., Sawa, B., and Prieto, B.: A novel technique using LiDAR to identify native-dominated and tame-dominated grasslands in Canada, Remote Sens. Environ., 218, 201–206, https://doi.org/10.1016/j.rse.2018.10.003, 2018.
Freschet, G. T. and Roumet, C.: Sampling roots to capture plant and soil functions, Funct. Ecol., 31, 1506–1518, https://doi.org/10.1111/1365-2435.12883, 2017.
Gang, C. C., Zhou, W., Chen, Y. Z., Wang, Z. Q., Sun, Z. G., Li, J. L., Qi, J. G., and Odeh, I.: Quantitative assessment of the contributions of climate change and human activities on global grassland degradation, Environ. Earth Sci., 72, 4273–4282, https://doi.org/10.1007/s12665-014-3322-6, 2014.
Gyssels, G. and Poesen, J.: The importance of plant root characteristics in controlling concentrated flow erosion rates, Earth Surf. Proc. Land., 28, 371–384, https://doi.org/10.1002/esp.447, 2003.
Gyssels, G., Poesen, J., Bochet, E., and Li, Y.: Impact of plant roots on the resistance of soils to erosion by water: a review, Prog. Phys. Geog., 29, 189–217, https://doi.org/10.1191/0309133305pp443ra, 2005.
Huang, Z., Tian, F. P., Wu, G. L., Liu, Y., and Dang, Z. Q.: Legume grasslands promote precipitation infiltration better than gramineous grasslands in arid regions, Land Degrad. Dev., 28, 309–316, https://doi.org/10.1002/ldr.2635, 2017.
Huang, Z., Liu, Y. F., Cui, Z., Liu, Y., Wang, D., Tian, F. P., and Wu, G. L.: Natural grasslands maintain soil water sustainability better than planted grasslands in arid areas, Agr. Ecosyst. Environ., 286, 106683, https://doi.org/10.1016/j.agee.2019.106683, 2019.
IUSS-WRB: World Reference Base for soil resources 2014, International soil classification system for naming soils and creating legends for soil maps, Update 2015, World Soil Resources Report 106, FAO, Rome, 2014.
Karayel, D. and Šarauskis, E.: Environmental impact of no-tillage farming, Environ. Res. Eng. Manag., 75, 7–12,https://doi.org/10.5755/j01.erem.75.1.20861, 2019.
Labuz, J. F. and Zang, A.: Mohr–Coulomb Failure Criterion, Rock Mech. Rock Eng., 45, 975–979, https://doi.org/10.1007/s00603-012-0281-7, 2012.
Leung, A. K., Garg, A., Coo, J. L., Ng, C. W. W., and Hau, B. C. H.: Effects of the roots of Cynodon dactylon and Schefflera heptaphylla on water infiltration rate and soil hydraulic conductivity, Hydrol. Process., 29, 3342–3354, https://doi.org/10.1002/hyp.1045, 2015.
Li, J., Wu, J., Yu, J., Wang, K., Li, J., Cui, Y., Shangguan Z. P., and Deng, L.: Soil enzyme activity and stoichiometry in response to precipitation changes in terrestrial ecosystems, Soil Biol. Biochem., 191, 109321, https://doi.org/10.1016/j.soilbio.2024.109321, 2024.
Li, W., Wang, J. L., Zhang, XJ., Shi, SL., and Cao, W. X.: Effect of degradation and rebuilding of artificial grasslands on soil respiration and carbon and nitrogen pools on an alpine meadow of the Qinghai-Tibetan Plateau, Ecol. Eng., 111, 134–142, https://doi.org/10.1016/j.ecoleng.2017.10.013, 2018.
Liebig, M. A., Kronberg, S. L., Hendrickson, J. R., Dong, X., and Gross, J. R.: Carbon dioxide efflux from long-term grazing management systems in a semiarid region, Agr. Ecosyst. Environ., 164, 137–144, https://doi.org/10.1016/j.agee.2012.09.015, 2013.
Liu, W. J., Luo, Q. P., Lu, H. J., Wu, J. E., and Duan, W. P.: The effect of litter layer on controlling surface runoff and erosion in rubber plantations on tropical mountain slopes, SW China, Catena, 149, 167–175, https://doi.org/10.1016/j.catena.2016.09.013, 2017.
Liu, Y., Li. S. Y., Niu, Y. L., Cui, Z., Zhang, Z. C., Wang, Y. L., Ma, Y. S., López-Vicente, M., and Wu, G. L.: Effectiveness of mixed cultivated grasslands to reduce sediment concentration in runoff on hillslopes in the Qinghai-Tibetan Plateau, Geoderma, 422, 115933, https://doi.org/10.1016/j.geoderma.2022.115933, 2022a.
Liu, Y., Zhao, L. R., Liu, Y. F., Huang, Z., Shi, J. J., Wang, Y. L., Ma, Y. S., Lucas-Borja, M. E., López-Vicente, M., and Wu, G. L.: Restoration of a hillslope grassland with an ecological grass species (Elymus tangutorum) favors rainfall interception and water infiltration and reduces soil loss on the Qinghai-Tibetan Plateau, Catena, 219, 106632, https://doi.org/10.1016/j.catena.2022.106632, 2022b.
Liu, Y. F., Liu, Y., Wu, G. L., and Shi, Z. H.: Runoff maintenance and sediment reduction of different grasslands based on simulated rainfall experiments, J. Hydrol., 572, 329–335, https://doi.org/10.1016/j.jhydrol.2019.03.008, 2019.
Liu, Y. F., Dunkerley, D., López-Vicente, M., Shi, Z. H., and Wu, G. L.: Trade-off between surface runoff and soil erosion during the implementation of ecological restoration programs in semiarid regions: A meta-analysis, Sci. Total Environ., 712, 136477, https://doi.org/10.1016/j.scitotenv.2019.136477, 2020.
López-Vicente, M. and Navas, A.: A new distributed rainfall-runoff (DR2) model based on soil saturation and runoff cumulative processes, Agr. Water Manage., 104, 128–141, https://doi.org/10.1016/j.agwat.2011.12.007, 2012.
Lu, J. R., Zhang, Q., Werner, A. D., Li, Y. L., Jiang, S. Y., and Ta, Z. Q.: Root-induced changes of soil hydraulic properties – a review, J. Hydrol., 589, 125203 https://doi.org/10.1016/j.jhydrol.2020.125203, 2020.
Ma, Y. L., Liu, Y. F., López-Vicente, M., and Wu G. L.: Divergent shift of normal alpine meadow towards shrub and degraded meadows reduces soil water retention and storage capacity, J. Hydrol., 625, 130109, https://doi.org/10.1016/j.jhydrol.2023.130109, 2023.
Ma, Y. L., Liu, Y. F., López-Vicente, M., and Wu, G. L.: Divergent shift of normal alpine meadow exacerbated soil loss of hillslope alpine meadows based on field experiments, Int. Soil Water Conserv. Res., 12, 565–577, https://doi.org/10.1016/j.iswcr.2023.11.007, 2024.
Minea, G., Mititelu-Ionu?, O., Gyasi-Agyei, Y., Ciobotaru, N., and Rodrigo-Comino, J.: Impacts of grazing by small ruminants on hillslope hydrological processes: A review of European current understanding, Water Resour. Res., 58, e2021WR030716, https://doi.org/10.1029/2021WR030716, 2022.
Mohamadi, M. A. and Kavian, A.: Effects of rainfall patterns on runoff and soil erosion in field plots, Int. Soil Water Conserv. Res., 3, 273–281, https://doi.org/10.1016/j.iswcr.2015.10.001, 2015.
Mohammad, A. G. and Adam, M. A.: The impact of vegetative cover type on runoff and soil erosion under different land uses, Catena, 81, 97–103, https://doi.org/10.1016/j.catena.2010.01.008, 2010.
Niu, Y. L., Li, S. Y., Liu, Y., Shi, J. J., Wang, Y. L., Ma, Y. S., and Wu, G. L.: Regulation of alpine meadow patch coverage on runoff and sediment under natural rainfall on the eastern Qinghai-Tibetan Plateau, J. Hydrol., 603, 127101, https://doi.org/10.1016/j.jhydrol.2021.127101, 2021.
O'Mara, F. P.: The role of grasslands in food security and climate change, Ann. Bot.-London, 110, 1263–1270, https://doi.org/10.1093/aob/mcs209, 2012.
Qiu, D. X., Xu, R. R., Wu, C. X., Mu, X. M., Zhao, G. J., and Gao P.: Vegetation restoration improves soil hydrological properties by regulating soil physicochemical properties in the Loess Plateau, China, J. Hydrol., 609, 127730, https://doi.org/10.1016/j.jhydrol.2022.127730, 2022.
Ren, F., Zhou, H. K., Zhao, X. Q., Han, F., Shi, L. N., Duan, J. C., and Zhao, J. Z.: Influence of simulated warming using OTC on physiological–biochemical characteristics of Elymus nutans in alpine meadow on Qinghai-Tibetan plateau, Acta Ecol. Sinica, 30, 166–171, https://doi.org/10.1016/j.chnaes.2010.04.007, 2010.
Robinson, M., Cognard-Plancq, A. L., Cosandey, C., David, J., Durand, P., Führer, H. W., Hall, R., Hendriques, M. O., Marc, V., McCarthy, R., McDonnell, M., Martin, C., Nisbet, T., O'Dea, P., Rodgers, M., and Zollner, A.: Studies of the impact of forests on peak flows and baseflows: a European perspective, Forest Ecol. Manag., 186, 85–97, https://doi.org/10.1016/s0378-1127(03)00238-x, 2003.
Rodrigo-Comino, J., Brevik, E. C., and Cerdà, A.: The age of vines as a controlling factor of soil erosion processes in mediterranean vineyards, Sci. Total Environ., 616–617, 1163–1173, https://doi.org/10.1016/j.scitotenv.2017.10.204, 2018.
Rumynin, V. G.: Surface Runoff Generation, Vertical Infiltration and Subsurface Lateral Flow, in: Overland flow dynamics and solute transport. Theory and Applications of Transport in Porous Media, vol 26, Springer, Cham, https://doi.org/10.1007/978-3-319-21801-4_1, 2015.
Saxton, K. E. and Rawls, W. J.: Soil water characteristic estimates by texture and organic matter for hydrologic solutions, Soil Sci. Soc. Am. J., 70, 1569–1578, https://doi.org/10.2136/sssaj2005.0117, 2006.
Schmidt, S., Alewell, C., and Meusburger, K.: Monthly RUSLE soil erosion risk of Swiss grasslands, J. Maps, 15, 247–256, https://doi.org/10.1080/17445647.2019.1585980, 2019.
Schwarz, M., Rist, A., Cohen, D., Giadrossich, F., Egorov, P., Büttner, D., Stolz, M., and Thormann, J. J.: Root reinforcement of soils under compression, J. Geophys. Res.-Earth, 120, 2103–2120, https://doi.org/10.1002/2015JF003632, 2015.
Shang, Z. H., Ma, Y. S., Long, R. J., and Ding, L. M.: Effect of fencing, artificial seeding and abandonment on vegetation composition and dynamics of `black soil land' in the headwaters of the Yangtze and the Yellow Rivers of the Qinghai-Tibetan Plateau, Land Degrad. Dev., 19, 554–563, https://doi.org/10.1002/ldr.861, 2008.
Shi, X., Zhang, F., Wang, L., Jagirani, M. D., Zeng, C., Xiao, X., and Wang, G. X.: Experimental study on the effects of multiple factors on spring meltwater erosion on an alpine meadow slope, Int. Soil Water Conse., 8, 116–123, https://doi.org/10.1016/j.iswcr.2020.02.001, 2020.
Török, P., Brudvig, L. A., Kollmann, J., Price, J., and Tóthmérész, B.: The present and future of grassland restoration, Restor. Ecol., 29, e13378, https://doi.org/10.1111/rec.13378, 2021.
Wang, B., Zhang, G., Yang, Y., Li, P., and Liu, J.: Response of soil detachment capacity to plant root and soil properties in typical grasslands on the Loess Plateau, Agr. Ecosyst. Environ., 266, 68–75, https://doi.org/10.1016/j.agee.2018.07.016, 2018.
Wang, C. T., Wang, G. X., Liu, W., Wang, Y., Hu, L., and Ma, L.: Effects of establishing an artificial grassland on vegetation characteristics and soil quality in a degraded meadow, Isr. J. Ecol. Evol., 59, 141–153, https://doi.org/10.1080/15659801.2013.863669, 2013a.
Wang, L., Liang, T., and Zhang, Q.: Laboratory experiments of phosphorus loss with surface runoff during simulated rainfall, Environ. Earth Sci., 70, 2839–2846, https://doi.org/10.1007/s12665-013-2344-9, 2013b.
Wang, Z. Q., Zhang, Y. Z., Yang, Y., Zhou, W., Gang, C. C., Zhang, Y., Li, J. L., An, R., Wang, K., Odeh, I., and Qi, J. G.: Quantitative assess the driving forces on the grassland degradation in the Qinghai–Tibet Plateau, in China, Ecol. Inform., 33, 32–44, https://doi.org/10.1016/j.ecoinf.2016.03.006, 2016.
Wen, Y. R., Liu, B., Lin, L. T., Hu, M. M., Wen, X., Li, T. Y., Rong, J. D., and Yao, S. H.: Shelterbelt effects on soil redistribution on an arable slope by wind and water, Catena, 241, 108044, https://doi.org/10.1016/j.catena.2024.108044, 2024.
Wick, A. F., Geaumont, B. A., Sedivec, K. K., and Hendrickson, J.: Grassland degradation, in: Biological and environmental hazards, risks, and disasters, edited by: Shroder, J. F. and Sivanpillai, R., Elsevier, 257–276, https://doi.org/10.1016/B978-0-12-394847-2.00016-4, 2016.
Wu, G. L., Liu, Z. H., Zhang, L., Hu, T., and Chen, J.: Effects of artificial grassland establishment on soil nutrients and carbon properties in a black-soil-type degraded grassland, Plant Soil, 333, 469–479, https://doi.org/10.1007/s11104-010-0363-9, 2010.
Wu, G. L., Huang, Z., Liu, Y. F., Cui, Z., Chang, X. F., Tian, F. P., López-Vicented, M., and Shi, Z. H.: Soil water response of plant functional groups along an artificial legume grassland succession under semi-arid conditions, Agr. Forest Meteorol., 278, 107670, https://doi.org/10.1016/j.agrformet.2019.107670, 2019.
Wu, G. L., Liu, Y. F., Cui, Z., Liu, Y., Shi, Z. H., Yin, R., and Kardol, P.: Trade-off between vegetation type, soil erosion control and surface water in global semi-arid regions: A meta-analysis, J. Appl. Ecol., 57, 875–885.https://doi.org/10.1111/1365-2664.13597, 2020.
Vanacker, V., Molina, A., Rosas, M. A., Bonnesoeur, V., Román-Dañobeytia, F., Ochoa-Tocachi, B. F., and Buytaert, W.: The effect of natural infrastructure on water erosion mitigation in the Andes, SOIL, 8, 133–147, https://doi.org/10.5194/soil-8-133-2022, 2022.
Xia, L., Song, X. Y., Fu, N., Cui, S. Y., Li, L. J., Li, H. Y., and Li, Y. L.: Effects of forest litter cover on hydrological response of hillslopes in the Loess Plateau of China, Catena, 181, 104076, https://doi.org/10.1016/j.catena.2019.104076, 2019.
Xu, J.: A cave δ18O based 1800-year reconstruction of sediment load and streamflow: The Yellow River source area, Catena, 161, 137–147, https://doi.org/10.1016/j.catena.2017.09.028, 2018.
Zhang, G. H., Tang, K. M., Ren, Z. P., and Zhang, X. C.: Impact of grass root mass density on soil detachment capacity by concentrated flow on steep slopes, T. ASABE, 56, 927–934, 2013.
Zhao, X., Huang, J., Wu, P., and Gao, X.: The dynamic effects of pastures and crop on runoff and sediments reduction at loess slopes under simulated rainfall conditions, Catena, 119, 1–7, https://doi.org/10.1016/j.catena.2014.03.001, 2014.
Zhao, Y. T., Pu, Y. F., Lin, H. L., and Tang, R.: Examining soil erosion responses to grassland conversation policy in Three-River Headwaters, China, Sustainability, 13, 2702, https://doi.org/10.3390/su13052702, 2021.
Zhou, J., Fu, B. J., Gao, G. Y., Lü, Y. H., Liu, Y., Lü, N., and Wang, S.: Effects of precipitation and restoration vegetation on soil erosion in a semi-arid environment in the Loess Plateau, China, Catena, 137, 1–11, https://doi.org/10.1016/j.catena.2015.08.015, 2016.
Zhu, P. Z., Zhang, G. H., Wang, H. X., Yang, H. Y., Zhang, B. J., and Wang, L. L.: Effectiveness of typical plant communities in controlling runoff and soil erosion on steep gully slopes on the Loess Plateau of China, J. Hydrol., 602, 126714, https://doi.org/10.1016/j.jhydrol.2021.126714, 2021.