the Creative Commons Attribution 4.0 License.
the Creative Commons Attribution 4.0 License.
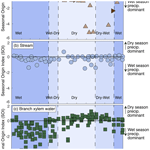
The seasonal origins and ages of water provisioning streams and trees in a tropical montane cloud forest
Gregory R. Goldsmith
Roxanne M. Cruz-de Hoyos
Adan Julian Ccahuana Quispe
A. Joshua West
Determining the sources of water provisioning streams, soils, and vegetation can provide important insights into the water that sustains critical ecosystem functions now and how those functions may be expected to respond given projected changes in the global hydrologic cycle. We developed multi-year time series of water isotope ratios (δ18O and δ2H) based on twice-monthly collections of precipitation, lysimeter, and tree branch xylem waters from a seasonally dry tropical montane cloud forest in the southeastern Andes mountains of Peru. We then used this information to determine indices of the seasonal origins, the young water fractions (Fyw), and the new water fractions (Fnew) of soil, stream, and tree water. There was no evidence for intra-annual variation in the seasonal origins of stream water and lysimeter water from 1 m depth, both of which were predominantly comprised of wet-season precipitation even during the dry seasons. However, branch xylem waters demonstrated an intra-annual shift in seasonal origin: xylem waters were comprised of wet-season precipitation during the wet season and dry-season precipitation during the dry season. The young water fractions of lysimeter (< 15 %) and stream (5 %) waters were lower than the young water fraction (37 %) in branch xylem waters. The new water fraction (an indicator of water ≤ 2 weeks old in this study) was estimated to be 12 % for branch xylem waters, while there was no significant evidence for new water in stream or lysimeter waters from 1 m depth. Our results indicate that the source of water for trees in this system varied seasonally, such that recent precipitation may be more immediately taken up by shallow tree roots. In comparison, the source of water for soils and streams did not vary seasonally, such that precipitation may mix and reside in soils and take longer to transit into the stream. Our insights into the seasonal origins and ages of water in soils, streams, and vegetation in this humid tropical montane cloud forest add to understanding of the mechanisms that govern the partitioning of water moving through different ecosystems.
- Article
(4596 KB) - Full-text XML
-
Supplement
(12181 KB) - BibTeX
- EndNote
Observations that the sources of precipitation that supply streamflow are not necessarily the same as the sources of precipitation that provision evapotranspiration (Brooks et al., 2010; Dawson and Ehleringer, 1991; Evaristo et al., 2015) raise questions about how precipitation entering an ecosystem will be partitioned into different fluxes over time (Kirchner and Allen, 2020). One interesting implication of such observations is that the origins and ages of water in these fluxes differ from one another and that these differences are likely to arise from variation induced by the transport, mixing, and storage of water in the subsurface (Berghuijs and Allen, 2019; Berghuijs and Kirchner, 2017). Measuring the time it takes for water to transit through ecosystems can thus provide fundamental insights into ecohydrological processes, such as the flood response of streams when there is significant precipitation (Gallart et al., 2020) or the drought response of plants when there is minimal precipitation (Harpold, 2016; Sprenger et al., 2022b).
Much of what we know about the origins and ages of pools and fluxes of water in ecosystems originates from a long history of observations of the ages of water that sustain streamflow, particularly in relation to discrete precipitation events (Kirchner et al., 2023; Sprenger et al., 2019). Such research has consistently demonstrated that streamflow is comprised of waters that have been stored and mixed over long time periods in the subsurface rather than waters from recent storms (McGuire and McDonnell, 2006). Nevertheless, stream water is generally known to range in age from days to decades (Jasechko, 2019; Sprenger et al., 2019). More recently, observations of the ages of transpiration waters have demonstrated that the water taken up by trees can originate from sources that are several months old (Allen et al., 2019; Miguez-Macho and Fan, 2021). However, most of this information has come from comparatively dry seasonal climates (Berkelhammer et al., 2020; Brinkmann et al., 2018; Ehleringer et al., 1991; Hu et al., 2010; Kerhoulas et al., 2013; Martin et al., 2018), and there is evidence that total precipitation amount plays a role in the seasonal origin, or age, of water taken up by plants (Goldsmith et al., 2022). Even rarer are empirical studies that simultaneously measure ages of water provisioning streams and plants at the same location (Dai et al., 2022), limiting our ability to build a complete understanding of ecosystem hydrology.
Stable isotopes of water (δ18O and δ2H) provide a means of estimating the origins and ages of water that provision trees and streamflow, and exciting new data analysis approaches now support more robust estimates (Benettin et al., 2022). Three of these approaches are of particular interest. First, comparing the isotopic signature of water in streams or plants to the isotopic signature of precipitation falling in the wet or dry season can facilitate the study of the seasonal origins of water in streamflow and transpiration (the seasonal origin index sensu Allen et al., 2019). One benefit of the seasonal origin index is that it can be calculated for relatively short time intervals, facilitating the examination of how seasonal origins of water leaving an ecosystem may change over time. Second, the amplitude of the precipitation isotope intra-annual cycle can be compared to the amplitude of the soil, stream, and plant branch xylem water isotope intra-annual cycles to understand the relative amounts of recent precipitation sustaining these pools and fluxes of water (the young water fraction sensu Kirchner, 2016). The young water fraction thus provides an approach to estimating the age of water in streams and taken up by plants. Third, a modified hydrograph separation framework can also be applied to stream and plant water, similar to techniques used for decades to understand the contribution of “new” water to streams during storms (e.g., Hooper et al., 1990). In this ensemble hydrograph separation approach, mixing end-members change with each sampling time step to include the previous sample as the “old” water and the precipitation that fell in between as the “new” water (Kirchner, 2019). The ensemble of hydrograph separations is then used to calculate a representative new water fraction or the fraction of water contained within streamflow or plant water that fell as precipitation from the previous sample. Taken together, the seasonal origin index (order of 3–6 months), young water fraction (order of ≤ 2 months), and new water fraction (order of 2 weeks) provide complementary approaches to estimating the origins and ages of water. When these approaches are applied to soils, streams, and plants simultaneously, they can provide insights into the partitioning of water within an ecosystem.
We studied the seasonal origins and ages of water within a tropical montane cloud forested watershed and used these results to address whether streamflow and transpiration are supplied by the same sources of water. While there has been considerable research on the origins and ages of soil water and streamflow, comparatively little is known about plants. Information about these multiple hydrological compartments from the same site can provide a more comprehensive understanding of how water flows through an ecosystem but requires significant effort to collect all the necessary data over time. In this study, we collected soil lysimeter (1 m depth), stream, and tree branch xylem waters approximately twice monthly over multiple years and used these data to estimate the seasonal origin index, the young water fractions, and the new water fractions of each compartment. In this seasonal wet tropical montane environment, we expected shallow rooted trees to take up relatively young water and expected streamflow to be comprised of relatively older water because of the permeable, fractured shale bedrock that increases the time it takes for precipitation to move through the watershed (Burt et al., 2023a).
2.1 Study site
This study was carried out in a tropical montane cloud forest within a small (0.33 km2), mountainous (mean elevation 3271 m, mean slope 33.8∘) watershed located in the Amazon Conservation Association's Wayqecha reserve in the southeastern Peruvian Andes (−13.19255∘, −71.58795∘; Fig. 1). The dominant lithology underlying the catchment is the San José Group shale (Carlotto et al., 1996), and the soils are classified as Umbrisols (Asner et al., 2017) with an organic layer depth of ∼ 20–26 cm (Girardin et al., 2010a; Wu et al., 2019). Three of the dominant plant genera are Weinmannia (16 % of woody stems), Clusia (13 % of woody stems), and Miconia (8 % of woody stems) (Rapp et al., 2012), and the leaf area index is 3.73 ± 0.14 m2 m−2 (Girardin et al., 2014). Mean annual precipitation, recorded via a tipping-bucket rain gauge (TB3; Hydrological Services Pty Ltd; Liverpool, NSW, Australia; accuracy ±2 %), was 4110 ± 418 mm measured from 2016 to 2021. The rainfall regime is characterized by a pronounced wet season from December to March, during which approximately 65 % of the annual precipitation occurs. The mean annual temperature is ∼ 11 ± 1∘C (Rapp and Silman, 2012). Previous studies in this tropical montane cloud forest have used stable isotopes of water to establish paleohydrologic proxies (Feakins et al., 2016) and as tools for fingerprinting organic carbon sources (Feakins et al., 2018; Ponton et al., 2014). Other past work at this site used stable isotopes of water to create a hydrologic budget for a mesoscale (161 km2) catchment (Clark et al., 2014), of which the small watershed studied here (0.33 km2) sits within the headwaters.
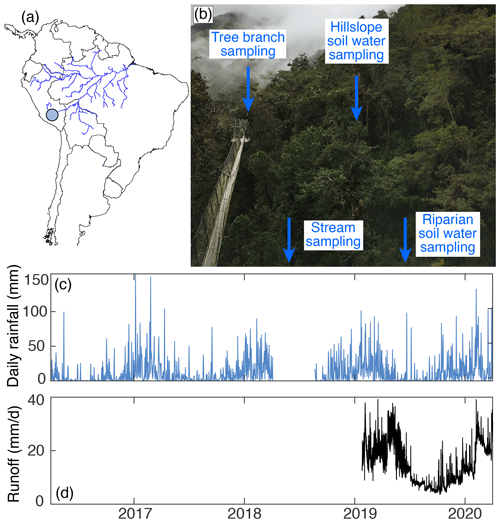
Figure 1(a) Map of South America: the blue circle represents the approximate location of the tropical montane cloud forested watershed studied in this paper. (b) Image of the study site: tree branch xylem samples were collected from the canopy platform visible in this photo. The stream and riparian lysimeters are located in the valley bottom beneath the canopy bridge (Fig. S1 in the Supplement). (c) Daily rainfall from 2016 to 2020. (d) Instantaneous stream runoff from 2019 to 2020.
2.2 Sample collection
From February 2018 to March 2020 we sampled tree branches two times per month. During each sampling, we used a canopy bridge (Fig. 1b) to access the crown of mature trees and collected one branch from one individual tree for three different species: Weinmannia bangii Rusby, Weinmannia reticulata Ruiz & Pav., and Clusia alata Planch. & Triana. Rather than sample the same three individual trees twice a month (which would quickly deplete available branches), we collected samples from a group of trees within ∼ 100 m of the stream we sampled (described below). Each branch sample was collected by cutting a mature branch sample > 10 cm away from the leaves and removing the bark. We then sealed the branch xylem samples into vials and immediately wrapped them with parafilm. The samples were frozen upon return from the field (< 24 h) and kept frozen until water extraction.
From April 2016 to March 2020, we collected stream water, soil lysimeter water, and precipitation samples twice per month. Stream water samples were filtered with a 0.2 µm diameter polyethersulfone filter in the field and sealed in glass vials with PolyCone cap liners. Soil water samples were collected from two suction lysimeters installed approximately 1 m below the soil surface: one in the valley bottom, near the stream riparian zone, and the other on the hillslope, near the tree sampling location (see Fig. 1b). The water in the lysimeters may thus reflect an integration of a 2-week period: the lysimeters were evacuated after each sampling and left under tension (−60 to −70 kPa) until the subsequent sampling, when the water was then collected. Upon collection, lysimeter waters were filtered with a 0.2 µm diameter polyethersulfone filter in the field and sealed in glass vials with PolyCone cap liners. Precipitation samples were also collected over 2-week time periods in a bucket with a layer of vegetable oil to prevent evaporation loss. On retrieval, precipitation samples were sealed in glass vials with PolyCone cap liners, and a sample from underneath the oil layer was subsequently filtered with a 0.2 µm diameter polyethersulfone filter in the lab to remove particulates. Precipitation was recorded using a tipping-bucket gauge maintained by the Andes Biodiversity and Ecosystem Research Group (Fig. 1c). Stream water level was logged every 15 min (WL16U Water Level Logger; Global Water; College Station, TX, USA; accuracy ± 0.2%) beginning in January 2019 and through the end of the study (Fig. 1d).
2.3 Stable isotope measurements
Water from branch xylem samples was extracted via cryogenic vacuum distillation at the Center for Stable Isotope Biogeochemistry at UC Berkeley. Each branch xylem sample was placed under a vacuum of ≤ 60 mT and submerged in boiling water for at least 70 min while the water vapor collected in a liquid nitrogen cold trap. Several studies have reported artifacts associated with processing and analyzing samples for stable isotopes of water (von Freyberg et al., 2020), particularly with respect to cryogenic vacuum extraction (Chen et al., 2020). Importantly, recent experiments suggest that the total volume of water extracted from the plant sample is related to the magnitude of isotopic biases, such that extracting > 600 µL of water minimizes methodological biases (Diao et al., 2022). Here, the volume of extraction water was ≥ 600 µL for most samples. To assess the completeness of the branch xylem water extraction, post-extraction branches were weighed, dried for 72 h, and reweighed. The post-extraction drying showed that cryogenic extraction resulted in the recovery of > 90 % of the branch xylem water in nearly all the branch samples (data not shown). The extracted branch waters were analyzed for δ18O (continuous flow using a Thermo Gas Bench II connected to a Thermo Delta V Plus Mass Spectrometer) and δ2H (dual inlet using a hot chromium reactor unit (H/Device) connected to a Thermo Delta V Plus Mass Spectrometer) at the Berkeley Center for Stable Isotope Biogeochemistry. The standard deviations of an independent quality-control sample were 0.1 ‰ or better for δ18O and 1.1 ‰ for δ2H.
Lysimeter, stream, and precipitation waters were analyzed for δ18O and δ2H via isotope ratio infrared spectroscopy using a Picarro L2130i (Chapman University) and two Los Gatos Research Liquid Water Isotope Analyzers (LGR) (Caltech and Lawrence Berkeley National Lab). The Chapman University Picarro and the Lawrence Berkeley National Lab LGR measured an independent quality-control standard at least five times per run, and the Caltech LGR measured reference materials after every five samples but did not use a quality-control sample. For the Picarro, the standard deviations of an independent quality-control sample were 0.1 ‰ for δ18O and 0.5 ‰ for δ2H. For the LGR at Lawrence Berkeley National Lab, the standard deviations of an independent quality-control sample across the runs were 0.1 ‰ for δ18O and 0.5 ‰ for δ2H. All the values are represented in per mil relative to Vienna Standard Mean Ocean Water (VSMOW), and all the data were corrected using standard practices accounting for calibration, memory, and drift correction.
Oxygen and hydrogen isotopes in precipitation display a strong relationship with elevation in this region (−1.7 ‰ in δ18O km−1 elevation and −12 ‰ in δ2H km−1 elevation; Burt et al., 2023a, b). Given that precipitation samples were collected at ∼ 2900 m and that the watershed elevation ranges from ∼ 2900 to 3500 m, we accounted for the effects of elevation on precipitation isotopes within the watershed by correcting the oxygen and hydrogen isotope values to a mean elevation of 3200 m.
2.4 Stable isotope data analysis
To account for the isotopic effects of evaporation, branch xylem water isotopes (δ18O and δ2H) were corrected back to their source waters using the method described in Benettin et al. (2018). Water isotope evaporation lines were calculated for each month using monthly average relative humidity and temperature (based on 3 years of unpublished data). The evaporation-corrected branch xylem water isotope and uncorrected soil lysimeter, stream, and precipitation isotope values were used to calculate an index of the seasonal origin of water and the young water fraction in branch xylem, soil, and stream waters.
To calculate an index of the seasonal origin of water in different pools and fluxes, we followed Allen et al. (2019):
where δx is the oxygen isotope composition of individual stream, lysimeter, or tree water samples; δannP is the volume-weighted annual precipitation oxygen isotope composition; δdryP is the average dry-season precipitation oxygen isotope composition; and δwetP is the average wet-season precipitation oxygen isotope composition. A seasonal origin index (SOI) near −1.0 indicates a sample of stream, lysimeter, or tree water mostly comprised of wet-season precipitation; a value of +1.0 indicates a sample of stream, lysimeter, or tree water mostly comprised of dry-season precipitation. Given the high variation in the isotopic composition of precipitation, individual xylem water samples responding to a given precipitation event may fall outside of the average seasonal precipitation end-members, resulting in an SOI greater than +1.0 or less than −1.0.
The young water fraction requires estimation of the amplitude of the seasonal differences in water isotopes. To do so, we fit a sinusoidal function to precipitation, lysimeter and stream water, as well as evaporation-corrected branch xylem water isotope values to characterize the seasonal cycle in oxygen isotopes (Allen et al., 2018):
where δ18O(t) is the oxygen isotope ratio of precipitation, lysimeter, stream, or tree water at a given time; f is the frequency of the oxygen isotope seasonal cycle in each reservoir (fixed to 1 year); a and b are the cosine and sine coefficients; and k is the isotopic offset. The fit of Eq. (3) to stream water and precipitation δ18O was flow-weighted by stream discharge and precipitation amount, respectively. Coefficients a and b from Eq. (3) were then used to calculate the δ18O seasonal cycle amplitude (A) for precipitation, lysimeter, stream and branch xylem water as follows:
where x is the amplitude for the different pools or fluxes of water: lysimeter, stream, or branch xylem water.
To calculate the young water fractions (Fyw) of lysimeter, stream, or branch xylem waters, we followed Kirchner (2016a, b):
where A is as defined by Eqs. (4) and (5) above.
To assess the uncertainty of the young water fraction estimates, we carried out a bootstrapped resampling of the precipitation, lysimeter, stream, and branch xylem water δ18O datasets. To resample each dataset, we drew one sample at random from the complete dataset and then repeated this resampling with replacement until we had drawn the same number of random samples as the original dataset (e.g., for the branch xylem water δ18O dataset with 142 observations, we randomly sampled 142 times, each time from the full dataset of tree water δ18O values). We repeated this 10 000 times each for the precipitation, stream, and tree water δ18O datasets. We then calculated Eqs. (3)–(6) for each of those 10 000 bootstrapped datasets. This allowed us to constrain the young water fraction as a distribution of values for the stream and tree water.
To calculate the new water fraction, the fraction of stream or branch xylem water comprised of precipitation that has fallen since the previous sampling (Kirchner, 2019), we applied an ensemble hydrograph separation framework to the stream and tree water δ18O datasets. In our study, the average time step between samples was 15 ± 6 d for the riparian and hillslope soil lysimeter waters, 17 ± 5 d for stream water, and 16 ± 5 d for branch xylem water. Therefore, the new water fractions represent the fraction of precipitation within each pool or flux of water that fell within the preceding ∼ 2 weeks (15–17 d). To determine new water fractions, we first calculated
where δ18Op,j is the oxygen isotope composition of precipitation at a given sampling time point j and is the oxygen isotope composition of stream or tree water at the previous sampling time point, j−1. We then calculated
where δ18Ox,j is the oxygen isotope composition of stream or tree water at a given sampling time point. We calculated Eqs. (7) and (8) for all the stream and branch xylem water sampling time points and created a scatterplot with the results of Eq. (7) on the x axis and Eq. (8) on the y axis. The new water fraction is equivalent to the slope of the regression line between x and y. Finally, we performed analysis of covariance (ANCOVA) to evaluate the relationship between δ18Op,j–δ18O and δ18Ox,j–δ18O for lysimeter, stream, and branch xylem water, with season as a covariate.
3.1 Stable oxygen and hydrogen isotopes of water
Precipitation isotope ratios ranged from −20.1 ‰ to −0.4 ‰ for δ18O and from −153 ‰ to 18 ‰ for δ2H and were used to calculate the local meteoric water line (LMWL), which had a slope of 8.49 and an intercept of 19.29 (Fig. 2). Given that precipitation was collected approximately 300 m below the mean watershed elevation, we applied an isotopic correction to account for the orographic effects (see “Materials and methods”). Precipitation isotope ratios ranged from −20.6 ‰ to −0.9 ‰ for δ18O and from −157 ‰ to 14 ‰ for δ2H after the orographic correction was applied. Stream water isotope ratios ranged from −14.5 ‰ to −10.5 ‰ for δ18O and from −97 ‰ to −80 ‰ for δ2H. Most stream water samples fell along the LMWL, and only a few samples had a greater deuterium excess relative to the LMWL. The small deviation from the LMWL suggests minimal contribution of cloud water to the stream in this study, since cloud water vapor at this site has a greater deuterium excess relative to the LMWL (Clark et al., 2014). In contrast, in their study of the mesoscale 161 km2 watershed, Clark et al. (2014) calculated that approximately 10 % of stream water originated from cloud water. Soil water isotope ratios in this study ranged from −18.0 ‰ to −7.9 ‰ for δ18O and from −129 ‰ to −47 ‰ for δ2H. Plant water isotope ratios ranged from −14.8 ‰ to −4.6 ‰ for δ18O and from −114 ‰ to −37 ‰ for δ2H. Plant water isotope ratios generally had a lower deuterium excess relative to the LMWL. Correction of plant water isotope ratios to account for evaporative enrichment yielded isotope ratios that ranged from −16.7 ‰ to −8.1 ‰ for δ18O and from −122 ‰ to −49 ‰ for δ2H (Fig. S2). We used the evaporation-corrected plant water isotope values for the seasonal origin index calculation and the young and new water fraction calculations.
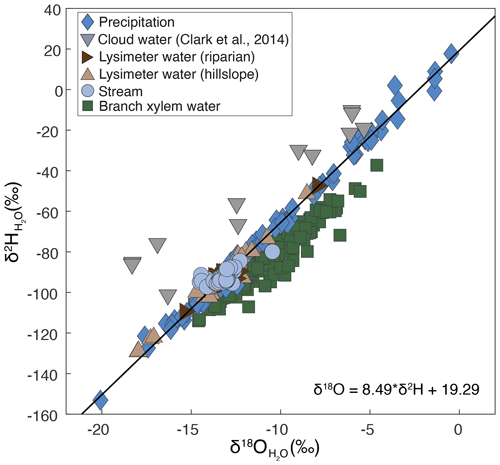
Figure 2Precipitation, stream, soil, and plant water isotope ratios collected between 2016 and 2020 from a tropical montane cloud forested watershed in the southeastern Peruvian Andes mountains. The regression line and the accompanying equation represent the local meteoric water line. Cloud water isotope ratios are from Clark et al. (2014). Lysimeter water was collected from two lysimeters, one in the stream riparian area (dark-brown sideways-facing triangles) and one on the hillslope (light-brown upwards-facing triangles).
Precipitation δ18O demonstrated a strong seasonal cycle (Fig. 3a), with heavier δ18O values during dry seasons (May–September) and lighter δ18O values during wet seasons (December–March). In contrast, lysimeter and stream waters (Fig. 3b, c) demonstrated minimal seasonal variability in δ18O. Tree branch xylem water δ18O (Fig. 3d) followed the precipitation isotope seasonal cycle more closely than stream or lysimeter water but was still attenuated compared to precipitation. The estimated amplitude of the δ18O seasonal cycle was 4.2 ± 0.5 ‰ (fit ±1 SE) for precipitation, 0.7 ± 0.3 ‰ for hillslope lysimeter water, 0.3 ± 0.1 ‰ for riparian lysimeter water, 0.2 ± 0.2 ‰ for stream water, and 1.6 ± 0.1 ‰ for branch xylem water.
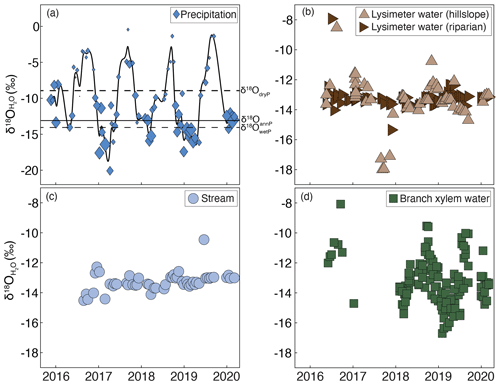
Figure 3δ18O of (a) precipitation, with the size of the marker representing the relative amount of precipitation that fell in the 2 weeks prior to sample collection and the solid line showing a smooth spline fit; (b) lysimeter waters; (c) stream water; and (d) branch xylem water from a tropical montane cloud forested watershed in the southeastern Peruvian Andes mountains.
3.2 Seasonal origin indices
We calculated the intra-annual SOI of lysimeter, stream, and tree waters (Fig. 4). Lysimeter and stream water SOI values were consistently around zero or negative (Fig. 4a, b). Neither lysimeter nor stream waters demonstrate intra-annual variation in SOI. Stream and lysimeter water SOI values both consistently indicate an origin from wet-season precipitation, even during the dry season (Fig. 4a, b). Branch xylem water SOI varied intra-annually, with a more negative SOI during the wet season and wet–dry transition and a more positive SOI during the dry season and dry–wet transition (Fig. 4c). To determine whether different plant species use waters from different seasonal origins, we compared the SOI of different plant species collected on the same dates and found no evidence for systematic differences (Fig. S3).
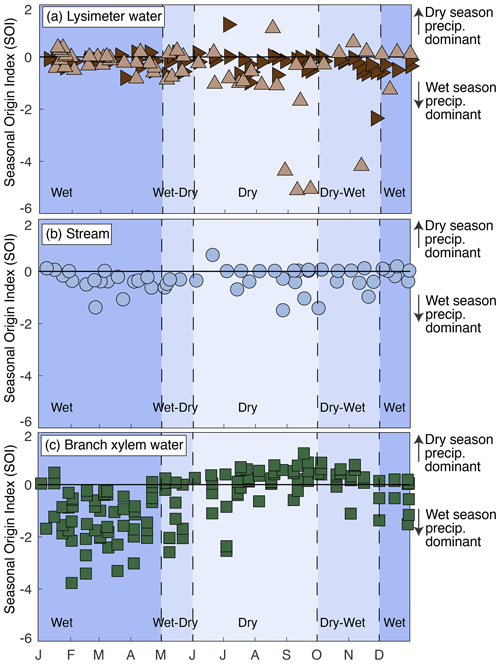
Figure 4Intra-annual patterns of the seasonal origins of lysimeter, stream, and branch xylem waters from the years 2016 to 2020 in a tropical montane cloud forested watershed in the southeastern Peruvian Andes mountains. Lysimeter water was collected from two lysimeters, one in the stream riparian area (dark-brown sideways-facing triangles) and one on the hillslope (light-brown upwards-facing triangles). The x axis shows months. Blue shading indicates the transitions between seasons.
3.3 Young water fractions
Young water fractions (Fyw) were calculated for lysimeter, stream, and branch xylem waters (Fig. 5). The lysimeter water Fyw median values and their Gaussian-propagated standard errors were 15 ± 43 % (hillslope lysimeter) and 6 ± 47 % (riparian lysimeter). The stream water Fyw was 5 ± 92 %. The high standard error of the stream water Fyw is a result of the very low amplitude of the seasonal cycle in δ18O. The branch xylem water Fyw was 37 ± 15 %. Thus branch xylem water had a greater fraction of young water than found in stream water and the hillslope and riparian lysimeters.
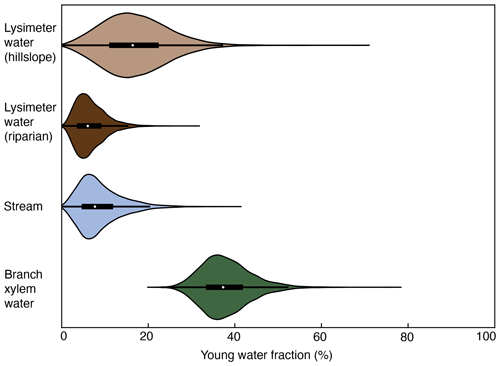
Figure 5Violin and inset boxplots of the young water fractions (Fyw) for lysimeter, stream, and branch xylem water, as determined from a bootstrap resampling routine. The Fyw interquartile range determined from the bootstrap resampling routine was 12 %–22 % for the hillslope lysimeter water, 4 %–9 % for the riparian water, 5 %–11 % for the stream water, and 34 %–41 % for the branch xylem water. Fyw values were calculated using data on isotopic composition of waters from the years 2016 to 2020 in a tropical montane cloud forested watershed in the southeastern Peruvian Andes mountains.
3.4 New water fractions
We calculated new water fractions (Fnew) for soil lysimeter (on the hillslope and in the riparian zone), stream, and branch xylem waters. To determine Fnew, we used the slope of the linear regression of data in Fig. 6.
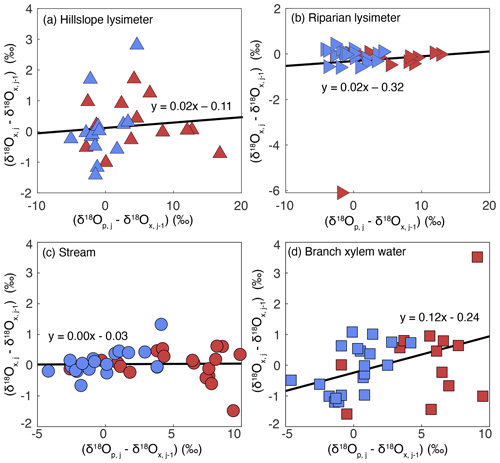
Figure 6Ensemble hydrograph separation performed on lysimeter waters (a, b), stream waters (c), and tree branch xylem waters (d) in a tropical montane cloud forested watershed in the southeastern Peruvian Andes mountains. The x axis is the δ18O of precipitation at a given time step minus the stream or xylem δ18O at the previous time step. The y axis is the stream or xylem δ18O at a given time step minus the stream or xylem δ18O at the previous time step. The slopes of the regression line through the stream and branch xylem water datasets are equivalent to their respective new water fractions. Blue symbols indicate stream and branch xylem water samples collected during the wet season, while red symbols indicate samples collected during the dry season. ANCOVA indicated no significant differences between the wet and dry seasons.
There is no evidence for a significant relationship between δ18Op,j–δ18O and δ18Ox,j–δ18O for hillslope soil lysimeter water ( 0.11, p= 0.7) or riparian soil lysimeter water ( 2.67, p= 0.1) as determined by ANCOVA. This result indicates that there is no evidence for hillslope and riparian soil water that originated from precipitation that fell within the ∼ 2 weeks prior to sample collection (Fnew = 0 %). There was no evidence for a significant relationship between δ18Op,j–δ18O and δ18Ox,j–δ18O for stream water ( 0.7, p= 0.4), nor was there evidence for differences between seasons ( 1.1, p= 0.3), such that the stream Fnew was 0 %. This indicates that, on average, no measurable streamflow originated from precipitation that fell within the ∼ 2 weeks prior to sample collection. Accounting for stream discharge in the Fnew calculations does not change the Fnew,stream (Fig. S5). There was evidence for a significant relationship between δ18Op,j–δ18O and δ18Ox,j–δ18O for branch xylem water ( 8.1, p= 0.008); however, there was no evidence for differences between seasons (f1,31 = 1.3, p= 0.3). This indicates that, on average, 12 % of the branch xylem water originated from precipitation that fell within the ∼ 2 weeks prior to sample collection.
By developing a multi-year time series of precipitation, lysimeter, stream, and branch xylem water isotopes, we were able to estimate the seasonal origins of water in key pools and fluxes of the hydrological cycle as well as to generate two different estimates of the proportion of water in these pools and fluxes originating from recent precipitation. Our results demonstrate that lysimeter and stream waters were predominantly comprised of wet-season precipitation, even during dry seasons. However, branch xylem waters demonstrated intra-annual variation in their seasonal origins: xylem waters were comprised of wet-season precipitation during the wet season and dry-season precipitation during the dry season. In contrast with previous studies focusing on the spatial variation in the seasonal origins of water in soils, streams, and trees (Allen et al., 2019; Goldsmith et al., 2022; Martin et al., 2018), our results make use of high-frequency sampling to provide insights into temporal variation. Moreover, lysimeter and stream waters displayed low young and new water fractions compared to branch xylem waters, indicating that plants take up water that is younger than is found in other pools and fluxes. Though theoretical predictions lead us to expect water stored within ecosystems to have different ages than water flowing out of ecosystems, the water taken up by plants is expected to be older than water sustaining streamflow (e.g., Berghuijs and Allen, 2019). Here, we find that water taken up by plants is younger than water sustaining streamflow. The reasons for this difference merit further consideration. Coupling estimates of the seasonal origin index with the young and new water fractions can contribute to resolving the ages of waters transiting through terrestrial ecosystems.
4.1 Seasonal origins of water in trees and streams and implications for plant rooting depth
The SOI is a measure of the season from which the water in a particular pool or flux originated (Allen et al., 2019). For example, values approaching −1.0 indicate that the sample is mostly comprised of wet-season precipitation. In this study, lysimeter and stream waters display little intra-annual variability in SOIs, and the values estimated were nearly always ≤ 0, indicating that these hydrologic compartments were consistently supplied over time by a source of water predominantly comprised of wet-season precipitation. The hillslope and riparian lysimeters display similar SOIs, though the hillslope lysimeter SOI varies slightly more than the riparian lysimeter SOI. One possible explanation for the lack of variation in the riparian lysimeter SOI could be buffering of the water supply by hyporheic exchange.
Soil water lysimeters provide an incomplete picture of soil water isotope dynamics. We expect lysimeter water to be held at a higher (less negative) matric potential and therefore to sample a pool of more mobile water than bulk soil water (Goldsmith et al., 2012). However, the lack of intra-annual variability in the lysimeter water SOI suggests that the lysimeters either do not sample pools of mobile water or simply do not sample a significant amount of recent precipitation. The lack of a recent precipitation signal in lysimeter waters could be due to recent precipitation rapidly mixing with large amounts of existing soil water that dilutes the signal, significant soil water mixing at the 1 m depth of sampling (consistent with observations from other tropical Andean watersheds; Lahuatte et al., 2022), or the fact that lysimeter waters were collected over a period of 2 weeks, leading to some temporal averaging in collection alone.
There were differences in the SOI (and δ18O) of the two lysimeters based on their geomorphic position: the lysimeter on the hillslope (Fig. 3; upwards-facing triangles) had a more variable SOI than the lysimeter in the riparian zone (Fig. 3; sideways-facing triangles). In January 2017, we sampled lysimeter and precipitation waters every 4 h and analyzed δ18O (Fig. S4), which provided insight to differences between the two lysimeters. On short timescales, precipitation δ18O was variable. The hillslope lysimeter displayed variable δ18O in response to heterogeneous δ18Oprecip, while the riparian lysimeter remained relatively constant over several days. This could potentially be due to extensive mixing of waters in the riparian zone and comparatively less mixing of waters along hillslopes. The differences in the SOI (and δ18O) between lysimeters on the hillslope and riparian zone may also be due to greater soil water storage at the valley bottom than on the hillslope, similar to what is observed in Andean páramo wetlands (Lazo et al., 2019). Despite the somewhat more variable δ18O in the hillslope lysimeter, it is worth nothing that the hillslope and riparian lysimeter both still exhibit significant dampening of the intra-annual precipitation isotope signal (Fig. 3).
Branch xylem water, in contrast, displayed marked intra-annual variability in the SOI. During the wet seasons, the SOI of branch water was highly variable, but was consistently estimated as < 0. Over the course of the transition to the dry season, SOI became less variable and was consistently estimated as > 0. Thus, the results indicate that trees consistently took up precipitation originating from the same season. The contrasting intra-annual trends in SOI between lysimeter, stream, and plant waters emphasize the differences in temporal origins of water that provision streams and trees.
The seasonally changing sources of plant waters observed in this wet environment are in contrast to observations from dry and temperate ecosystems, which is important for understanding the role of stored water in sustaining plants on a global scale. Previous studies of temperate ecosystems, largely carried out at a single time point, have observed that plants do not appear to use summer precipitation during seasonally-dry summers and instead rely on stored winter precipitation (Allen et al., 2019; Berkelhammer et al., 2020; Martin et al., 2018). Moreover, other studies in dry settings demonstrate that plants use groundwater (Feakins and Sessions, 2010; McCormick et al., 2021; Rempe and Dietrich, 2018), with some positing that water taken up by trees in extremely dry environments may have been in aquifers for decades (Zhang et al., 2017). However, recent modeling results suggest that the use of stored water by plants is overestimated globally due to the overrepresentation of temperate regions in isotope-based ecohydrology studies (Miguez-Macho and Fan, 2021). Moreover, even within a seasonally dry temperate ecosystem, Goldsmith et al. (2022) observed that increasing site mean annual precipitation was significantly related to higher use of summer precipitation during summer (i.e., intra-annual changes in sources of water taken up by plants), a result generally consistent with the pattern we observe in this wet tropical ecosystem. These results emphasize the influence of hydroclimate on sources of water taken up by plants: in wet environments, plant water sources change intra-annually, while in dry and temperate environments, plants rely on winter or wet-season precipitation year-round.
Shallow root systems provide one possible explanation for the changing seasonal origins of plant water in wet environments. In the tropical montane forested watershed studied here, the bedrock is well-draining and the climate is seasonally wet: two factors that are known to lead to shallow roots (Fan et al., 2017) and facilitate the uptake of recent precipitation by plants. Though we do not have detailed measurements of rooting depth, Girardin et al. (2013) found that most roots were contained in the soil organic layer, which is approximately 20–26 cm deep (Girardin et al., 2010b; Wu et al., 2019). Given that precipitation outpaces actual evapotranspiration (Clark et al., 2014) even in the driest months (June-September), there is a consistent supply of recent precipitation that may lessen the demand for plants to root deeper to access stored water. Although the species in this study are capable of foliar water uptake via leaf wetting (Goldsmith et al., 2017b), this is unlikely to be a significant water source, particularly given that trees are unlikely to experience significant water deficits in this humid ecosystem (Goldsmith et al., 2017a). We infer that plants use a significant portion of water from shallow soils (e.g., < 30 cm), which would be consistent with observations of shallow tree rooting depths from wet tropical forests (Jackson et al., 1995; Goldsmith et al., 2012; Sohel et al., 2021; Evaristo et al., 2016). Plants using water from shallow soils may explain the lack of recent precipitation signal captured in the lysimeters at 1 m depth.
4.2 Water ages in ecosystems
The ages of water taken up by plants are largely unknown (Evaristo et al., 2019; Sprenger et al., 2019) but are crucial for understanding how water moves through ecosystems. Previous studies have focused on determining the depth of water taken up by plants (Ehleringer and Dawson, 1992; Evaristo and McDonnell, 2017), potential differences in sources of water used by co-occurring plant species (Guo et al., 2018), or the environmental controls on plant water sources (Allen et al., 2019). Streamflow in mountainous watersheds is often dominated by old water with damped intra-annual δ18O cycles (Jasechko et al., 2016; Lutz et al., 2018; Muñoz-Villers and McDonnell, 2012), yet we have very few estimates of how the age of water taken up by plants compares to water in streams (Dai et al., 2022). An emphasis on the temporal sampling of branch xylem waters allowed us to estimate that the young water fraction (Fyw, water that fell as precipitation within the prior ∼ 2 months) and new water fraction (Fnew, water that fell as precipitation within the prior ∼ 2 weeks) of trees (Fyw= 37 %; Fnew= 12 %) was greater than that of streams (Fyw= 5 %; Fnew= 0 %; Fig. 5). This result is consistent with intra-annual patterns in the branch xylem water SOI, which indicates that trees took up water that fell as precipitation within the same season (Figs. 3 and 4). The low Fyw and Fnew of soil lysimeter water on the hillslope (Fyw= 15 %, Fnew= 0 %) and in the riparian zone (Fyw= 6 %, Fnew= 0 %) highlight the significant transit time for water to infiltrate to the 1 m collection depth. The low lysimeter water Fyw and relatively higher plant xylem water Fyw lend additional support to a working hypothesis that plants in this tropical montane cloud forest utilize shallow root networks. Overall, the significantly different Fyw in the pools and fluxes of water provide insights into how water moves through ecosystems.
One possible explanation for the differing Fyw and Fnew between stream and branch xylem water could be differing storage sizes for the reservoirs that sustain each flux. Transit times are equivalent to the ratio of the storage volume to the flow rate through the storage: given equal flow rates, larger storage reservoirs should lead to older water ages and vice versa (Botter et al., 2010). Previous work in this tropical montane cloud forested watershed has pointed to permeable bedrock, which may be indicative of a large groundwater storage reservoir as one reason for the low Fyw in stream water (Burt et al., 2023a). Other studies have observed old stream water ages in watersheds with permeable bedrock (Hale and McDonnell, 2016) and found that large groundwater storage reservoirs provide a mechanistic explanation for the old water in streams (Hale et al., 2016; Kirchner et al., 2023). Here, water taken up by plants from the shallow soil (as discussed above) is contained within a smaller storage volume than groundwater, leading to a faster transit time of water through the reservoir that is consistent with the high Fyw and Fnew observed in plant waters.
Additionally, we did not find significant differences in Fnew for stream or branch xylem water between wet and dry seasons (Fig. 6) or when discharge-weighting the Fnew,stream (Fig. S5), suggesting that seasonal change in wetness (and the potential influence on watershed storage) does not strongly influence the age of water as it does in some other systems (Harman, 2015; Kuppel et al., 2020). The combination of plant and stream water ages has provided useful information about how water moves through this tropical montane cloud forested ecosystem; future studies including isotope-based estimates of stream and plant water ages would provide exciting avenues for further understanding of ecosystem hydrology.
4.3 The fate of precipitation in ecosystems
Understanding how precipitation is partitioned between streams and plants over space and time remains an open question in ecohydrology; however, combining results from different theoretical, empirical, and modeling approaches can contribute to our more general understanding. More generally, recent research has demonstrated that precipitation from different seasons is not always evenly partitioned between streamflow and evapotranspiration (Kirchner and Allen, 2020; Sprenger et al., 2022). Differences in sources of water that sustain streamflow and evapotranspiration (“ecohydrologic separation”) are thought to be caused – at least in part – by heterogeneity of flow paths in watersheds: some water travels quickly through the watershed (sustaining streamflow), while other water persists for longer (sustaining transpiration) (Berghuijs and Allen, 2019). Studies that use empirical observations of water isotopes often emphasize the importance of water originating from winter or wet-season precipitation for sustaining plant transpiration (Miguez-Macho and Fan, 2021). However, the same study by Miguez-Macho and Fan (2021), when modeling the age of water taken up by plants, found that global ecosystems use only 30 % of past precipitation annually, while isotope-based approaches result in estimates of 50 ± 21 % ecosystem use of past precipitation. In general, studies that use particle tracking models show that precipitation is partitioned more rapidly to plants than streamflow (Kuppel et al., 2020; Maxwell et al., 2019; Rahimpour Asenjan and Danesh-Yazdi, 2020; Wilusz et al., 2020). These modeling approaches are likely to be unable to incorporate the complex reality of preferential flow paths into watersheds, which influences the age of water fluxes through the critical zone (Berghuijs and Allen, 2019; Sprenger and Allen, 2020). Including stable isotope tracer measurements (Sprenger et al., 2022a) and accounting for preferential flow paths (Knighton et al., 2019; Sprenger et al., 2018) in hydrologic models can improve estimates of plant water ages, suggesting that particle tracking models which assume a well-mixed soil domain do not capture the complexity of hydrologic flow paths and plant–water relations. Though modeling studies can provide information at scales larger than any tracer-based study, they do not easily allow for preferential flow within watersheds. Given that modeling approaches cannot capture complex water flow pathways, more water isotope data are needed to better understand the sources of water taken up by plants and the fate of precipitation in ecosystems.
The provisioning of precipitation to streams and plants is also mediated by hydroclimate. In a Mediterranean catchment, plants use precipitation that is older than streamflow, and the age of water taken up by plants varies intra-annually (Sprenger et al., 2022a). Plants in North America take up older water in dry climates and younger water in humid climates (Hahm et al., 2022). Other studies have shown that streamflow (von Freyberg et al., 2018) and plant waters (Allen et al., 2019; Goldsmith et al., 2022) are comprised of more recent precipitation in wetter climates than drier climates. Our results also show the prevalence of young water in plants and suggest that both wet- and dry-season precipitation contribute to evapotranspiration, perhaps because precipitation is greater than actual evapotranspiration demands year-round – even in the dry season (Clark et al., 2014). Though in wet environments plants will take up recent precipitation, we still lack a systematic understanding over a range of hydroclimates of how much wet- and dry-season precipitation will contribute to each season's evapotranspiration and streamflow.
We measured water isotopes of precipitation, lysimeter, stream, and branch xylem water in a tropical montane cloud forest to estimate the seasonal origins as well as young and new water fractions for different pools and fluxes of water. Branch xylem demonstrated strong intra-annual variability in the seasonal origins of source water for the 2-year period during which plant samples were collected and comparatively high Fyw (37 %) and Fnew (12 %). In comparison, stream water demonstrated no intra-annual trend in the seasonal origin index and low Fyw (5 %) and Fnew (0 %). Soil lysimeter waters from 1 m depth also demonstrated no intra-annual trend in the seasonal origin index and had low Fyw (riparian: 5 %; hillslope: 16 %) and Fnew (riparian and hillslope: 0 %). Taken together, the high Fyw, Fnew, and changing seasonal origin of all three tree species studied here are indicative of transpiration supplied by water within shallow soils that is consistently replenished by recent precipitation, while estimates for streamflow are indicative of water that predominantly fell as wet-season precipitation and originated from a large, seasonally buffered water reservoir. Interestingly, soil waters more closely mirrored results from stream waters than plant waters. By simultaneously studying the seasonal origins and ages of water in plants, soils, and streams over time, our results provide an important comparison to past studies in drier climates that show how the amount and seasonality of precipitation interact with subsurface storage and plant rooting patterns to shape the flow of water through ecosystems.
All the stable isotope, discharge, and precipitation data used for the analysis, together with the code for the young water fraction bootstrap resampling routine, are available online via https://doi.org/10.4211/hs.fdfdddbc35494e21ad2dda279f81832b (Burt et al., 2023c).
The supplement related to this article is available online at: https://doi.org/10.5194/hess-27-4173-2023-supplement.
EIB, RMCdH, AJCQ, and AJW designed and carried out the fieldwork. EIB and GRG carried out the isotope analyses. EIB wrote the code, analyzed the data, and made the figures with input from GRG, AJW, and RMCdH. EIB and GRG wrote the manuscript with input from all the authors.
The contact author has declared that none of the authors has any competing interests.
Publisher's note: Copernicus Publications remains neutral with regard to jurisdictional claims made in the text, published maps, institutional affiliations, or any other geographical representation in this paper. While Copernicus Publications makes every effort to include appropriate place names, the final responsibility lies with the authors.
We thank the Andes Biodiversity and Ecosystem Research Group (ABERG) for their support in the field and access to rainfall data (collected with the support of NSF DEB LTREB 1754647 to Miles Silman). We thank the Amazon Conservation Association, the Wayqecha Biological Station, as well as Abra Atwood and Daxs Herson Coayla Rimachi for their support in the field. We thank Alex Sessions and Fenfang Wu at Caltech, Markus Bill at Lawrence Berkeley National Lab, and Fernando Silva at Chapman University for support with the stable isotope measurements. We thank Caleb Henshaw for his assistance with the post-cryogenic extraction drying and weighing of branches. We thank James Kirchner and Scott Allen for their feedback. We thank the Servicio Nacional Forestal y de Fauna Silvestre (SERFOR) for their support in permitting this work (permit no. 142-2016-SERFOR/DGGSPFFS).
This project was funded by National Science Foundation Award no. EAR-1455352 to A. Joshua West and USDA Award no. 2020-67014-30917 to Gregory R. Goldsmith.
This paper was edited by Miriam Coenders-Gerrits and reviewed by two anonymous referees.
Allen, S. T., Kirchner, J. W., and Goldsmith, G. R.: Predicting Spatial Patterns in Precipitation Isotope (δ2H and δ18O) Seasonality Using Sinusoidal Isoscapes, Geophys. Res. Lett., 45, 4859–4868, https://doi.org/10.1029/2018GL077458, 2018.
Allen, S. T., Kirchner, J. W., Braun, S., Siegwolf, R. T. W., and Goldsmith, G. R.: Seasonal origins of soil water used by trees, Hydrol. Earth Syst. Sci., 23, 1199–1210, https://doi.org/10.5194/hess-23-1199-2019, 2019.
Asner, G. P., Martin, R. E., Anderson, C. B., Kryston, K., Vaughn, N., Knapp, D. E., Bentley, L. P., Shenkin, A., Salinas, N., Sinca, F., Tupayachi, R., Quispe Huaypar, K., Montoya Pillco, M., Ccori Álvarez, F. D., Díaz, S., Enquist, B. J., and Malhi, Y.: Scale dependence of canopy trait distributions along a tropical forest elevation gradient, New Phytol., 214, 973–988, https://doi.org/10.1111/nph.14068, 2017.
Benettin, P., Volkmann, T. H. M., von Freyberg, J., Frentress, J., Penna, D., Dawson, T. E., and Kirchner, J. W.: Effects of climatic seasonality on the isotopic composition of evaporating soil waters, Hydrol. Earth Syst. Sci., 22, 2881–2890, https://doi.org/10.5194/hess-22-2881-2018, 2018.
Benettin, P., Rodriguez, N. B., Sprenger, M., Kim, M., Klaus, J., Harman, C. J., van der Velde, Y., Hrachowitz, M., Botter, G., McGuire, K. J., Kirchner, J. W., Rinaldo, A., and McDonnell, J. J.: Transit Time Estimation in Catchments: Recent Developments and Future Directions, Water Resour. Res., 58, e2022WR033096, https://doi.org/10.1029/2022WR033096, 2022.
Berghuijs, W. R. and Allen, S. T.: Waters flowing out of systems are younger than the waters stored in those same systems, Hydrol. Process., 33, 3251–3254, https://doi.org/10.1002/hyp.13569, 2019.
Berghuijs, W. R. and Kirchner, J. W.: The relationship between contrasting ages of groundwater and streamflow: Connecting Storage and Streamflow Ages, Geophys. Res. Lett., 44, 8925–8935, https://doi.org/10.1002/2017GL074962, 2017.
Berkelhammer, M., Still, C. J., Ritter, F., Winnick, M., Anderson, L., Carroll, R., Carbone, M., and Williams, K. H.: Persistence and Plasticity in Conifer Water-Use Strategies, J. Geophys. Res.-Biogeo., 125, e2018JG00484, https://doi.org/10.1029/2018JG004845, 2020.
Botter, G., Bertuzzo, E., and Rinaldo, A.: Transport in the hydrologic response: Travel time distributions, soil moisture dynamics, and the old water paradox: A Theory of transport in the hydrologic response, Water Resour. Res., 46, W03514, https://doi.org/10.1029/2009WR008371, 2010.
Brinkmann, N., Seeger, S., Weiler, M., Buchmann, N., Eugster, W., and Kahmen, A.: Employing stable isotopes to determine the residence times of soil water and the temporal origin of water taken up by Fagus sylvatica and Picea abies in a temperate forest, New Phytol., 219, 1300–1313, https://doi.org/10.1111/nph.15255, 2018.
Brooks, J. R., Barnard, H. R., Coulombe, R., and McDonnell, J. J.: Ecohydrologic separation of water between trees and streams in a Mediterranean climate, Nat. Geosci., 3, 100–104, https://doi.org/10.1038/ngeo722, 2010.
Burt, E. I., Coayla Rimachi, D. H., Ccahuana Quispe, A. J., Atwood, A., and West, A. J.: Isotope-derived young water fractions in streamflow across the tropical Andes mountains and Amazon floodplain, Hydrol. Earth Syst. Sci., 27, 2883–2898, https://doi.org/10.5194/hess-27-2883-2023, 2023a.
Burt, E. I., Coayla Rimachi, D. H., Ccahuana Quispe, A. J., and West, A. J.: Oxygen and hydrogen isotopes in streams and precipitation and young water fractions across the Andes mountains and Amazon floodplain, HydroShare [data set], https://doi.org/10.4211/hs.c01ef51ca2b3495785d0f24c62142e23, 2023b.
Burt, E. I., Goldsmith, G. R., Cruz-de Hoyos, R., Ccahuana Quispe, A. J., and West, A. J.: Stable isotopes of water and seasonal origin indices of precipitation, stream, lysimeter and plant xylem waters, HydroShare [data set], https://doi.org/10.4211/hs.fdfdddbc35494e21ad2dda279f81832b, 2023c.
Carlotto, V., Gil, W., Cárdenas, J., Chávez, R., Ascue, J., and Rozas, H.: Mapa geológico del cuadrángulo de Calca 27s, Instituto Geológico Minero y Metalúrgico, Lima, Perú, https://hdl.handle.net/20.500.12544/187 (last access: 1 March 2023), 1996.
Chen, Y., Helliker, B. R., Tang, X., Li, F., Zhou, Y., and Song, X.: Stem water cryogenic extraction biases estimation in deuterium isotope composition of plant source water, P. Natl. Acad. Sci., 117, 33345–33350, https://doi.org/10.1073/pnas.2014422117, 2020.
Clark, K. E., Torres, M. A., West, A. J., Hilton, R. G., New, M., Horwath, A. B., Fisher, J. B., Rapp, J. M., Robles Caceres, A., and Malhi, Y.: The hydrological regime of a forested tropical Andean catchment, Hydrol. Earth Syst. Sci., 18, 5377–5397, https://doi.org/10.5194/hess-18-5377-2014, 2014.
Dai, J., Zhang, X., Wang, L., Luo, Z., Wang, R., Liu, Z., He, X., Rao, Z., and Guan, H.: Seasonal isotopic cycles used to identify transit times and the young water fraction within the critical zone in a subtropical catchment in China, J. Hydrol., 612, 128138, https://doi.org/10.1016/j.jhydrol.2022.128138, 2022.
Dawson, T. E. and Ehleringer, J. R.: Streamside trees that do not use stream water, Nature, 350, 335–337, https://doi.org/10.1038/350335a0, 1991.
Diao, H., Schuler, P., Goldsmith, G. R., Siegwolf, R. T. W., Saurer, M., and Lehmann, M. M.: Technical note: On uncertainties in plant water isotopic composition following extraction by cryogenic vacuum distillation, Hydrol. Earth Syst. Sci., 26, 5835–5847, https://doi.org/10.5194/hess-26-5835-2022, 2022.
Ehleringer, J. R. and Dawson, T. E.: Water uptake by plants: perspectives from stable isotope composition, Plant Cell Environ., 15, 1073–1082, https://doi.org/10.1111/j.1365-3040.1992.tb01657.x, 1992.
Ehleringer, J. R., Phillips, S. L., Schuster, W. S. F., and Sandquist, D. R.: Differential utilization of summer rains by desert plants, Oecologia, 88, 430–434, https://doi.org/10.1007/BF00317589, 1991.
Evaristo, J. and McDonnell, J. J.: Prevalence and magnitude of groundwater use by vegetation: a global stable isotope meta-analysis, Sci. Rep., 7, 44110, https://doi.org/10.1038/srep44110, 2017.
Evaristo, J., Jasechko, S., and McDonnell, J. J.: Global separation of plant transpiration from groundwater and streamflow, Nature, 525, 91–94, https://doi.org/10.1038/nature14983, 2015.
Evaristo, J., McDonnell, J. J., Scholl, M. A., Bruijnzeel, L. A., and Chun, K. P.: Insights into plant water uptake from xylem-water isotope measurements in two tropical catchments with contrasting moisture conditions: Ecohydrological Separation in Less Seasonal Humid Tropics, Hydrol. Process., 30, 3210–3227, https://doi.org/10.1002/hyp.10841, 2016.
Evaristo, J., Kim, M., Haren, J., Pangle, L. A., Harman, C. J., Troch, P. A., and McDonnell, J. J.: Characterizing the Fluxes and Age Distribution of Soil Water, Plant Water, and Deep Percolation in a Model Tropical Ecosystem, Water Resour. Res., 55, 3307–3327, https://doi.org/10.1029/2018WR023265, 2019.
Fan, Y., Miguez-Macho, G., Jobbágy, E. G., Jackson, R. B., and Otero-Casal, C.: Hydrologic regulation of plant rooting depth, P. Natl. Acad. Sci., 114, 10572–10577, https://doi.org/10.1073/pnas.1712381114, 2017.
Feakins, S. J. and Sessions, A. L.: Controls on the ratios of plant leaf waxes in an arid ecosystem, Geochim. Cosmochim. Ac., 74, 2128–2141, https://doi.org/10.1016/j.gca.2010.01.016, 2010.
Feakins, S. J., Bentley, L. P., Salinas, N., Shenkin, A., Blonder, B., Goldsmith, G. R., Ponton, C., Arvin, L. J., Wu, M. S., Peters, T., West, A. J., Martin, R. E., Enquist, B. J., Asner, G. P., and Malhi, Y.: Plant leaf wax biomarkers capture gradients in hydrogen isotopes of precipitation from the Andes and Amazon, Geochim. Cosmochim. Ac., 182, 155–172, https://doi.org/10.1016/j.gca.2016.03.018, 2016.
Feakins, S. J., Wu, M. S., Ponton, C., Galy, V., and West, A. J.: Dual isotope evidence for sedimentary integration of plant wax biomarkers across an Andes-Amazon elevation transect, Geochim. Cosmochim. Ac., 242, 64–81, https://doi.org/10.1016/j.gca.2018.09.007, 2018.
Gallart, F., Valiente, M., Llorens, P., Cayuela, C., Sprenger, M., and Latron, J.: Investigating young water fractions in a small Mediterranean mountain catchment: Both precipitation forcing and sampling frequency matter, Hydrol. Process., 34, 3618–3634, https://doi.org/10.1002/hyp.13806, 2020.
Girardin, C. A. J., Malhi, Y., Aragão, L. E. O. C., Mamani, M., Huaraca Huasco, W., Durand, L., Feeley, K. J., Rapp, J., Silva-Espejo, J. E., Silman, M., Salinas, N., and Whittaker, R. J.: Net primary productivity allocation and cycling of carbon along a tropical forest elevational transect in the Peruvian Andes: Net primary productivity from Andes to Amazon, Glob. Change Biol., 16, 3176–3192, https://doi.org/10.1111/j.1365-2486.2010.02235.x, 2010a.
Girardin, C. A. J., Malhi, Y., Aragão, L. E. O. C., Mamani, M., Huaraca Huasco, W., Durand, L., Feeley, K. J., Rapp, J., Silva-Espejo, J. E., Silman, M., Salinas, N., and Whittaker, R. J.: Net primary productivity allocation and cycling of carbon along a tropical forest elevational transect in the Peruvian Andes: Net primary productivity from andes to amazon, Glob. Change Biol., 16, 3176–3192, https://doi.org/10.1111/j.1365-2486.2010.02235.x, 2010b.
Girardin, C. A. J., Aragão, L. E. O. C., Malhi, Y., Huaraca Huasco, W., Metcalfe, D. B., Durand, L., Mamani, M., Silva-Espejo, J. E., and Whittaker, R. J.: Fine root dynamics along an elevational gradient in tropical Amazonian and Andean forests: Fine root dynamics from Andes to Amazon, Global Biogeochem. Cy., 27, 252–264, https://doi.org/10.1029/2011GB004082, 2013.
Girardin, C. A. J., Espejob, J. E. S., Doughty, C. E., Huasco, W. H., Metcalfe, D. B., Durand-Baca, L., Marthews, T. R., Aragao, L. E. O. C., Farfán-Rios, W., García-Cabrera, K., Halladay, K., Fisher, J. B., Galiano-Cabrera, D. F., Huaraca-Quispe, L. P., Alzamora-Taype, I., Eguiluz-Mora, L., Revilla, N. S., Silman, M. R., Meir, P., and Malhi, Y.: Productivity and carbon allocation in a tropical montane cloud forest in the Peruvian Andes, Plant Ecol. Divers., 7, 107–123, https://doi.org/10.1080/17550874.2013.820222, 2014.
Goldsmith, G. R., Muñoz-Villers, L. E., Holwerda, F., McDonnell, J. J., Asbjornsen, H., and Dawson, T. E.: Stable isotopes reveal linkages among ecohydrological processes in a seasonally dry tropical montane cloud forest: Tropical montane cloud forest ecohydrology, Ecohydrology, 5, 779–790, https://doi.org/10.1002/eco.268, 2012.
Goldsmith, G. R., Lehmann, M. M., Cernusak, L. A., Arend, M., and Siegwolf, R. T. W.: Inferring foliar water uptake using stable isotopes of water, Oecologia, 184, 763–766, https://doi.org/10.1007/s00442-017-3917-1, 2017a.
Goldsmith, G. R., Bentley, L. P., Shenkin, A., Salinas, N., Blonder, B., Martin, R. E., Castro-Ccossco, R., Chambi-Porroa, P., Diaz, S., Enquist, B. J., Asner, G. P., and Malhi, Y.: Variation in leaf wettability traits along a tropical montane elevation gradient, New Phytol., 214, 989–1001, https://doi.org/10.1111/nph.14121, 2017b.
Goldsmith, G. R., Allen, S. T., Braun, S., Siegwolf, R. T. W., and Kirchner, J. W.: Climatic Influences on Summer Use of Winter Precipitation by Trees, Geophys. Res. Lett., 49, e2022GL098323, https://doi.org/10.1029/2022GL098323, 2022.
Guo, J. S., Hungate, B. A., Kolb, T. E., and Koch, G. W.: Water source niche overlap increases with site moisture availability in woody perennials, Plant Ecol., 219, 719–735, https://doi.org/10.1007/s11258-018-0829-z, 2018.
Hahm, W. J., Lapides, D. A., Rempe, D. M., McCormick, E. L., and Dralle, D. N.: The Age of Evapotranspiration: Lower-Bound Constraints From Distributed Water Fluxes Across the Continental United States, Water Resour. Res., 58, e2022WR032961, https://doi.org/10.1029/2022WR032961, 2022.
Hale, V. C. and McDonnell, J. J.: Effect of bedrock permeability on stream base flow mean transit time scaling relations: 1. A multiscale catchment intercomparison, Water Resour. Res., 52, 1358–1374, https://doi.org/10.1002/2014WR016124, 2016.
Hale, V. C., McDonnell, J. J., Stewart, M. K., Solomon, D. K., Doolitte, J., Ice, G. G., and Pack, R. T.: Effect of bedrock permeability on stream base flow mean transit time scaling relationships: 2. Process study of storage and release, Water Resour. Res., 52, 1375–1397, https://doi.org/10.1002/2015WR017660, 2016.
Harman, C. J.: Time-variable transit time distributions and transport: Theory and application to storage-dependent transport of chloride in a watershed, Water Resour. Res., 51, 1–30, https://doi.org/10.1002/2014WR015707, 2015.
Harpold, A. A.: Diverging sensitivity of soil water stress to changing snowmelt timing in the Western U.S., Adv. Water Resour., 92, 116–129, https://doi.org/10.1016/j.advwatres.2016.03.017, 2016.
Hooper, R. P., Christophersen, N., and Peters, N. E.: Modelling streamwater chemistry as a mixture of soilwater end-members – An application to the Panola Mountain catchment, Georgia, U.S.A., J. Hydrol., 116, 321–343, https://doi.org/10.1016/0022-1694(90)90131-G, 1990.
Hu, J., Moore, D. J. P., Burns, S. P., and Monson, R. K.: Longer growing seasons lead to less carbon sequestration by a subalpine forest, Glob. Change Biol., 16, 771–783, https://doi.org/10.1111/j.1365-2486.2009.01967.x, 2010.
Jackson, P. C., Cavelier, J., Goldstein, G., Meinzer, F. C., and Holbrook, N. M.: Partitioning of water resources among plants of a lowland tropical forest, Oecologia, 101, 197–203, https://doi.org/10.1007/BF00317284, 1995.
Jasechko, S.: Global Isotope Hydrogeology Review, Rev. Geophys., 57, 835–965, https://doi.org/10.1029/2018RG000627, 2019.
Jasechko, S., Kirchner, J. W., Welker, J. M., and McDonnell, J. J.: Substantial proportion of global streamflow less than three months old, Nat. Geosci., 9, 126–129, https://doi.org/10.1038/ngeo2636, 2016.
Kerhoulas, L. P., Kolb, T. E., and Koch, G. W.: Tree size, stand density, and the source of water used across seasons by ponderosa pine in northern Arizona, Forest Ecol. Manag., 289, 425–433, https://doi.org/10.1016/j.foreco.2012.10.036, 2013.
Kirchner, J. W.: Aggregation in environmental systems – Part 1: Seasonal tracer cycles quantify young water fractions, but not mean transit times, in spatially heterogeneous catchments, Hydrol. Earth Syst. Sci., 20, 279–297, https://doi.org/10.5194/hess-20-279-2016, 2016.
Kirchner, J. W.: Quantifying new water fractions and transit time distributions using ensemble hydrograph separation: theory and benchmark tests, Hydrol. Earth Syst. Sci., 23, 303–349, https://doi.org/10.5194/hess-23-303-2019, 2019.
Kirchner, J. W. and Allen, S. T.: Seasonal partitioning of precipitation between streamflow and evapotranspiration, inferred from end-member splitting analysis, Hydrol. Earth Syst. Sci., 24, 17–39, https://doi.org/10.5194/hess-24-17-2020, 2020.
Kirchner, J. W., Benettin, P., and van Meerveld, I.: Instructive Surprises in the Hydrological Functioning of Landscapes, Annu. Rev. Earth Pl. Sc., 51, 277–299, https://doi.org/10.1146/annurev-earth-071822-100356, 2023.
Knighton, J., Souter-Kline, V., Volkmann, T., Troch, P. A., Kim, M., Harman, C. J., Morris, C., Buchanan, B., and Walter, M. T.: Seasonal and Topographic Variations in Ecohydrological Separation Within a Small, Temperate, Snow-Influenced Catchment, Water Resour. Res., 55, 6417–6435, https://doi.org/10.1029/2019WR025174, 2019.
Kuppel, S., Tetzlaff, D., Maneta, M. P., and Soulsby, C.: Critical Zone Storage Controls on the Water Ages of Ecohydrological Outputs, Geophys. Res. Lett., 47, e2020GL088897, https://doi.org/10.1029/2020GL088897, 2020.
Lahuatte, B., Mosquera, G. M., Páez-Bimos, S., Calispa, M., Vanacker, V., Zapata-Ríos, X., Muñoz, T., and Crespo, P.: Delineation of water flow paths in a tropical Andean headwater catchment with deep soils and permeable bedrock, Hydrol. Process., 36, e14725, https://doi.org/10.1002/hyp.14725, 2022.
Lazo, P. X., Mosquera, G. M., McDonnell, J. J., and Crespo, P.: The role of vegetation, soils, and precipitation on water storage and hydrological services in Andean Páramo catchments, J. Hydrol., 572, 805–819, https://doi.org/10.1016/j.jhydrol.2019.03.050, 2019.
Lutz, S. R., Krieg, R., Müller, C., Zink, M., Knöller, K., Samaniego, L., and Merz, R.: Spatial Patterns of Water Age: Using Young Water Fractions to Improve the Characterization of Transit Times in Contrasting Catchments, Water Resour. Res., 54, 4767–4784, https://doi.org/10.1029/2017WR022216, 2018.
Martin, J., Looker, N., Hoylman, Z., Jencso, K., and Hu, J.: Differential use of winter precipitation by upper and lower elevation Douglas fir in the Northern Rockies, Glob. Change Biol., 24, 5607–5621, https://doi.org/10.1111/gcb.14435, 2018.
Maxwell, R. M., Condon, L. E., Danesh-Yazdi, M., and Bearup, L. A.: Exploring source water mixing and transient residence time distributions of outflow and evapotranspiration with an integrated hydrologic model and Lagrangian particle tracking approach, Ecohydrology, 12, e2042, https://doi.org/10.1002/eco.2042, 2019.
McCormick, E. L., Dralle, D. N., Hahm, W. J., Tune, A. K., Schmidt, L. M., Chadwick, K. D., and Rempe, D. M.: Widespread woody plant use of water stored in bedrock, Nature, 597, 225–229, https://doi.org/10.1038/s41586-021-03761-3, 2021.
McGuire, K. J. and McDonnell, J. J.: A review and evaluation of catchment transit time modeling, J. Hydrol., 330, 543–563, https://doi.org/10.1016/j.jhydrol.2006.04.020, 2006.
Miguez-Macho, G. and Fan, Y.: Spatiotemporal origin of soil water taken up by vegetation, Nature, 598, 624–628, https://doi.org/10.1038/s41586-021-03958-6, 2021.
Muñoz-Villers, L. E. and McDonnell, J. J.: Runoff generation in a steep, tropical montane cloud forest catchment on permeable volcanic substrate, Water Resour. Res., 48, 2011WR011316, https://doi.org/10.1029/2011WR011316, 2012.
Ponton, C., West, A. J., Feakins, S. J., and Galy, V.: Leaf wax biomarkers in transit record river catchment composition, Geophys. Res. Lett., 41, 6420–6427, https://doi.org/10.1002/2014GL061328, 2014.
Rahimpour Asenjan, M. and Danesh-Yazdi, M.: The effect of seasonal variation in precipitation and evapotranspiration on the transient travel time distributions, Adv. Water Resour., 142, 103618, https://doi.org/10.1016/j.advwatres.2020.103618, 2020.
Rapp, J. and Silman, M.: Diurnal, seasonal, and altitudinal trends in microclimate across a tropical montane cloud forest, Clim. Res., 55, 17–32, https://doi.org/10.3354/cr01127, 2012.
Rapp, J. M., Silman, M. R., Clark, J. S., Girardin, C. A. J., Galiano, D., and Tito, R.: Intra- and interspecific tree growth across a long altitudinal gradient in the Peruvian Andes, Ecology, 93, 2061–2072, https://doi.org/10.1890/11-1725.1, 2012.
Rempe, D. M. and Dietrich, W. E.: Direct observations of rock moisture, a hidden component of the hydrologic cycle, P. Natl. Acad. Sci., 115, 2664–2669, https://doi.org/10.1073/pnas.1800141115, 2018.
Sohel, Md. S. I., Grau, A. V., McDonnell, J. J., and Herbohn, J.: Tropical forest water source patterns revealed by stable isotopes: A preliminary analysis of 46 neighboring species, Forest Ecol. Manag., 494, 119355, https://doi.org/10.1016/j.foreco.2021.119355, 2021.
Sprenger, M. and Allen, S. T.: What Ecohydrologic Separation Is and Where We Can Go With It, Water Resour. Res., 56, e2020WR02723, https://doi.org/10.1029/2020WR027238, 2020.
Sprenger, M., Tetzlaff, D., Buttle, J., Laudon, H., Leistert, H., Mitchell, C. P. J., Snelgrove, J., Weiler, M., and Soulsby, C.: Measuring and Modeling Stable Isotopes of Mobile and Bulk Soil Water, Vadose Zone J., 17, 1–18, https://doi.org/10.2136/vzj2017.08.0149, 2018.
Sprenger, M., Stumpp, C., Weiler, M., Aeschbach, W., Allen, S. T., Benettin, P., Dubbert, M., Hartmann, A., Hrachowitz, M., Kirchner, J. W., McDonnell, J. J., Orlowski, N., Penna, D., Pfahl, S., Rinderer, M., Rodriguez, N., Schmidt, M., and Werner, C.: The Demographics of Water: A Review of Water Ages in the Critical Zone, Rev. Geophys., 57, 800–834, https://doi.org/10.1029/2018RG000633, 2019.
Sprenger, M., Llorens, P., Gallart, F., Benettin, P., Allen, S. T., and Latron, J.: Precipitation fate and transport in a Mediterranean catchment through models calibrated on plant and stream water isotope data, Hydrol. Earth Syst. Sci., 26, 4093–4107, https://doi.org/10.5194/hess-26-4093-2022, 2022a.
Sprenger, M., Carroll, R. W. H., Dennedy-Frank, J., Siirila-Woodburn, E. R., Newcomer, M. E., Brown, W., Newman, A., Beutler, C., Bill, M., Hubbard, S. S., and Williams, K. H.: Variability of Snow and Rainfall Partitioning Into Evapotranspiration and Summer Runoff Across Nine Mountainous Catchments, Geophys. Res. Lett., 49, e2022GL099324, https://doi.org/10.1029/2022GL099324, 2022b.
von Freyberg, J., Allen, S. T., Seeger, S., Weiler, M., and Kirchner, J. W.: Sensitivity of young water fractions to hydro-climatic forcing and landscape properties across 22 Swiss catchments, Hydrol. Earth Syst. Sci., 22, 3841–3861, https://doi.org/10.5194/hess-22-3841-2018, 2018.
von Freyberg, J., Allen, S. T., Grossiord, C., and Dawson, T. E.: Plant and root-zone water isotopes are difficult to measure, explain, and predict: Some practical recommendations for determining plant water sources, Methods Ecol. Evol., 11, 1352–1367, https://doi.org/10.1111/2041-210X.13461, 2020.
Wilusz, D. C., Harman, C. J., Ball, W. P., Maxwell, R. M., and Buda, A. R.: Using Particle Tracking to Understand Flow Paths, Age Distributions, and the Paradoxical Origins of the Inverse Storage Effect in an Experimental Catchment, Water Resour. Res., 56, e2019WR02514, https://doi.org/10.1029/2019WR025140, 2020.
Wu, M. S., West, A. J., and Feakins, S. J.: Tropical soil profiles reveal the fate of plant wax biomarkers during soil storage, Org. Geochem., 128, 1–15, https://doi.org/10.1016/j.orggeochem.2018.12.011, 2019.
Zhang, Z. Q., Evaristo, J., Li, Z., Si, B. C., and McDonnell, J. J.: Tritium analysis shows apple trees may be transpiring water several decades old, Hydrol. Process., 31, 1196–1201, https://doi.org/10.1002/hyp.11108, 2017.