the Creative Commons Attribution 4.0 License.
the Creative Commons Attribution 4.0 License.
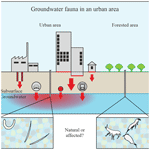
Groundwater fauna in an urban area – natural or affected?
Kathrin Menberg
Svenja Schweikert
Cornelia Spengler
Hans Jürgen Hahn
Philipp Blum
In Germany, 70 % of the drinking water demand is met by groundwater, for which the quality is the product of multiple physical–chemical and biological processes. As healthy groundwater ecosystems help to provide clean drinking water, it is necessary to assess their ecological conditions. This is particularly true for densely populated urban areas, where faunistic groundwater investigations are still scarce. The aim of this study is, therefore, to provide a first assessment of the groundwater fauna in an urban area. Thus, we examine the ecological status of an anthropogenically influenced aquifer by analysing fauna in 39 groundwater monitoring wells in the city of Karlsruhe (Germany). For classification, we apply the groundwater ecosystem status index (GESI), in which a threshold of more than 70 % of crustaceans and less than 20 % of oligochaetes serves as an indication for very good and good ecological conditions. Our study reveals that only 35 % of the wells in the residential, commercial and industrial areas and 50 % of wells in the forested area fulfil these criteria. However, the study did not find clear spatial patterns with respect to land use and other anthropogenic impacts, in particular with respect to groundwater temperature. Nevertheless, there are noticeable differences in the spatial distribution of species in combination with abiotic groundwater characteristics in groundwater of the different areas of the city, which indicate that a more comprehensive assessment is required to evaluate the groundwater ecological status in more detail. In particular, more indicators, such as groundwater temperature, indicator species, delineation of site-specific characteristics and natural reference conditions should be considered.
- Article
(5423 KB) -
Supplement
(1488 KB) - BibTeX
- EndNote
In Germany, 70 % of the drinking water demand is met by groundwater, for which the quality is the product of multiple physical–chemical and biological processes (German Environment Agency, 2018). Groundwater ecosystems are responsible for several services that help to provide clean drinking water, which is a vital resource for humanity (Griebler and Avramov, 2015). Bacteria and fauna also play an important role in the biological self-purification of groundwater by the retention of organic matter, natural attenuation of pollutants, storing and buffering of nutrients as well as the elimination of pathogens. Organic matter and pollutants can be degraded and converted to biomass or bound by microbial activity. Protozoa and higher organisms can graze resulting biofilms, loosen the substrate and, therefore, stimulate biological self-purification (Hancock et al., 2005; Boulton et al., 2008; Griebler and Avramov, 2015).
Healthy groundwater ecosystems can provide clean drinking water; however, they are sensitive to external influences such as chemical and thermal disturbances. The latter drives hydro-geochemical and biological processes in groundwater systems which are typically isothermal (Brielmann et al., 2009, 2011). Groundwater fauna mainly consist of stygobiont species which spend their entire life in groundwater and are adjusted to this habitat (Hahn, 2006). Hence, in central Europe, they are assumed to be cold stenotherm, which means that they prefer cold temperatures. A variability in temperature tolerance among groundwater faunal groups and species is reported in various studies, which explains why the use of individual temperature thresholds is more useful for capturing different preferences. According to Spengler (2017), faunal diversity is generally declining at a temperature above 14 ∘C. Various authors reported species-specific temperature preferences between 8 and 16 ∘C (for individuals of the species Niphargus inopinatus and Proasselus cavaticus; Brielmann et al., 2009, 2011) and a specific temperature threshold of up to 19 ∘C (for Parastenocaris phyllura; Glatzel, 1990). Above these thresholds, the mortality of individuals raises until groundwater fauna is almost absent, for example at 22 ∘C in the study of Foulquier et al. (2011). However, temperature sensitivity is not only an issue at species level but also for the communities as a whole. Spengler (2017) reported 12 ∘C to be a temperature threshold value indicated by a shift in community structure for faunal communities of groundwater of the Upper Rhine valley.
Nevertheless, in German and European legislation, as in many countries globally, groundwater is not yet recognised as a habitat worthy of protection, and there is no common understanding of the best practice for assessing the ecological status of groundwater (Hahn et al., 2018; Spengler and Hahn, 2018). The assessment of surface water is typically based on biological and physical–chemical criteria and is also supported by hydro-morphological criteria (European Water Framework Directive and German legislation; article 5 – “Regulation on the Protection of Surface Water”). While groundwater quality is mostly assessed by physical–chemical and quantitative criteria, very few quantifiable ecological criteria are available for the assessment of the health of groundwater ecosystems. The availability of ecological criteria can only be increased by conducting a large number of studies dealing with the analyses of groundwater ecosystem health by investigating groundwater fauna. Results from previous faunistic groundwater analyses are contained in a Germany-wide data record (Hahn, 2005; Berkhoff, 2010; Stein et al., 2012; Gutjahr, 2013; Spengler, 2017; Spengler and Hahn, 2018). The study by Hahn and Fuchs (2009) focuses on defining stygoregions based on different hydrogeological units located in Baden-Württemberg, Germany. They conclude that the observed patterns of groundwater communities reflect a high spatial and temporal heterogeneity in aquifer types with respect to habitat structure, food and oxygen supply. Although there are various studies on this topic (e.g. Gibert and Deharveng, 2002; Malard et al., 2002; Deharveng et al., 2009; Dole-Olivier et al., 2009b), stygobiotic biodiversity is still likely to be underestimated.
Regional investigations on the spatial variation in groundwater fauna, i.e. stygobiont occurrences, and corresponding environmental parameters, such as geological site characteristics and altitude, are rare (Dole-Olivier et al., 2009a; Gibert et al., 2009). An approach for elucidating groundwater biodiversity patterns in six European regions was conducted in the PASCALIS (Protocol for the Assessment and Conservation of Aquatic Life In the Subsurface) project (Gibert et al., 2009), which aimed at mapping biodiversity and endemism patterns (Deharveng et al., 2009) and shows that regional processes, such as hydrological connectivity, in a specific habitat (e.g. river floodplains as in Ward and Tockner, 2001) have a much stronger influence on species composition than local habitat features, such as permeability and saturation. Within a region, hydrogeology, altitude, palaeographical factors and human activities can interact in complex ways to produce dissimilar patterns of species compositions and diversity (Gibert et al., 2009). The PASCALIS sampling protocol recommends selecting hydro-geographic basins that are not strongly affected by human activities, such as groundwater pollution (Malard et al., 2002), and do not biogeographically classify a groundwater system (Stein et al., 2012).
In urban areas, anthropogenic impacts such as a dense building development, underground car parks, open geothermal systems and injections of thermal wastewater from industry result in local thermal alteration of groundwater by up to several degrees (e.g. Taylor and Stefan, 2009; Zhu et al., 2010; Menberg et al., 2013b; Tissen et al., 2019). According to Brielmann et al. (2011), annual temperature fluctuations in aquifers caused by shallow geothermal energy systems range between 4 ∘C in winter and ≤20 ∘C in summer. In 2000, the European Union (EU) Water Framework Directive defined the release of heat in the groundwater as pollution, whereas the cooling of the groundwater is not mentioned. Until now, there are scientifically derived threshold values for groundwater temperature in the case of thermal (heat) pollution published, but none of these have been implemented in official regulations or water law (Hähnlein et al., 2010, 2013; Blum et al., 2021). This results in a tension between conservation, exploitation and thermal use of groundwater. However, as seen in an aquifer ecosystem downstream from an industrial facility in Freising (Germany), where groundwater is used for cooling, resulting in a warm thermal plume, no relation between faunal abundance and groundwater temperature could be identified (Brielmann et al., 2009). Investigation of hydro-geochemical parameters, microbial activities, bacterial communities and groundwater faunal assemblages indicates that bacterial diversity increased with temperature, while faunal diversity decreased with temperature (Brielmann et al., 2009). Similar results are provided by Griebler et al. (2016), where potential impacts of geothermal energy use and storage of heat on groundwater are investigated. Temperature changes in groundwater correspond to changes in groundwater chemistry, biodiversity, community composition, microbial processes and function of the ecosystem. How exactly groundwater communities react to changes in the temperature and concentration of nutrients, dissolved organic carbon and oxygen is not yet fully understood (Brielmann et al., 2009, 2011; Spengler, 2017; Castaño-Sánchez et al., 2020).
Several approaches exist that allow a local assessment of the ecological state of groundwater based on different faunistic, hydro-chemical and physical parameters. Korbel and Hose (2011, 2017) introduced the Groundwater Health Index (GHI), which is a tiered framework for assessing the health of groundwater ecosystems. Here, both biotic and abiotic attributes of groundwater ecosystems are used as benchmarks for ecosystem health. Their study shows that ecosystem health benchmarks are probably more associated with aquifer typology than being applicable for local areas. This index is applied and tested by Di Lorenzo et al. (2020b) in unconsolidated aquifers in Italy located in nitrate vulnerable zones. They refined the index (wGHIN) and demonstrated its applicability on shallow and deep aquifers and also revealed that this new index is limited due to low correlations between the indicators. Commissioned by the Federal Environmental Agency of Germany (Umweltbundesamt – UBA), Griebler et al. (2014) developed a concept for an ecologically based assessment scheme for groundwater ecosystems, which builds on the assessment of Korbel and Hose (2011, 2017). This two-step scheme characterises groundwater on two different levels by using the most important physical–chemical parameters, such as content of dissolved oxygen and microbiological and faunistic characteristics, i.e. the number of oligochaetes and crustaceans, and comparing these to reference values for natural, undisturbed and ecologically intact groundwater ecosystems (Griebler et al., 2014).
Furthermore, the groundwater fauna index (GFI), introduced by Hahn (2006), quantifies the relevant ecological conditions in the groundwater as a result of hydrological exchanges between surface and groundwater. It incorporates ecologically important groundwater parameters, such as the relative amount of detritus, variation in groundwater temperature and concentration of dissolved oxygen (Hahn, 2006). Gutjahr et al. (2014) used the GFI as part of a proposal for a groundwater habitat classification on a local scale, which introduced five types of faunistic habitats as a result of surface water influence, the content of dissolved oxygen and amount of organic matter. Moreover, in the study of Berkhoff (2010), the GFI was used to examine the impact of the surface water influence on groundwater with the aim of developing a faunistic monitoring concept for hydrological exchange processes in the surrounding river bank filtration plants. Spengler and Hahn (2018) argued for the definition of a regional and ecological temperature threshold and an ecology-based assessment of thermal stress in groundwater.
The objective of this study is to investigate, specifically, the groundwater fauna beneath residential, commercial and industrial, i.e. urban, areas in comparison to a forested area outside the built-up area of Karlsruhe to determine whether land use has an impact on groundwater faunal communities. Hence, in 39 groundwater monitoring wells in Karlsruhe, Germany, the groundwater fauna are sampled, the groundwater temperatures are measured and chemical properties are analysed. In our study, the classification scheme developed by Griebler et al. (2014) is applied. The wells are characterised regarding the state of their ecosystem. Finally, we aim to distinguish areas with natural groundwater ecology from anthropogenically disturbed areas.
2.1 Study site
The study is performed in Karlsruhe, a city in the Upper Rhine valley in southwestern Germany. The urban region covers an area of 173 km2 and has about 310 000 inhabitants (Amt für Stadtentwicklung – Statistikstelle, 2018). The Cenozoic continental rift valley is filled with Tertiary and Quaternary sediments, which are dominated by sands and gravels with minor contents of silt, clay and stones (Geyer et al., 2011). Sporadic layers with lower permeabilities lead to a separation of up to three aquifer levels (Wirsing and Luz, 2007). The upper aquifer is unconfined, with a water table between 2 and 10 m below the ground. The flow direction is northwest of the Rhine, with groundwater flow velocities ranging between 0.5 and 1.5 m/d (Technologiezentrum Wasser, 2018).
Based on the land use plan of Karlsruhe, about 20 % of the area (i.e. urban area, city centre, neighbouring districts, and parts of the Hardtwald forest and several outskirts) is covered by buildings. The rest is vegetation (∼56 %) and artificial surface covers (∼24 %), showing the complexity and heterogeneity of the urban environment. According to Benz et al. (2016), the annual mean groundwater temperature (GWT) in Karlsruhe in the years 2011 and 2012 was 13.0±1.0 ∘C. Distinct temperature hot spots occur mainly below the city centre, where building densities are highest. In the northwestern part of Karlsruhe, the increase in GWT was about 3 K warmer than the annual mean land surface temperature (LST), which is mainly caused by several groundwater reinjections of thermal wastewater (Benz et al., 2016).
In general, groundwater in the region of Karlsruhe is of good quality, and the local drinking water supplier (Stadtwerke Karlsruhe) only needs to remove oxidised iron and manganese from the pumped groundwater. However, two main contaminations which affect groundwater quality are known in the urban area (Stadt Karlsruhe, 2006). A contaminant plume, which contains a polycyclic aromatic hydrocarbons concentration of up to 500 µg/L, of 200 m length over the entire aquifer thickness is located at a former gas plant in the east of Karlsruhe (Fig. S1b; Kühlers et al., 2012). Moreover, three parallel contamination plumes, of 2.5 km length each, can be found in the southeast of Karlsruhe (Fig. S1b), where highly volatile chlorinated hydrocarbons (7–26 µg/L) and their degradation products were detected (Wickert et al., 2006).
2.2 Material and sampling
From 2011 to 2014, samplings of groundwater parameters and fauna were performed in 39 groundwater monitoring wells in the city area of Karlsruhe, of which eight wells are in the forested area and 31 in the residential, commercial and industrial areas (urban area). At the beginning of each sampling process, temperature and electrical conductivity were measured with an electric contact gauge (type 120-LTC; Hydrotechnik) at a depth interval of 1 m. Using a bailer (aqua sampler; Cole-Parmer), water from the bottom of the groundwater monitoring wells was sampled, and the pH value (MultiLine type 3430; Xylem Analytics, Weilheim, Germany) and the contents of dissolved oxygen (MultiLine type 3430; Xylem Analytics, Weilheim, Germany), iron, nitrate (NO) and phosphate (PO; (RQflex® plus 10 Reflectoquant®; Merck KGaA, Darmstadt, Germany) were measured.
In accordance with the suggestion made by Hahn and Gutjahr (2014), several integrative samplings (i.e. repeated samples taken over a period of time) were conducted to capture an ecological representation of groundwater fauna which reflects the occurring species at a community level. Every well was sampled at least three times. From 2011–2012, 22 measurement wells (mainly in the Hardtwald and the northwest of Karlsruhe) were sampled six times at a minimum interval of 2 months. In 2014, 17 measurement wells, mainly located in the south or in the inner city, were sampled three times (see Table S2). As the aim of this study is to provide a first-tier screening of the groundwater ecological status, we sampled the fauna in the monitoring wells in accordance with the sampling manual of the European PASCALIS project (Malard et al., 2002) and the procedure described by Hahn and Fuchs (2009), using a modified Cvetkov net.
Furthermore, the relative amount of sediment as an indication of the nutrient availability and the cavity system was measured. Before the fauna sample from the net sampler was passed over a sieve with a mesh size of 74 µm, the sediment was separated and classified into different categories (sand, fine sand, ochre, detritus, and silt). It should be noted that the detritus content was not recorded quantitatively but on the basis of estimated frequency classes. The estimation of the relative amounts of sediment per sample is based on Table S1 in the Supplement.
Mann–Whitney tests (U tests) were applied to detect the potential impacts of groundwater characteristics (physical–chemical parameters), geology and well design on the groundwater quality as well as on groundwater fauna. Samples were regarded as significantly different if the p value was .
To better understand large-scale relationships and the fine structures of high-dimensional biological data, the PHATE (Potential of Heat-diffusion for Affinity-based Trajectory Embedding) analysis introduced by Moon et al. (2019; https://github.com/KrishnaswamyLab/PHATE, last access: 15 April 2021) was used. This dimensionality reduction method generates a low-dimensional embedding specific for visualisation, which provides an accurate, denoised representation of both local and global structures of a data set without imposing strong assumptions on the structure of the data. The PHATE algorithm computes the pairwise distances from the data matrix and transforms the distances to affinities to encode local information by applying a kernel function, which is developed to Euclidian distances. By using diffusion processes, global relationships are learnt and encoded using the potential distance. Finally, the potential distance information is embedded into low dimensions for visualisation with metric multidimensional scaling (MDS; Moon et al., 2019). Objects that are close to each other in the final graph, therefore, have similar characteristics.
Crustaceans, especially amphipods and copepods represent the majority of groundwater fauna. The identification keys from the following studies were used to identify the different groups in the samples: Einsle (1993), Janetzka et al. (1996), Meisch (2000), Schellenberg (1942) and Schminke et al. (2007). The sampled fauna for this study can be assigned to the subphylum Crustacea and four other subordinate taxa (Table 1).
2.3 Classification scheme by Griebler et al. (2014)
Commissioned by the Federal Environmental Agency of Germany (UBA), Griebler et al. (2014) developed a two-step, ecologically based classification scheme for the characterisation of groundwater ecosystems and also defined spatially dependent reference values of ecologically intact groundwater ecosystems. In order to enable a statement about the exposure of the groundwater at a specific site, biotic and abiotic parameters, which are determined and compared with reference values, are used to distinguish locations with very good or good ecological conditions or locations which fail these criteria, i.e. affected areas (Fig. 1). If an ecological assessment of groundwater ecosystems, which is based on the groundwater fauna analysis, takes place, some faunistic criteria must be considered. Invertebrates avoid habitats that are ochred or have a low dissolved oxygen content. Thus, unstressed or natural habitats are defined as areas, with a dissolved oxygen content >1.0 mg/L, that are not ochred and have an existing fauna, i.e. an amount of > 50 % of stygobites, of > 70 % of crustaceans and of < 20 % of oligochaetes (Fig. 1). This allows a qualitative interpretation of the ecological condition of the groundwater system. If the results indicate affected ecological conditions, i.e. one or more biological and/or ecological indicators are out of the reference range, then an assessment according to the level 2 scheme is necessary. This requires a determination of the reference values at local reference locations, which are protected and have a weak surface influence, and a subsequent comparison of these values with measured data. As our aim is a first-tier screening of an urban area, we only apply level 1 in our study.
3.1 Physical and chemical parameters
First, the groundwater conditions in the study area are evaluated by their physical–chemical characteristics. The following values are average values of the individual samplings from each monitoring well. In order to allow for a spatially differentiated assessment, the study site (city area of Karlsruhe) is classified into different zones based on land use types provided by the European seamless vector data of the CORINE Land Cover (CLC) inventory (European Environment Agency, 2016). Based on this data, the city area is subdivided into (1) forested area (forest; local name – Hardtwald) and (2) industrial, commercial and residential areas (urban area; Fig. 2a). For simplification, the phrases forest and urban area are used in the following. A more detailed subdivision in the urban area did not appear reasonable due to the heterogeneous structure.
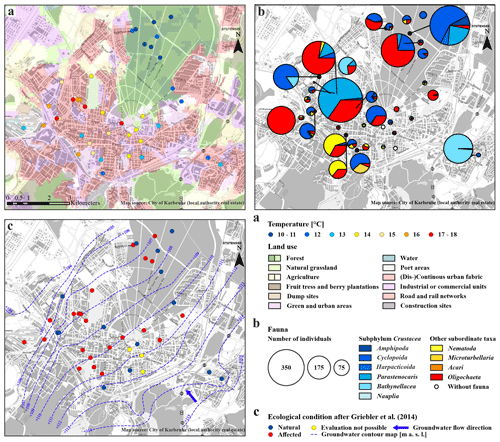
Figure 2Overview map of the city area of Karlsruhe. (a) Land use plan (European Environment Agency, 2016) and average groundwater temperature of the multiple measurements (degrees Celsius) at the bottom of the monitoring wells. (b) Detailed groundwater fauna – colours of the circles show the different taxa in the sample (percent), and the size indicates the number of individuals. (c) Faunistic evaluation, after Griebler et al. (2014), and groundwater contour map in metres above sea level (modified after the local authority real estate of Karlsruhe).
As expected, measured GWTs at the bottom of the wells with 8.5 to 39.0 m depth, are mainly constant over the repeated measurements. The lowest GWTs, ranging between 10.5 and 10.9 ∘C, were measured in the eight wells of the forested area (Table S2). In contrast, the highest average GWT, at 17.5 ∘C, was measured in a well near the city hospital (T113; Fig. 2a). The mean value of all wells is 13.5±2.1 ∘C, which is similar to the results from Benz et al. (2015), with 13.0±1.0 ∘C. According to Benz et al. (2017), annual shallow GWTs vary between 6 and 16 ∘C in the area of Karlsruhe, which is in line with the temperatures measured during fauna sampling (Fig. 3a). For the urban area in the northwestern part of the city, Fig. 2a shows a clear warming trend, which was also observed by Menberg et al. (2013a, b). The increased GWT in this area can be traced back to effects of urban infrastructures and industries, which use groundwater for cooling purposes.
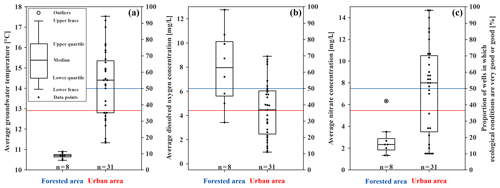
Figure 3Box plots of the physical and chemical parameters for the forested and urban area in the study site and the proportion of wells in which ecological conditions are very good or good (in percentage), indicated by the blue (forested area) and red (urban area) lines (secondary axis). (a) Average temperature of the repeated measurements (degrees Celsius) at the bottom of the monitoring wells. (b) Average content of dissolved oxygen (milligrams per litre) of the monitoring wells. (c) Average nitrate content (milligrams per litre) of each monitoring well (n is the number of wells).
The dissolved oxygen content acts as a limiting factor for groundwater fauna, since groundwater is usually undersaturated, with a varying oxygen content between 0 and 8 mg/L (Griebler et al., 2014; Kunkel et al., 2004). In this study, the average content of dissolved oxygen in all wells is between 1.0 and 12.8 mg/L (Figs. 3b and S1a). As expected, the monitoring wells located in the forested area (Hardtwald) show the highest content, while the lowest values are found in urban areas and are likely linked to aquifer contamination and other anthropogenic effects (dissolved oxygen content of forested vs. urban area; U test – p value = ; n=8; 31). Urban water can be polluted in multiple ways, which affects the chemical and biological oxygen consumption in the groundwater. The higher the pollution and/or biological activity, the lower the dissolved oxygen (Kunkel et al., 2004; Griebler et al., 2014). Moreover, it seems that, with a greater depth of the measurement wells, the dissolved oxygen content increases (U test – p value ; n=39). This can be explained by the fact that shallow wells can have a low water column in which oxygen can rapidly be consumed by groundwater microorganisms, chemical reactions and/or groundwater fauna. In the upper, unscreened part of deeper wells, dissolved oxygen can be consumed, while in the lower, screened part, oxygen is continuously being refilled by oxic groundwater from the surroundings (Malard et al., 2002). Furthermore, reducing conditions in the overlaying soil can result in a low content of dissolved oxygen in groundwater.
Nitrate is often named as an important pollutant in groundwater. The natural and geogenic concentration of nitrate in groundwater is usually under 10 mg/L (Griebler et al., 2014). In our study area, the average nitrate content of all wells varies between 1.3 and 14.7 mg/L. In the urban area, the average nitrate concentrations are generally higher and correlate with the content of dissolved oxygen (U test – p value ; n=39), showing the link between nitrate content and oxygen consumption. Wells with a dissolved oxygen content below 1.5 mg/L have an average nitrate content of 1.5 mg/L, most likely caused by nitrate reduction under anoxic conditions. Groundwater with reducing conditions (<5 mg/L dissolved oxygen) has an average nitrate content of about 7 mg/L, which is in contrast to groundwater with oxidising conditions that has 9 mg/L, which promotes the oxidation of ammonium to nitrate. The lowest nitrate concentrations are found in the forested area (Figs. 3c and S1c), where atmospheric nitrogen is held back by forest soils (U test – p value ; n=8), and fertilisation is prohibited due to water protection regulations in the forested area (Aber et al., 1998; Schönthaler and von Adrian-Werburg, 2008). Moreover, the average concentrations of iron and phosphate are low and, in most cases, below the detection limit of the test (Fig. S1d, e) and also below the natural and geogenic concentrations within the study site (phosphate – 0.05 mg/L; Griebler et al., 2014; iron – 3.3 mg/L; Kunkel et al., 2004).
Considering these findings, clear differences in the spatial distribution patterns of abiotic groundwater characteristics are noticeable. The forested area shows lower average GWT than the urban area (U test – p value ; n=8; 31), lower nitrate concentrations (U test – p value ; n=8; 31) and higher dissolved oxygen concentrations (U test – p value ; n=8; 31), which indicates a correlation between abiotic groundwater characteristics and land use in the study area. Moreover, no impact of groundwater originating from the urban area is observed on the wells in the forested area, as the groundwater flow direction in Karlsruhe is northwest (see Sect. 2.1 and Fig. 2c). Further investigations demonstrated that, besides one larger and two smaller contamination sites (still with concentrations below the threshold values, however; Fig. S1b), only minor groundwater pollution is documented in Karlsruhe (see the Supplement). The chemical and physical parameters considered in the long-term monitoring system are within the range of local background and below threshold values of the drinking water ordinance of Germany (see the Supplement for more information). Thus, the main documented impacts on groundwater quality in the study area are related to temperature and oxygen.
3.2 Groundwater fauna
The biotic communities of the groundwater consist of microorganisms and invertebrates (in particular crustaceans; Griebler et al., 2014). In the pool of samples, 3666 individuals were detected in 37 of 39 wells, which means that 95 % of the wells are colonised (Table S3). With 2047 individuals, the group of Crustacea was found to be the most abundant (56 %). A total of 976 individuals (27 %) of the order of Cyclopoida dominated this group, followed by the genus Parastenocaris, with 599 individuals (16 %), the order of Bathynellacea (371), Amphipoda (66), Harpacticoida (33) and nauplia. The communities of the monitoring wells also frequently contained oligochaetes (1343 individuals, 37 %). Furthermore, individuals of the phylum Nematoda (228 individuals) and microturbellaria (46 individuals) were also often present.
Overall, there is a noticeable difference in the spatial distribution of species within the study area. Individuals of the subphylum Crustacea were found in larger numbers, with respect to the number of wells, in the monitoring wells in the forested area (690 individuals in eight wells) compared to those in the urban area (1357 individuals in 31 wells). Furthermore, no individuals of the order Bathynellacea and only 135 individuals of the genus Parastenocaris were found in the forested area. In contrast, larger numbers of the latter species as well as of oligochaetes are characteristically found in the wells in the urban area. However, in contrast to the abiotic characteristics, no clear pattern of faunal diversity and land use was observed, as crustaceans and individuals of other subordinate taxa were found both in the forested and in the urban area.
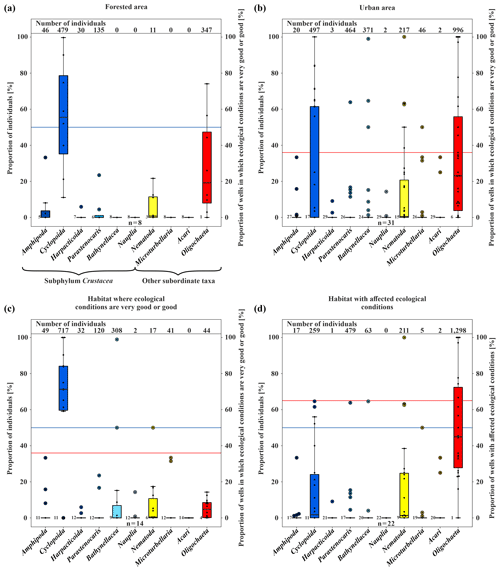
Figure 4Box plots of the amount of fauna (percent). (a) Proportion of individuals and wells in which ecological conditions are very good or good (secondary axis; percent) in the forested area. (b) Proportion of individuals and wells in which ecological conditions are very good or good (percent) in the urban area. (c) Proportion of individuals and wells in which ecological conditions are very good or good (percent), divided based on the results of the classification scheme by Griebler et al. (2014). (d) Proportion of individuals and wells with affected ecological conditions (percent), divided based on the results of the classification scheme by Griebler et al. (2014). The colour of the boxes shows the different taxa in the samples (n is the number of wells).
Stygobiotic amphipods, i.e. large-bodied invertebrates, which, due to their size, have a habitat preference for open spaces such as wells (Table 1; e.g. Hahn and Matzke, 2005; Korbel et al., 2017), were found in only three wells (Fig. 2b). A total of 46 individuals of this order were detected in the forest and 20 individuals in the urban area (Fig. 4a, b). Although statistical analysis showed no clear differences between the abundance of amphipods and land use (U test – p value ; n=8; 31), the higher number of individuals in the forest area could support the hypothesis that amphipods indicate healthy groundwater ecosystems as they react most sensitively to disturbances such as pollutants (Korbel and Hose, 2011) and groundwater temperature. In laboratory experiments with a thermal tank, Brielmann et al. (2011) found that 77 % of the individuals of the studied amphipods (Niphargus inopinatus) preferred areas with a temperature between 8 and 16 ∘C. In addition, Spengler (2017) and Issartel et al. (2005) observed maximum temperatures of up to 17 ∘C. The lack of a statistically significant correlation might also be related to the low number of wells (n =8 in the forested area) and individuals (n=46). Amphipods are important ecosystem service providers in terms of bioturbation and organic decomposition (Boulton et al., 2008). As observed in laboratory experiments (Smith et al., 2016), they actively move, with migration speeds between 1.7 and 3.5×104 m per year. In most cases when amphipods were found, higher concentrations of individuals of the order Cyclopoida were also identified (abundance of Amphipoda vs. Cyclopoida; U test – p value ; n=39). Individuals of the latter order were generally found in larger quantities in the majority of the wells (479 in the forested area and 497 in the urban area), as they are the largest group of crustaceans in this environment (Fuchs et al., 2006) and can tolerate a wide temperature range (e.g. upper thermal limit of 26.9±0.2 ∘C in laboratory tests by Castaño-Sánchez et al., 2020; Spengler, 2017).
The order Harpacticoida, which includes the genus Parastenocaris, have an elongated body shape and a stem-chiselling movement, which is why they are predestined for living in cavities and groundwater (Hahn, 1996; Fuchs, 2007) and prefer sand and gravel as a substrate (Galassi et al., 2009). Larger numbers of Parastenocaris (464 individuals), which can tolerate GWT from 8 to > 20 ∘C (Fuchs et al., 2006), e.g. Parastenocaris phyllura withstood up to 22.5 ∘C in laboratory tests (Glatzel, 1990), were found in the urban area, especially in the northwestern area (Fig. 2b). This area is characterised by GWTs between 16 and 18 ∘C, the highest at the study site. This observation is comparable with previous studies (Hahn, 2006; Hahn et al., 2013; Spengler, 2017), which showed that the genus Parastenocaris is particularly non-competitive and can often be found isolated in structurally burdened and physically and chemically altered areas. Accordingly, only 135 individuals were detected in the forested area.
In addition, quantities of Bathynellacea (371 individuals) were found in five monitoring wells all located in the urban area at a depth of 9.0 to 13.5 m with a GWT of 12–15 ∘C (Fig. 4b). This order typically inhabits the interstitial groundwater, which is characterised by a dominant exchange with the surface water and high variations in GWT and can tolerate temperatures up to 18 ∘C (Stein et al., 2012). Interestingly, one location in the southern city area with 272 individuals is characterised by a high fluctuation in GWT (standard deviation of 3.4 ∘C) and a rather high nitrate content (8.3 mg/L) compared to wells in the forested area, which are both indications for a disturbed and stressed habitat.
Besides the group of crustaceans, oligochaetes, which can tolerate a wide temperature range, were also found in large abundance in the study site. A significant amount of the subclass Oligochaeta (996 individuals) was found in the urban area (Fig. 4b), compared to an overall number of 1343 individuals. In general, the number of oligochaetes is larger in locations with high GWT (12.6–17.3 ∘C) and nitrate concentrations up to 14 mg/L, which is above the geogenic concentration of 10 mg/L and higher compared to wells in the forested area.
Finally, nematodes and microturbellarians were found at locations with unfavourable living conditions, such as a low dissolved oxygen content or a high amount of fine substrates, as also reported by Hahn et al. (2013), and both can tolerate high temperature ranges (Turbellaria – 2–20 ∘C; Herrmann, 1985; Acari – 9.1–18.5 ∘C; Więcek et al., 2013). Here, both were found in larger quantities in the urban area of Karlsruhe (Fig. 4b). This area has the lowest content of dissolved oxygen and a relatively higher amount of detritus (>2).
Eventually, correlation analysis between groundwater fauna and the chemical parameters showed that stygobites are only slightly affected by groundwater chemistry (Hahn, 2006; Schmidt et al., 2007; Stein et al., 2010). Only the Spearman rank correlation coefficient ρ between the number of taxa and the dissolved oxygen content is significant, with a value of ρ=0.55 (p value ; n=39). Moreover, it is assumed that groundwater fauna can usually cope well with short-term changes in physical–chemical parameters (Griebler et al., 2016). Previous studies showed that some species can even benefit from pollutants (Matzke, 2006; Zuurbier et al., 2013). In case of nitrate, numerous studies emphasise that nitrate at concentrations below 50 mg/L does not directly affect groundwater fauna (Fakher el Abiari et al., 1998; Mösslacher and Notenboom, 2000; Di Lorenzo and Galassi, 2013; Di Lorenzo et al., 2020a). As the highest average nitrate content per well is below 15 mg/L in this study, a direct negative effect of the nitrate concentration on the groundwater fauna is unlikely. Thus, nitrate is only mentioned as one measured parameter and is not discussed as a potential anthropogenic impact in this study.
The natural influences on porosity, groundwater flow and nutrient delivery were also discussed as being a primary influence on natural stygobite distribution in previous studies (Hahn, 2006; Korbel and Hose, 2015). An important natural influence is the local geology, as fine sands and silts are typically rather harsh environments, resulting in an impoverishment of specific groundwater fauna such as Crustacea (Hahn, 1996). The city of Karlsruhe is located on carbonate (Würm) gravel and river terrace sands, pervaded by bands of drifting sand and inland dune sands. These sediments are highly water permeable and show vertical seepage of water movement almost exclusively. Flood sediments (on top of river gravel) and bog formations, are located in the east and west of Karlsruhe (Regierungspräsidium Freiburg, 2019). This local geology limits the cavity size and, therefore, has impacts on the habitat of the groundwater fauna (Wirsing and Luz, 2007). For example, individuals of the genus Parastenocaris typically inhabit small-scale cavity systems (Spengler, 2017). Individuals of this genus can be found both in the wells drilled in gravel (four wells) and in drifting sand sediments (three wells; abundance of Parastenocaris vs. geological units; U test – p value ; n=39). Amphipods are predominantly found in measurement wells located in the Würm gravel (in five of seven wells; abundance of Amphipoda vs. geological units; U test – p value ; n=39). Moreover, it seems that differences in the geological units have an influence on the total amount of individuals (U test – p value ; n=39) and the relative amount of detritus (U test – p value ; n=39). As these results show, regional geology seems to have an influence on the occurrence of specific groundwater taxa and on the number of individuals and on food supply, in terms of available organic matter. However, it is not possible to give a reliable estimate of the strength of the anthropogenic impacts, e.g. if they are strong enough to overrule the regional selective forces. Hence, this should be investigated in more detail in future studies.
Limitations regarding the sampling method must be considered when interpreting the faunistic results. In this study, a simple basic screening of well water was conducted using a net sampler and bailer to examine conditions in the groundwater monitoring wells (39 wells with an average diameter of 132.5 mm, which corresponds to an area of 0.003 ‰ of the total urban area). According to the sampling manual of the PASCALIS project, “the use of a phreatobiological net alone is considered as [being] a satisfactory method for sampling groundwater fauna in large diameter wells” (Malard et al., 2002). Yet, several studies (e.g. Scheytt, 2014) report that scooped samples of wells are not representative, and therefore, the water remaining in a well has to be purged and discarded before sampling. Nevertheless, pumping can result in the selection of the taxa, especially in the presence of very fine sediments, and can result in changes in the sediment composition in the surrounding of the wells and, therefore, in habitat conditions. Other studies, on the other hand, found no significant differences in hydro-chemical values (temperature, pH, dissolved oxygen, etc.) between the surrounding groundwater and the standing water in a well (Hahn and Matzke, 2005; Korbel et al., 2017). The sampled groundwater fauna of corresponding wells and aquifers were also shown to be similar with respect to the types of faunal communities. However, in terms of total abundance, and the numbers of individuals per litre, monitoring wells appear to exhibit larger numbers caused by filtration effects (Hahn and Matzke, 2005; Hahn and Gutjahr, 2014; Korbel et al., 2017). As the aim of this study is to provide an overview of the groundwater fauna community and to receive a first impression of groundwater ecology, sampling the fauna by using a net sampler is sufficient. In order to achieve a representative sampling of groundwater fauna in the aquifer and to reflect the occurring species at a community level, a more comprehensive sampling method is required, e.g. the use of a defined standard sampling method, using a pump, to collect animals (Malard et al., 2002). Care should also be taken when interpreting faunistic results of sites that are sampled in different years. To improve comparisons of the biotic communities, a consistent sampling period of every well is necessary in the future.
3.3 Classification scheme by Griebler et al. (2014)
In three wells, evaluation with the classification scheme by Griebler et al. (2014) was not possible due to ocherous conditions in two monitoring wells and low dissolved oxygen content (<1 mg/L) in the third well. According to the classification scheme by Griebler et al. (2014), unstressed (meaning no natural or anthropogenic stressors) or natural groundwater habitats have more than 70 % of crustaceans and less than 20 % of oligochaetes. In 36 % of the sampled wells, i.e. 14 out of 39, these criteria were fulfilled, indicating very good or good ecological conditions or, in other words, a natural groundwater habitat (Fig. 4c). These natural areas tend to contain more individuals of the orders of Amphipoda, Cyclopoida and Bathynellacea. Monitoring wells, which do not fulfil these criteria and are accordingly defined as affected areas not having natural ecological conditions, contain more oligochaetes and also nematodes, which is partly explained by the criteria of this classification scheme (Fig. 4d).
Surprisingly, only 50 % of the wells in the forest, which is also the catchment area of the drinking water supply of Karlsruhe, are described as being natural groundwater habitats. An identical number of wells yielded habitats with affected ecological conditions. The main difference between natural and affected wells in the forested area arises from the occurrence of specific species. A total of 86 %–100 % of species found in natural wells are crustaceans, in contrast to affected wells with only 33 %–67 % (Tables S2 and S3). However, the abiotic parameters scarcely differ between natural and affected wells (average values for GWT – 10.8 and 10.6 ∘C; dissolved oxygen – 7.1 and 8.8 mg/L; nitrate – 2.5 and 3.0 mg/L), indicating that there are other processes or parameters that influence the groundwater fauna in these wells. A reason could be the varying local geology, as mentioned above. Moreover, food supply is one of the most limiting parameters for the survival of groundwater fauna (Datry et al., 2005; Hahn, 2006). If the organic carbon supply varies on a small scale, this can influence the microbiology and, therefore, the groundwater fauna as well, although short-term changes in nutrient supply can be compensated by groundwater fauna.
In contrast to the forest land, the majority of wells (65 %) in the urban area are categorised as affected habitats. As expected, this indicates anthropogenically influenced groundwater ecosystems beneath the studied urban area. Once more, no significant differences between the abiotic parameters of natural and affected wells are observed (e.g. median of dissolved oxygen – 4.7 and 5.8 mg/L; median of nitrate – 7.2 and 7.8 mg/L). On the other hand, the remaining 35 % of the wells in the urban area show natural ecological conditions even though some of them are located in areas with anthropogenic impacts such as increased groundwater temperatures. Hence, no distinct spatial pattern of the ecological condition with respect to land use could be identified.
In future, a further subdivision of a study area in more land use categories could be beneficial for specifically looking at typical anthropogenic impacts. Furthermore, the integration of more biological criteria is useful to improve the results of the assessment, according to Griebler et al. (2014). Because of heterogeneous groundwater ecosystems in Germany, it is likely that the reference values provided by Griebler et al. (2014) do not reflect the situation in Karlsruhe correctly. Considering site-specific characteristics and reference values would lead to a more robust assessment. Other assessments, like the similarly structured GHI or wGHIN (Korbel and Hose, 2017; Di Lorenzo et al., 2020b) can, additionally, be used. Moreover, there are a few newly developed indexes, like the D–A–C (prokaryotic cell density – D; activity – A; bioavailable carbon – C) Index, which is based on microbiological indicators and shows whether groundwater reserves deviate from natural references (Fillinger et al., 2019), which can be used in the future. As mentioned in the introduction, another way of quantifying the relevant ecological conditions in the groundwater is the GFI. During the preparation of this study, the GFI was tested on the data (see the Supplement); however, it did not provide any additional information or valuable insights and was therefore excluded. The influence of multiple stressors, such as the pollution of the groundwater through industrial plants, etc., and their effects on the governing parameters can bias the GFI. In general, the GFI seems to be suitable only for unpolluted and anthropogenically undisturbed groundwater with sufficient oxygen concentrations (>1 mg/L). Moreover, under urban areas, changes in GWT are caused by anthropogenic heat inputs (Menberg et al., 2013a, b; Benz et al., 2014; Tissen et al., 2018) rather than being related to surface water influences. Hence, the GFI appears to be unsuitable for the assessment of the groundwater fauna in an urban setting. The same outcome emerges for the Shannon diversity index, which was also tested during the preparation of the study and showed no clear distribution pattern according to faunal diversity and was therefore not considered further.
3.4 PHATE analysis
A PHATE analysis is conducted using the following input parameters: depth, GWT, nitrate and phosphate content, the relative amount of detritus, geological unit, number of taxa, number of individuals, Shannon diversity, number of crustaceans and oligochaetes (according to Griebler et al., 2014) and the abundance of amphipods as well as of individuals of the orders Cyclopoida and Bathynellacea and the genus Parastenocaris. The content of dissolved oxygen is not considered in this analysis since it was always above the limit of 1 mg/L, except in one case. Thus, dissolved oxygen is not expected to have an influence on the groundwater fauna in our study area.
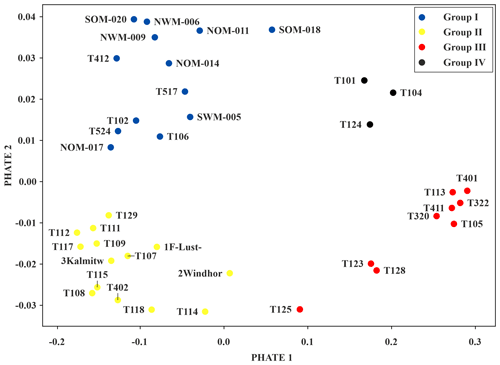
Figure 5PHATE visualisation showing similarities between measurement wells. Different colours indicate the four clearly separable groups.
There are four groups, which can be assigned predominant characteristics, that can be distinguished in the PHATE visualisation (Figs. 5 and S3–S4). A total of three measurement wells (group IV) contain neither oligochaetes nor crustaceans, indicating unfavourable living conditions. In contrast, the nine wells of group III contain high amounts of oligochaetes (100 % of oligochaetes according to the scheme of Griebler et al., 2014, and an average GWT of 14.3 ∘C; Table S4). However, diversity and abundance was found to be low in group III.
An even higher average GWT of 15.0 ∘C was found for group II, which mostly consists of wells drilled in drifting sand sediments. Surprisingly, these wells also show the highest diversity (≥ three taxa per well), the highest Shannon diversity (see the Supplement), and the highest number of individuals in total and of individuals of the genus Parastenocaris. Individuals of this genus are often found isolated in altered areas (Spengler, 2017). Moreover, in five wells of group II, individuals of the order Bathynellacea, which can tolerate temperatures up to 18 ∘C and typically inhabit interstitial groundwater (Stein et al., 2012), were found. The presence of individuals of the genus Parastenocaris and the order Bathynellacea in group II suggests that they may act as type species for urban situations. The observation that group II shows the highest GWT and the highest Shannon diversity is in contrast to findings of previous studies that noticed decreased diversity at elevated temperatures (Brielmann et al., 2009). These diverging observations suggest that faunal quantities, such as diversity or abundance, are not always suitable indicators for changes within organism communities. For example, if species disappear due to increased temperatures and are substituted by more tolerant species, the difference in diversity may be marginal and the change in the community may not be noticeable.
Wells of group I (blue) are drilled predominantly in Würm gravel (geological unit of group I vs. group II; U test – p value ,; n=13; 14), while having the lowest GWT (GWT of group I vs. group II; U test – p value ; n=13; 14). These wells show a moderate diversity and number of individuals, yet have the highest average number of crustaceans and the highest number of amphipods and individuals of the order Cyclopoida. Considering these findings and the U test results (see Table S5), the grouping of the measurement wells seems to be influenced by the composition of the groundwater organism communities, the faunal diversity (numbers of taxa and individuals) and the geological unit and the GWT (Figs. S3–S4).
Considering the spatial distribution of the grouped wells in the study area, it becomes apparent that all wells in the forested area fall within group I (Fig. 5). Those wells which are located outside the forested area are in locations with nearby green areas (parks, recreational areas, etc.). In contrast, the wells of the other three groups are heterogeneously distributed within the urban area. Many of the measurement wells of groups III and IV are associated with suspected or known contaminated sites (Fig. S1b). Overall, a spatial pattern of abiotic groundwater characteristics (GWT and nitrate content) and the occurrence of particular species (Parastenocaris) within the study area is apparent in the PHATE analysis, which confirms the classification according to land use. Yet again, no clear spatial pattern regarding faunal diversity in the study area could be identified, although a tendency of clustering of wells from group III with higher diversity and number of individuals can be seen in the northwestern area of the city.
The aim of this study is to provide a first assessment of the ecological state of groundwater in an urban area and to distinguish areas with a natural state of groundwater ecology from anthropogenically affected areas. To achieve this, we examine the groundwater fauna and abiotic parameters in 39 groundwater monitoring wells in residential, commercial and industrial areas (31 wells) and a forested area (eight wells) outside the built-up area of Karlsruhe, Germany, using the simple classification scheme by Griebler et al. (2014) to characterise the sampled monitoring wells.
We found a noticeable difference in the spatial distribution of abiotic groundwater characteristics and special species within the study area. The forested area shows lower GWT, lower nitrate concentrations and higher dissolved oxygen concentrations, which indicates a correlation between abiotic groundwater characteristics and land use. Moreover, amphipods are more abundant in wells in the forested than in urban area. However, in both the rural forested and in the urban area, crustaceans and individuals of other subordinate taxa were widely found, and therefore, no clear spatial pattern regarding faunal diversity and land use was found. In terms of faunal quantity, crustaceans were found in larger numbers, with respect to the number of wells, in the monitoring wells in the forested area compared to those in the urban area. Larger numbers of the genus Parastenocaris and of nematodes and oligochaetes were found to be characteristics for wells in the urban area.
Furthermore, no clear spatial pattern of ecological groundwater conditions, according to the classification scheme by Griebler et al. (2014), could be observed. Surprisingly, only 50 % of the sampled wells in the forested area were described as natural (undisturbed) groundwater habitats, while the other four were characterised as habitats with affected ecological conditions. Yet, the majority of wells (65 %) in the urban area were classified as affected locations, suggesting that there are noticeable differences in the groundwater ecosystems between the surrounding forested and urban areas. The level 2 assessment from Griebler et al. (2014) can help to achieve a more reliable and quantitative ecological assessment of urban aquifers as it divides groundwater ecosystems into ecological grades according to the intensity of anthropogenic disturbance. It is based on the use of local reference values and the collaboration with experts; however, is challenging to apply. Therefore, further studies with large-scale and repeated measurement campaigns are needed to verify our findings. This should also include other cities and the determination of undisturbed local reference values which are required for a more reliable, but also quantitative, ecological assessment of urban aquifers. Moreover, a wider range of indicators should be considered in a classification scheme, such as temperature, porosity of the aquifer, groundwater flow, pollutants and nutrient supply, especially when investigating urban areas. In addition, an important adaptation for an improved evaluation method is the determination of fauna at species level, which will provide more information (i.e. about stygobionts, stygophiles and stygoxenes) and also consider the endemism of stygobiotic species. In this context, classification schemes should pay more attention to the different groundwater species and their potential use as indicator species.
Finally, city and energy planning should seriously consider urban groundwater ecosystems as they provide valuable information for a sustainable use of the subsurface.
The code for the PHATE analysis is available at https://doi.org/10.5281/zenodo.4881204 (KIT-IngGeo, 2021) and https://github.com/KIT-IngGeo/Groundwater_Ecology (last access: 19 May 2021).
The access to the land cover data is provided by the European seamless vector data set of the CORINE Land Cover (CLC) inventory at https://land.copernicus.eu/pan-european/corine-land-cover/clc2018?tab=download (European Environment Agency, 2016). Results of the physical–chemical, faunistic and statistical analysis are available in the Supplement of this article.
The supplement related to this article is available online at: https://doi.org/10.5194/hess-25-3053-2021-supplement.
PB and HJH provided the topic and supervised the work, together with KM. SvS and CS executed the fieldwork and evaluated the samples. FK evaluated the collected data, interpreted and visualised the results and wrote the first draft of the paper. KM, CS, HJH and PB edited the paper.
The authors declare that they have no conflict of interest.
We would like to thank Annette März (Environmental Service, City of Karlsruhe), Michael Schönthal (Public Utilities, Karlsruhe) and Friedhelm Fischer (Civil Engineering Office of Karlsruhe). Special thanks to Christine Buschhaus and Tanja Liesch for their support with the measurement and sampling (Institute of Applied Geosciences, Karlsruhe Institute of Technology).
We acknowledge support from the KIT Publication Fund of the Karlsruhe Institute of Technology.
The article processing charges for this open-access publication were covered by the Karlsruhe Institute of Technology (KIT).
This paper was edited by Christine Stumpp and reviewed by two anonymous referees.
Aber, J., Mcdowell, W., Nadelhoffer, K., Magill, A., Berntson, G., Mcnulty, S., Currie, W., Rustad, L., and Fernandez, I.: Nitrogen saturation in temperate forest ecosystems – Hypotheses revisited, Bioscience, 48, 921–934, 1998.
Amt für Stadtentwicklung – Statistikstelle: Statistic Atlas Karlsruhe, available at: https://web3.karlsruhe.de/Stadtentwicklung/statistik/atlas/?select=005 (last access: 27 February 2019), 2018.
Batzer, D. and Boix, D.: Invertebrates in Freswater Wetlands: An International Perspective on their Ecology, Springer International Publishing, Heidelberg, 2016.
Benz, S., Bayer, P., Menberg, K., and Blum, P.: Comparison of local and regional heat transport processes into the subsurface urban heat island of Karlsruhe, Germany, Geophys. Res. Abstr. EGU Gen. Assem., 16, 11252, 2014.
Benz, S., Bayer, S., and Blum, P.: Identifying anthropogenic anomalies in air, surface and groundwater temperatures in Germany, Sci. Total Environ., 584–584, 145–153, 2017.
Benz, S. A., Bayer, P., Goettsche, F. M., Olesen, F. S., and Blum, P.: Linking Surface Urban Heat Islands with Groundwater Temperatures, Environ. Sci. Technol., 50, 70–78, https://doi.org/10.1021/acs.est.5b03672, 2016.
Berkhoff, S.: Die Meiofauna des Interstitials und Grundwassers als Indikator für Oberflächenwasser-Grundwasser-Interaktionen im Bereich einer Uferfiltrationsanlage, University Koblenz-Landau, Landau, available at: https://kola.opus.hbz-nrw.de/frontdoor/index/index/year/2010/docId/388 (last access: 19 May 2021), 2010.
Blum, P., Menberg, K., Koch, F., Benz, S. A., Tissen, C., Hemmerle, H., and Bayer, P.: Is thermal use of groundwater a pollution?, J. Contam. Hydrol., 239, 103791, https://doi.org/10.1016/j.jconhyd.2021.103791, 2021.
Botosaneanu, L.: Stygofauna mundi: a faunistic, distributional, and ecological synthesis of the world fauna inhabiting subterranean waters (including the marine interstitial), Leiden, The Netherlands, 1986.
Boulton, A. J., Fenwick, G. D., Hancock, P. J., and Harvey, M. S.: Biodiversity, functional roles and ecosystem services of groundwater invertebrates, Invertebr. Syst., 22, 103–116, https://doi.org/10.1071/IS07024, 2008.
Brielmann, H., Griebler, C., Schmidt, S. I., Michel, R., and Lueders, T.: Effects of thermal energy discharge on shallow groundwater ecosystems, FEMS Microbiol. Ecol., 68, 273–286, https://doi.org/10.1111/j.1574-6941.2009.00674.x, 2009.
Brielmann, H., Lueders, T., Schreglmann, K., Ferraro, F., Avramov, M., Hammerl, V., Blum, P., Bayer, P., and Griebler, C.: Oberflächennahe Geothermie und ihre potenziellen Auswirkungen auf Grundwasserökosysteme, Grundwasser, 16, 77–91, https://doi.org/10.1007/s00767-011-0166-9, 2011.
Camacho, A. I.: An annotated checklist of the Syncarida (Crustacea, Malacostraca) of the world, Zootaxa, 1374, 1–54, 2006.
Castaño-Sánchez, A., Hose, G. C., and Reboleira, A. S. P. S.: Salinity and temperature increase impact groundwater crustaceans, Sci. Rep., 10, 1–9, https://doi.org/10.1038/s41598-020-69050-7, 2020.
Datry, T., Malard, F., and Gibert, J.: Response of invertebrate assemblages to increased groundwater recharge rates in a phreatic aquifer, J. North Am. Benthol. Soc., 24, 461–477, https://doi.org/10.1899/04-140.1, 2005.
Deharveng, L., Stoch, F., Gibert, J., Bedos, A., Galassi, D. M. P., Zagmajster, M., Brancelj, A., Camacho, A. I., Fiers, F., Martin, P., Giani, N., Magniez, G., and Marmonier, P.: Groundwater biodiversity in Europe, Freshw. Biol., 54, 709–726, https://doi.org/10.1111/j.1365-2427.2008.01972.x, 2009.
Di Lorenzo, T. and Galassi, D. M. P.: Agricultural impact on Mediterranean alluvial aquifers: Do groundwater communities respond?, Fundam. Appl. Limnol., 182, 271–282, https://doi.org/10.1127/1863-9135/2013/0398, 2013.
Di Lorenzo, T., Fiasca, B., Di Cicco, M., and Galassi, D. M. P.: The impact of nitrate on the groundwater assemblages of European unconsolidated aquifers is likely less severe than expected, Environ. Sci. Pollut. Res., 28, 11518–11527, https://doi.org/10.1007/s11356-020-11408-5, 2020a.
Di Lorenzo, T., Fiasca, B., di Camillo Tabilio, A., Murolo, A., Di Cicco, M., and Galassi, D. M. P.: The weighted Groundwater Health Index (wGHI) by Korbel and Hose (2017) in European groundwater bodies in nitrate vulnerable zones, Ecol. Indic., 116, 11, https://doi.org/10.1016/j.ecolind.2020.106525, 2020b.
Dole-Olivier, M. J., Malard, F., Martin, D., Lefébure, T., and Gibert, J.: Relationships between environmental variables and groundwater biodiversity at the regional scale, Freshw. Biol., 54, 797–813, https://doi.org/10.1111/j.1365-2427.2009.02184.x, 2009a.
Dole-Olivier, M. J., Castellarini, F., Coineau, N., Galassi, D. M. P., Martin, P., Mori, N., Valdecasas, A., and Gibert, J.: Towards an optimal sampling strategy to assess groundwater biodiversity: Comparison across six European regions, Freshw. Biol., 54, 777–796, https://doi.org/10.1111/j.1365-2427.2008.02133.x, 2009b.
Eckert, J., Friedhoff, K. T., Zahner, H., and Deplazes, P.: Lehrbuch der Parasitologie für die Tiermedizin Teil II Parasiten und Parasitosen: 3 Metazoa, 2nd ed., Thieme Verlagsgruppe Stuttgart/Enke Verlag, Stuttgart, 2008.
Einsle, U.: Crustacea: Copepoda, Calanoida and Cyclopoida – Süßwasserfauna von Mitteleuropa, 8/4–1, Gustav Fischer Verlag Stuttgart, Stuttgart, 1993.
Fakher el Abiari, A., Oulbaz, Z., Yacoubi-Khebiza, M., Coineau, N., and Boutin, C.: Etude expérimentale de la sensibilité comparée de trois crustacés stygobies vis-à-vis de diverses substances toxiques pouvant se rencontrer dans les eaux souterraines, in: Mémoires de Biospéologie, volume 25, 167–181, 1998.
Fillinger, L., Hug, K., Trimbach, A. M., Wang, H., Kellermann, C., Meyer, A., Bendinger, B., and Griebler, C.: The D-A-(C) index: A practical approach towards the microbiological-ecological monitoring of groundwater ecosystems, Water Res., 163, 114902, https://doi.org/10.1016/j.watres.2019.114902, 2019.
Foulquier, A., Malard, F., Mermillod-Blondin, F., Montuelle, B., Dolédec, S., Volat, B., and Gibert, J.: Surface Water Linkages Regulate Trophic Interactions in a Groundwater Food Web, Ecosystems, 14, 1339–1353, https://doi.org/10.1007/s10021-011-9484-0, 2011.
Fuchs, A.: Erhebung und Beschreibung der Grundwasserfauna in Baden-Württemberg, 1–109, available at: https://kola.opus.hbz-nrw.de/frontdoor/index/index/docId/175 (last access: 15 April 2021), 2007.
Fuchs, A., Hahn, H. J., and Barufke, K. P.: Grundwasser-Überwachungsprogramm – Erhebung und Beschreibung der Grundwasserfauna in Baden-Württemberg, LUBW Landesanstalt für Umwelt, Messungen und Naturschutz Baden-Württemberg' in Karlsruhe, available at: https://pudi.lubw.de/detailseite/-/publication/77258 (12 May 2021), 2006.
Galassi, D. M. P.: Groundwater copepods: Diversity patterns over ecological and evolutionary scales, Hydrobiologia, 453–454, 227–253, https://doi.org/10.1023/A:1013100924948, 2001.
Galassi, D. M. P., Huys, R., and Reid, J. W.: Diversity, ecology and evolution of groundwater copepods, Freshw. Biol., 54, 691–708, https://doi.org/10.1111/j.1365-2427.2009.02185.x, 2009.
German Environment Agency: Bericht des Bundesministeriums für Gesundheit und des Umweltbundesamtes an die Verbraucherinnen und Verbraucher über die Qualität von Wasser für den menschlichen Gebrauch (Trinkwasser) in Deutschland 2014–2016, German Federal Ministry of Health, German Environment Agency (UBA), Dessau-Roßlau, available at: https://www.umweltbundesamt.de/publikationen/bericht-des-bundesministeriums-fuer-gesundheit-des-3 (last access: 12 May 2021), 2018.
Geyer, O. F., Gwinner, M. P., Nitsch, E., and Simon, T.: Geologie von Baden-Württemberg, Schweizerbart, Stuttgart, 2011.
Gibert, J. and Deharveng, L.: Subterranean Ecosystems: A Truncated Functional Biodiversity, Bioscience, 52, 473, https://doi.org/10.1641/0006-3568(2002)052[0473:seatfb]2.0.co;2, 2002.
Gibert, J., Culver, D. C., Dole-Olivier, M.-J., Malard, F., Christman, M. C., and Deharveng, L.: Assessing and conserving groundwater biodiversity: Synthesis and perspectives, Freshw. Biol., 54, 930–941, https://doi.org/10.1111/j.1365-2427.2009.02201.x, 2009.
European Environment Agency: Corine Land Cover (CLC) European seamless vector database, EEA/Copernicus, Kopenhagen, available at: https://land.copernicus.eu/pan-european/corine-land-cover/clc2018?tab=download (last access: 19 May 2021), 2016.
Glatzel, T.: On the biology of Parastenocaris phyllura Kiefer 1938 (Copepoda: Harpacticoida), Stygologia, 5, 131–136, 1990.
Griebler, C. and Avramov, M.: Groundwater ecosystem services: A review, Freshw. Sci., 34, 355–367, https://doi.org/10.1086/679903, 2015.
Griebler, C., Stein, H., Hahn, H. J., Steube, C., Kellemrann, C., Fuchs, A., Berkhoff, S., and Brielmann, H.: Entwicklung biologischer Bewertungsmethoden und -kriterien für Grundwasserökosysteme, Umweltbundesamt, Dessau, 2014.
Griebler, C., Brielmann, H., Haberer, C. M., Kaschuba, S., Kellermann, C., Stumpp, C., Hegler, F., Kuntz, D., Walker-Hertkorn, S., and Lueders, T.: Potential impacts of geothermal energy use and storage of heat on groundwater quality, biodiversity, and ecosystem processes, Environ. Earth Sci., 75, 1–18, https://doi.org/10.1007/s12665-016-6207-z, 2016.
Gutjahr, S.: Grundwasserlebensräume in der Landschaft – Untersuchungen zur Bedeutung von Hydrologie und Hydrogeologie für Grundwasserlebensgemeinschaften, Universität Koblenz-Landau, Landau, 2013.
Gutjahr, S., Schmidt, S. I., and Hahn, H. J.: A proposal for a groundwater habitat classification at local scale, Subterr. Biol., 14, 25–49, https://doi.org/10.3897/subtbiol.14.5429, 2014.
Hahn, H. J.: Die Ökologie der Sedimente eines Buntsandsteinbaches im Pfälzerwald unter besonderer Berücksichtigung der Ostracoden und Harpacticoiden (Crustacea), 62nd ed., Tectum-Verlag, Marburg, 1996.
Hahn, H. J.: Unbaited phreatic traps: A new method of sampling stygofauna, Limnologica, 35, 248–261, https://doi.org/10.1016/j.limno.2005.04.004, 2005.
Hahn, H. J.: A first approach to a quantitative ecological assessment of groundwater habitats: The GW-Fauna-Index, Limnologica, 36, 119–137, 2006.
Hahn, H. J. and Fuchs, A.: Distribution patterns of groundwater communities across aquifer types in south-western Germany, Freshw. Biol., 54, 848–860, https://doi.org/10.1111/j.1365-2427.2008.02132.x, 2009.
Hahn, H. J. and Gutjahr, S.: Bioindikation im Grundwasser funktioniert – Erwiderung zum Kommentar von T. Scheytt zum Beitrag „Grundwasserfauna als Indikator für komplexe hydrogeologische Verhältnisse am westlichen Kaiserstuhl“ von Gutjahr, S., Bork, J., and Hahn, H. J. in Grundwasser 18, Grundwasser, 19, 215–218, https://doi.org/10.1007/s00767-014-0266-4, 2014.
Hahn, H. J. and Matzke, D.: A comparison of stygofauna communities inside and outside groundwater bores, Limologica, 35, 31–44, 2005.
Hahn, H. J., Matzke, D., Kolberg, A., and Limberg, A.: Untersuchung zur Fauna des Berliner Grundwassers – erste Ergebnisse, Brandenburgische Geowissenschaftliche Beiträge by the LBGR (Landesamt für Bergbau, Geologie und Rohstoffe Brandenburg), Cottbus, 20, 85–92, 2013.
Hahn, H. J., Schweer, C., and Griebler, C.: Are groundwater ecosystem rights being preserved?: A critical evaluation of the legal background of groundwater ecosystems, Grundwasser, 23, 209–218, https://doi.org/10.1007/s00767-018-0394-3, 2018.
Hähnlein, S., Bayer, P., and Blum, P.: International legal status of the use of shallow geothermal energy, Renew. Sustain. Energy Rev., 14, 2611–2625, https://doi.org/10.1016/j.rser.2010.07.069, 2010.
Hähnlein, S., Bayer, P., Ferguson, G., and Blum, P.: Sustainability and policy for the thermal use of shallow geothermal energy, Energy Policy, 59, 914–925, https://doi.org/10.1016/j.enpol.2013.04.040, 2013.
Hancock, P. J., Boulton, A. J., and Humphreys, W. F.: Aquifers and hyporheic zones: Towards an ecological understanding of groundwater, Hydrogeol. J., 13, 98–111, https://doi.org/10.1007/s10040-004-0421-6, 2005.
Herrmann, J.: Dependence of Reproduction in Dendrocoelum lacteum (Turbellaria): An Experimental Approach, Oikos, 44, 268–272, 1985.
Hunkeler, D., Goldscheider, N., Rossi, P., and Burn, C.: Biozönosen im Grundwasser – Grundlagen und Methoden der Charakterisierung von mikrobiellen Gemeinschaften, Umwelt-Wissen No. 0603, Bundesamt für Umwelt (BAFU), Bern, 2006.
Issartel, J., Hervant, F., Voituron, Y., Renault, D., and Vernon, P.: Behavioural, ventilatory and respiratory responses of epigean and hypogean crustaceans to different temperatures, Comp. Biochem. Physiol. Part A, 141, 1–7, https://doi.org/10.1016/j.cbpb.2005.02.013, 2005.
Janetzka, W., Enderle, R., and Noodt, W.: Crustacea: Copepoda: Gelyelloida and Harpacticoida – Süßwasserfauna von Mitteleuropa, 8/4–2, Gustav Fischer Verlag Stuttgart, Stuttgart, 1996.
KIT-IngGeo, KIT-IngGeo/Groundwater_Ecology: first release (Version v1.0.0), Zenodo, https://doi.org/10.5281/zenodo.4881204, last access: 31 May 2021.
Korbel, K., Chariton, A., Stephenson, S., Greenfield, P., and Hose, G. C.: Wells provide a distorted view of life in the aquifer: Implications for sampling, monitoring and assessment of groundwater ecosystems, Sci. Rep., 7, 1–14, https://doi.org/10.1038/srep40702, 2017.
Korbel, K. L. and Hose, G. C.: A tiered framework for assessing groundwater ecosystem health, Hydrobiologia, 661, 329–349, https://doi.org/10.1007/s10750-010-0541-z, 2011.
Korbel, K. L. and Hose, G. C.: Habitat, water quality, seasonality, or site? Identifying environmental correlates of the distribution of groundwater biota, Freshw. Sci., 34, 329–342, https://doi.org/10.1086/680038, 2015.
Korbel, K. L. and Hose, G. C.: The weighted groundwater health index: Improving the monitoring and management of groundwater resources, Ecol. Indic., 75, 164–181, https://doi.org/10.1016/j.ecolind.2016.11.039, 2017.
Kühlers, D., Maier, M., and Roth, K.: Sanierung im Verborgenen, TerraTech Sanierungspraxis, 3, 14–16, 2012.
Kunkel, R., Wendland, F., and Hannappel, S.: Die natürliche, ubiquitär überprägte Grundwasserbeschaffenheit in Deutschland, 47th ed., Schriften des Forschungszentrums Jülich, Forschungszentrum Jülich GmbH, Jülich, 2004.
Malard, F., Dole-Olivier, M.-J., Mathieu, J., Stoch, F., Boutin, C., Brancelj, A., Camacho, A. I., Fiers, F., Galassi, D., Gibert, J., Lefebure, T., Martin, P., Sket, B., and Valdecasas, A. G.: Sampling Manual for the Assessment of Regional Groundwater Biodiversity, available at: http://www.eugris.info/displayresource.aspx?r=5247 (last access: 12 May 2021), 2002.
Matzke, D.: Untersuchungen zum Verhalten von Grundwasserfauna in Altlastflächen mit vorangegangenem Vergleich unterschiedlicher Sammeltechniken, University of Koblenz-Landau, Landau, available at: https://kola.opus.hbz-nrw.de/frontdoor/index/index/year/2006/docId/12 (last access: 12 May 2021), 2006.
Meisch, C.: Freshwater Ostracoda of Western and Central Europe - Süßwasserfauna von Mitteleuropa, 8/3, Spektrum Akademischer Verlag, Heidelberg, 2000.
Menberg, K., Blum, P., Schaffitel, A., and Bayer, P.: Long-term evolution of anthropogenic heat fluxes into a subsurface urban heat island, Environ. Sci. Technol., 47, 9747–9755, https://doi.org/10.1021/es401546u, 2013a.
Menberg, K., Bayer, P., Zosseder, K., Rumohr, S., and Blum, P.: Subsurface urban heat islands in German cities, Sci. Total Environ., 442, 123–133, https://doi.org/10.1016/j.scitotenv.2012.10.043, 2013b.
Moon, K. R., van Dijk, D., Wang, Z., Gigante, S., Burkhardt, D. B., Chen, W. S., Yim, K., Elzen, A. van den, Hirn, M. J., Coifman, R. R., Ivanova, N. B., Wolf, G., and Krishnaswamy, S.: Visualizing structure and transitions in high-dimensional biological data, Nat. Biotechnol., 37, 1482–1492, https://doi.org/10.1038/s41587-019-0336-3, 2019.
Mösslacher, F. and Notenboom, J.: Groundwater biomonitoring, in Biomonitoring of polluted water, edited by: Gerhardt, A., 119–139, Trans Tech Publications Ltd, Uetikon, Zurich, 2000.
Preuß, G. and Schminke, H. K.: Ein globales ökosystem: Grundwasser lebt!, Chemie Unserer Zeit, 38, 340–347, https://doi.org/10.1002/ciuz.200400307, 2004.
Regierungspräsidium Freiburg: LGRB-Kartenviewer – Layer GK50: Geologische Einheiten (Flächen), available at: https://maps.lgrb-bw.de/ (last access: 6 July 2020), 2019.
Di Sabatino, A., Gerecke, R., and Martin, P.: The biology and ecology of lotic water mites (Hydrachnidia), Freshw. Biol., 44, 47–62, 2000.
Sauermost, R. and Freudig, D.: Bathynellacea, Spektrum Akad. Verlag, Heidelberg, available at: https://www.spektrum.de/lexikon/biologie/bathynellacea/7445 (last access: 27 February 2019), 1999.
Sauermost, R. and Freudig, D.: Oligochaeta, Spektrum Akad. Verlag, Heidelberg, available at: https://www.spektrum.de/lexikon/biologie/oligochaeta/47593 (last access: 27 February 2019), 1999.
Sauermost, R. and Freudig, D.: Strudelwürmer, Spektrum Akad. Verlag, Heidelberg, available at: https://www.spektrum.de/lexikon/biologie/strudelwuermer/64369 (last access: 27 February 2019), 1999.
Schellenberg, A.: Krebstiere oder Crustace, IV: Flohkebs oder Amphipoda, in: Die Tierwelt Deutschlands und der angrenzenden Meeresteile nach ihren Merkmalen und nach ihrer Lebensweise, p. 252, Gustav Fischer Verlag, Jena, 1942.
Scheytt, T.: Kommentar zur Veröffentlichung von Gutjahr, S., Bork, J. und Hahn, H. J.: Grundwasserfauna als Indikator für komplexe hydrogeologische Verhältnisse am westlichen Kaiserstuhl in Grundwasser 18, 173–184 (2013), Grundwasser, 19, 211–213, https://doi.org/10.1007/s00767-014-0267-3, 2014.
Schmidt, S. I., Hahn, H. J., Hatton, T. J., and Humphreys, W. F.: Do faunal assemblages reflect the exchange intensity in groundwater zones?, Hydrobiologia, 583, 1–19, https://doi.org/10.1007/s10750-006-0405-8, 2007.
Schminke, H. K., Grad, G., Ahlrichs, W., Bartsch, I., Christl, H., Gerecke, R., Martin, P., Rumm, P., and Wägele, J. W.: Grundwasserfauna Deutschlands – Ein Bestimmungswerk: DWA-Themen, 1st ed., Deutsche Vereinigung für Wasserwirtschaft, Abwasser und Abfall, Hennef, 2007.
Schönthaler, K. and von Adrian-Werburg, S.: Erster integrierter Umweltbericht für das länderübergreifende UNESCO-Biosphärenreservat Rhön, Bayerisches Staatsministerium für Umwelt, Gesundheit und Verbraucherschutz (BayStMUGV) Hessisches Ministerium für Umwelt, ländlichen Raum und Verbraucherschutz (HMULV) Thüringer Ministerium für Landwirtschaft, Naturschutz und Umwelt (TMLNU), 2008.
Smith, R. J., Paterson, J. S., Launer, E., Tobe, S. S., Morello, E., Leijs, R., Marri, S., and Mitchell, J. G.: Stygofauna enhance prokaryotic transport in groundwater ecosystems, Sci. Rep., 6, 1–7, https://doi.org/10.1038/srep32738, 2016.
Spengler, C.: Die Auswirkungen von anthropogenen Temperaturerhöhungen auf die Crustaceagemeinschaften im Grundwasser, Universität Koblenz-Landau, Landau, available at: https://kola.opus.hbz-nrw.de/frontdoor/index/index/docId/1554 (last access: 19 May 2021), 2017.
Spengler, C. and Hahn, J.: Thermostress: Ökologisch begründete, thermische Schwellenwerte und Be- wertungsansätze für das Grundwasser, Korrespondenz Wasserwirtschaft Fachbeiträge Gewässer und Böden, 11, 521–525, https://doi.org/10.3243/kwe2018.09.001, 2018.
Stadt Karlsruhe: Bodenschutz- und Altlastenkataster der Stadt Karlsruhe, available at: https://www.karlsruhe.de/b3/natur_und_umwelt/umweltschutz/altlasten.de (last access: 23 October 2019), 2006.
Stein, H., Kellermann, C., Schmidt, S. I., Brielmann, H., Steube, C., Berkhoff, S. E., Fuchs, A., Hahn, H. J., Thulin, B., and Griebler, C.: The potential use of fauna and bacteria as ecological indicators for the assessment of groundwater quality, J. Environ. Monit., 12, 242–254, https://doi.org/10.1039/b913484k, 2010.
Stein, H., Griebler, C., Berkhoff, S., Matzke, D., Fuchs, A., and Hahn, H. J.: Stygoregions-a promising approach to a bioregional classification of groundwater systems, Sci. Rep., 2, 1–9, https://doi.org/10.1038/srep00673, 2012.
Stoch, F., Artheau, M., Brancelj, A., Galassi, D. M. P., and Malard, F.: Biodiversity indicators in European ground waters: Towards a predictive model of stygobiotic species richness, Freshw. Biol., 54, 745–755, https://doi.org/10.1111/j.1365-2427.2008.02143.x, 2009.
Taylor, C. A. and Stefan, H. G.: Shallow groundwater temperature response to climate change and urbanization, J. Hydrol., 375, 601–612, https://doi.org/10.1016/j.jhydrol.2009.07.009, 2009.
Technologiezentrum Wasser: Grundwasserdatenbank Wasserversorgung: Regionale Auswertung – Region Mittlerer Oberrhein, available at: http://www.grundwasserdatenbank.de/regionmo.htm (last access: 27 February 2019), 2018.
Tissen, C., Menberg, K., Bayer, P., and Blum, P.: Heat supply by shallow geothermal energy in Karlsruhe, in Groundwater in the surrounding of mining, energy and urban space, Conference of the professional division Hydrogeology in the DGGV, Bochum, 21–24 March 2018, 2018.
Tissen, C., Benz, S. A., Menberg, K., Bayer, P., and Blum, P.: Groundwater temperature anomalies in central Europe, Environ. Res. Lett., 14, 104012, https://doi.org/10.1088/1748-9326/ab4240, 2019.
Ward, J. V. and Tockner, K.: Biodiversity: Towards a unifying theme for river ecology, Freshw. Biol., 46, 807–819, https://doi.org/10.1046/j.1365-2427.2001.00713.x, 2001.
Wickert, F., Müller, A., Schäfer, W., and Tiehm, A.: Vergleich hochauflösender Grundwasserprobennahmeverfahren zur Charakterisierung der vertikalen LCKW-Verteilung im Grundwasserleiter, Altlastenspektrum, 01, 29–35, 2006.
Więcek, M., Martin, P., and Gąbka, M.: Distribution patterns and environmental correlates of water mites (Hydrachnidia, Acari) in peatland microhabitats, Exp. Appl. Acarol., 61, 147–160, https://doi.org/10.1007/s10493-013-9692-8, 2013.
Wirsing, G. and Luz, A.: Hydrogeologischer Bau und Aquifereigenschaften der Lockergesteine im Oberrheingraben (Baden Württemberg), LGRB-Informationen, 19, 130, Regierungspräsidium Freiburg, Freiburg, 2007.
Zenker, S., Bogon, K., and Weigand, A.: Die Höhlentiere Deutschlands, Quelle & Meyer Verlag, Wiebelsheim, 2020.
Zhu, K., Blum, P., Ferguson, G., Balke, K. D., and Bayer, P.: The geothermal potential of urban heat Islands, Environ. Res. Lett., 5, 1–6, https://doi.org/10.1088/1748-9326/6/1/019501, 2010.
Zuurbier, K. G., Hartog, N., Valstar, J., Post, V. E. A., and Van Breukelen, B. M.: The impact of low-temperature seasonal aquifer thermal energy storage (SATES) systems on chlorinated solvent contaminated groundwater: Modeling of spreading and degradation, J. Contam. Hydrol., 147, 1–13, https://doi.org/10.1016/j.jconhyd.2013.01.002, 2013.