the Creative Commons Attribution 4.0 License.
the Creative Commons Attribution 4.0 License.
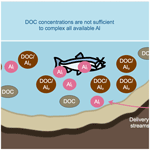
Ionic aluminium concentrations exceed thresholds for aquatic health in Nova Scotian rivers, even during conditions of high dissolved organic carbon and low flow
Shannon M. Sterling
Sarah MacLeod
Lobke Rotteveel
Kristin Hart
Thomas A. Clair
Edmund A. Halfyard
Nicole L. O'Brien
Acid deposition released large amounts of aluminium into streams and lakes during the last century in northern Europe and eastern North America. Elevated aluminium concentrations caused major environmental concern due to aluminium's toxicity to terrestrial and aquatic organisms and led to the extirpation of wild Atlantic salmon populations. Air pollution reduction legislation that began in the 1990s in North America and Europe successfully reduced acid deposition, and the aluminium problem was widely considered solved. However, accumulating evidence indicates that freshwater systems still show delays in recovery from acidification, with poorly understood implications for aluminium concentrations. Here, we investigate spatial and temporal patterns of labile cationic forms of aluminium (Ali) from 2015 to 2018 in 10 catchments in Nova Scotia, Canada; this region was one of the hardest hit by acid deposition, although it was not considered to have an aluminium problem due to its high dissolved organic carbon (DOC) concentrations that were expected to reduce Ali concentrations. Surprisingly, our results show the widespread and frequent occurrences of Ali concentrations that exceed toxic thresholds in all sampled rivers despite high DOC concentrations. Generalized linear mixed model results reveal that DOC, instead of being inversely related to Ali, is the strongest predictor (positive) of Ali concentrations, suggesting that the recruitment properties of DOC in soils outweigh its protective properties in streams. Lastly, we find that, contrary to the common conceptualization that high Ali levels are associated with storm flow, high Ali concentrations are found during base flow. Our results demonstrate that elevated Ali concentrations in Nova Scotia continue to pose a threat to aquatic organisms, such as the biologically, economically, and culturally significant Atlantic salmon (Salmo salar).
- Article
(5214 KB) -
Supplement
(1226 KB) - BibTeX
- EndNote
Increased rates of acid deposition, predominantly deriving from upwind fossil fuel burning, resulted in the acidification of soils, rivers, and lakes during the last century (e.g., Kerekes et al., 1986), depleting base cations and increasing toxic aluminium concentrations in soils and drainage water. Increased aluminium concentrations caused the extirpation of native Atlantic salmon (Salmo salar) populations in many rivers (Rosseland et al., 1990), for example in Scandinavia (Henriksen et al., 1984; Hesthagen and Hansen, 1991), the eastern USA (Monette and McCormick, 2008; Parrish et al., 1998), and Nova Scotia, Canada (Watt, 1987). The acidification problem was widely considered solved following reductions in anthropogenic sulfur emissions in North America and Europe since the 1990s. Many rivers showed steady improvements in annual average stream chemistry (Evans et al., 2001; Monteith et al., 2014; Skjelkvåle et al., 2005; Stoddard et al., 1999; Warby et al., 2005), including reduced concentrations of aluminium (Al) in the USA (Baldigo and Lawrence, 2000; Buchanan et al., 2017; Burns et al., 2006) and Europe (Beneš et al., 2017; Davies et al., 2005; Monteith et al., 2014). However, recent evidence highlights delayed recovery from acidification in other regions (Houle et al., 2006; Warby et al., 2009; Watmough et al., 2016), including Nova Scotia (Clair et al., 2011), raising questions about the possibility of elevated aluminium concentrations in freshwater systems.
Al in freshwater can exist as inorganic monomers, inorganic polymers, in amorphous and microcrystalline inorganic forms, and in fast reactive or unreactive organic forms (Chew et al., 1988; Driscoll et al., 1980; LaZerte, 1984). While a variety of Al species in circumneutral waters are toxic to fish (Gensemer and Playle, 1999), including precipitated forms (Gensemer et al., 2018), the cationic species of Al (Ali), such as Al3+, Al(OH), and Al(OH)2+, are considered to be the most labile and toxic to salmonids. Al species bind to the negatively charged fish gills and cause morbidity and mortality through suffocation (Exley et al., 1991), reduce nutrient intake at gill sites, and alter blood plasma levels (Nilsen et al., 2010). The effects of sublethal exposure to freshwater Al elicit osmoregulatory impairment (Monette and McCormick, 2008; Regish et al., 2018), which reduces survival in the hypertonic marine environment (McCormick et al., 2009; Staurnes et al., 1996). Elevated concentrations of Ali are also toxic to other freshwater and terrestrial organisms (Boudot et al., 1994; Wauer and Teien, 2010), such as frogs and aquatic birds (Lacoul et al., 2011).
Al speciation varies with pH (Helliweli et al., 1983; Lydersen, 1990): positive Al species dominate over neutral and negative species below pH 6.3 at 2 ∘C and below pH 5.7 at 25 ∘C (Lydersen, 1990), and the most toxic Al species, Al(OH) (Helliweli et al., 1983), dominates Al speciation between pH 5.0 and 6.0 at 25 ∘C, and between 5.5 and 6.5 at 2 ∘C (Lydersen, 1990). Thus, the toxicity of Al increases with increasing pH up to approximately 6.0 at 25 ∘C or 6.5 at 5 ∘C, when aqueous aluminium precipitates, forming gibbsite (Lydersen, 1990; Schofield and Trojnar, 1980); colder water will have a higher proportion of toxic species at higher pH values than warmer water (Driscoll and Schecher, 1990).
The bioavailability of Al is reduced by the presence of calcium (Ca; Brown, 1983), which can occupy the negatively charged gill sites. Dissolved organic carbon (DOC) also reduces the bioavailability of aluminium via the formation of organo-Al complexes (Alo; Erlandsson et al., 2010; Neville, 1985). High levels of DOC in rivers have been believed to be sufficient to protect fish gills from adverse Al effects (Lacroix and Kan, 1986; Vogt and Muniz, 1997; Witters et al., 1990).
Despite being the most common metal on Earth's crust, Al is usually immobilized in clays or hydroxide minerals in soils. Rates of Al release into soil water from soil minerals increase with three drivers: (1) low soil pH, (2) low soil base saturation, and (3) high soil DOC concentrations. Lowered pH increases the solubility of secondary minerals containing Al; Ali concentrations in stream water are generally negatively correlated with pH (Campbell et al., 1992; Kopáček et al., 2006; Seip et al., 1989). Low levels of base saturation can cause charge imbalances resulting in the release of Al into soil water and later into drainage water (Fernandez et al., 2003); thus, chronic acidification shifts available exchangeable cations in the soil water from Ca and magnesium (Mg) towards Al (Schlesinger and Bernhardt, 2013; Walker et al., 1990). Higher concentrations of DOC in soil water increase the release of Al via two mechanisms: (1) as an organic acid, DOC decreases soil pH, thereby increasing Al release (Lawrence et al., 2013); and (2) by forming organic complexes with Ali, DOC maintains a negative Al concentration gradient from the cation exchange sites to the soil water, increasing the rates of Al release (Edzwald and Van Benschoten, 1990; Jansen et al., 2003). Field studies confirm Al concentrations to be positively correlated with DOC (Campbell et al., 1992; Kopáček et al., 2006).
The general consensus in the literature is that elevated Ali concentrations occur during episodic storm events due to three possible mechanisms: (1) dilution of base cations during storm events, where flow paths move through shallower, more organic-rich soil layers; (2) added anions in snowmelt or rainfall (e.g., Cl−, , and F−) providing mobile anions that increase Ali export to streams; and (3) low pH associated with storm events that redissolves Al accumulated in the soil (Hooper and Shoemaker, 1985) and/or from the streambed (Norton et al., 1990). For example, from 1983 to 1984, Al concentrations for the River Severn in Wales increased tenfold during the storm flow peak compared with the base flow (Neal et al., 1986). The general consensus in the literature is also that Ali is seasonally elevated during spring snowmelt and autumn rainfall events, and it is seasonally depressed during summer months due to higher levels of DOC, such as in Quebec (Campbell et al., 1992), Russia (Rodushkin et al., 1995), and along the Czech–German border (Kopáček et al., 2000; Kopáček et al., 2006). Timing of the Ali peaks is important. If peak Ali concentrations coincide with vulnerable life stages of Atlantic salmon, such as during the spring when salmon transition from parr to smolt (i.e., smoltification) in preparation for life in the ocean (Kroglund et al., 2007; Monette and McCormick, 2008; Nilsen et al., 2013) or during the emergence of salmon fry from eggs (e.g., Farmer, 2000), the potential for large biological impacts from elevated Ali is particularly high.
Despite much progress in acidification research, the processes affecting Ali dynamics are not well understood (e.g., Mulder et al., 1990). Our understanding of Ali is limited by the relative paucity of samples; Ali is not measured as part of standard analyses. Thus, comprehension of Ali is also limited by the difficulty involved in comparing the wide variety of methods for estimating Ali; different definitions, often operational, of toxic Al include inorganic Al, inorganic monomeric Al, labile Al, Al3+, and cationic Al (Supplement Table S1.1).
Acid-sensitive areas of Nova Scotia, Canada, here abbreviated as NSA (see Clair et al., 2007), with once-famous wild Atlantic salmon populations, were heavily impacted by sulfur deposition at the end of the last century, which originated from coal burning in Central Canada and the Northeastern USA (Hindar, 2001; Summers and Whelpdale, 1976). NSA catchments are particularly sensitive to acid deposition due to base-cation-poor and slowly weathering bedrock that generates thin soils with a low acid neutralizing capacity (ANC), extensive wetlands, and episodic sea salt inputs (Clair et al., 2011; Freedman and Clair, 1987; Watt et al., 2000; Whitfield et al., 2006). Al was not considered to be a threat to Atlantic salmon in Nova Scotia because of the high natural levels of DOC in NSA rivers following preliminary research by Lacroix and Townsend (1987) and Lacroix (1989). A 2006 fall survey, however, (Dennis and Clair, 2012) found that Ali concentrations in NS exceeded the 15 µg L−1 toxic threshold for aquatic health, as determined from an extensive review of toxicological and geochemical literature by the European Inland Fisheries Advisory Council (EIFAC), in 7 of 42 rivers surveyed (Howells et al., 1990). No assessment of Ali has been carried out in NSA since that time, and little is known about the current extent and patterns of Ali in the region. Here, we conduct a 4-year survey of Ali concentrations in 10 streams in NSA in order to test the hypothesis that elevated DOC concentrations are sufficient to protect life from Ali and to identify the hydrologic conditions associated with elevated Ali concentrations.
2.1 Study area
We surveyed Ali concentrations at 10 study catchments in NSA, ranging from headwater to higher-order systems: Mersey River (MR), Moose Pit Brook (MPB), Pine Marten Brook (PMB), Maria Brook (MB), Brandon Lake Brook (BLB), above the West River lime doser (ALD), Upper Killag River (UKR), Little River (LR), Keef Brook (KB), and Colwell Creek (CC) (Table 1, Fig. 1). Our study catchments are predominantly forested with a mix of coniferous and deciduous species, and they drain slow-weathering, base-cation-poor bedrock, producing soils with a low ANC (Langan and Wilson, 1992; Tipping, 1989). The catchments also have relatively high DOC concentrations (Ginn et al., 2007) associated with the abundant wetlands in the region (Clair et al., 2008; Gorham et al., 1986; Kerekes et al., 1986).
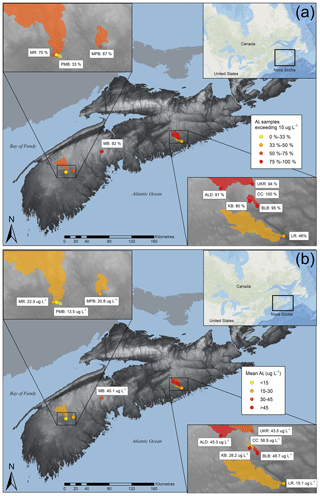
Figure 1Study site locations showing (a) mean Ali concentrations and (b) the proportion of samples in which Ali concentrations exceeded the 15 µg L−1 toxic threshold between spring 2015 and fall 2018. The shaded region corresponds to the catchments of monitoring sites. For additional site details, refer to Table 1.
Table 1Study site characteristics. “n” refers to the number of sampling events. Numbers in parentheses after the mean concentration represent the standard deviation. One Ali outlier was removed for MR (a value of 2 µg L−1 from 30 April 2015). pH is calibrated using the method outlined in Sect. S4.4.
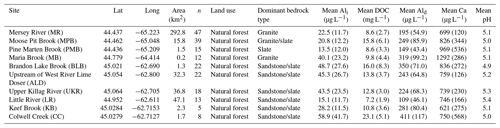
2.2 Data collection and analysis
We measured Ali concentrations at 3 of the 10 catchments from April 2015 to September 2017 (MR, MPB, and PMB) at a weekly to monthly frequency during the snow-free season (approximately April to November, Table S1.2). In the period from 2016 to 2018, seven sites were added and were sampled biweekly to monthly during the snow-free season.
Ali sampling events comprise grab samples for laboratory analysis and in situ measurements of pH and water temperature (Tw). We calculate Ali as the difference between dissolved Al (Ald) and Alo following Dennis and Clair (2012) and Poléo (1995), as in Eq. 1, separating the species in the field to reduce errors caused by Al species change due to variations in temperature and pH during transport from the field to laboratory.
Ald is measured as the Al concentration of a filtered (0.45 µm) sample and Alo is measured as the eluate from passing filtered water through a 3 cm negatively charged cation exchange column (Bond Elut Jr. strong cation exchange column). Samples were passed through the cation exchange column at a rate of approximately 30 to 60 drops per minute. Using this method, Alo is operationally defined as the non-labile, organically complexed metals and colloids; and Ali is defined as the positive ionic species of Al (e.g., Al3+, Al(OH)2+, and Al(OH)).
Thus, the cation exchange method determines the concentrations of weak monomeric organic Al complexes (passed through the column), monomeric inorganic Al (retained in the column), and colloidal, polymeric, and strong organic complexes that are measured after acid digestion of the sample (Gensemer and Playle, 1999). An assumption here is that the Al species retained on the exchange column would also be retained on the negatively charged fish gills and, therefore, have a potentially toxic effect (see Gensemer and Playle, 1999). The eluate is generally considered to be nontoxic; however, there is some evidence that precipitated polymeric Al and colloidal Al can be toxic to aquatic life (Parent and Campbell, 1994; Gensemer and Playle, 1999; Gensemer et al., 2018), although the nuances of this toxicity are unclear. To this end, the calculated Ali reported in this study represents a minimum concentration. Ultrafiltration (following Simpson et al., 2014) may improve the accuracy of the estimations of recently precipitated colloidal Al concentrations.
Stream chemistry samples (50 mL) were collected using sterilized polyethylene syringes into sterilized polyethylene bottles. Samples for sulfate () analysis were not filtered. Trace metal samples were filtered (0.45 µm) and preserved with nitric acid (HNO3). Samples for DOC analysis were filtered (0.45 µm) and transported in amber glass bottles containing sulfuric acid preservative (H2SO4) to prevent denaturation. All samples were cooled to 7 ∘C during transport to the laboratories. Samples were delivered to the laboratories within 48 h of collection, where they were further cooled to ≤4 ∘C prior to analysis (Sect. S4).
We examined correlations between Ali and the following water chemistry parameters: Ald, Ca, DOC, pH, , Tw, fluoride (F−), nitrate (), and runoff (where data were available). Correlations were analysed within and across sites. For the purposes of this study, we use the toxic threshold of Ali of 15 µg L−1, as the majority of our pH observations were greater than or equal to 5.0 (Table S1.2, Sect. S4.3).
We developed a generalized linear mixed model (GLMM) to identify the main drivers of the Ali concentration at the study sites. We tested Ald, DOC, Ca, , F−, , season, and Tw as potential drivers. The GLMM analysis was implemented with R version 3.6.2. (R Core Team, 2019) using the lme4 package (Bates et al., 2015). Due to the nonnormality of the Ali concentration data, the glmer function was employed for model fitting which uses the method of maximum likelihood for parameter estimation via Laplace approximation (Raudenbush et al., 2000). The study sites in the analysis were included as the random effect with the fixed effects provided in Table S1.3; Ald was not included in the GLMM, as it results in an overfit model (singularity). The Wald t test statistic and the Akaike information criterion (AIC) were used as measures of goodness of fit (Akaike, 1974; Bolker et al., 2009). Numerous iterations of fixed effects and interactions were considered in the GLMM development (Table S1.3). Multiple fixed effects were initially considered; however, several of the effects were not significant, although the overall model fit provided a low AIC. GLMMs were applied to assess seasonal drivers of Ali concentrations; however, owing to the limited amount of seasonal data collected, an analysis of both the site and seasonal random effects could not be carried out due to model singularities.
3.1 Prevalence of Ali
Ali concentrations exceed toxic levels (15 µg L−1) at all sites during the study period (Table S1.2) despite relatively high DOC concentrations (mean values ranging from 7.2 to 23.1 mg L−1 among the catchments; Table 1). Mean Ali concentrations across all sites range from 13 to 60 µg L−1 (Table 1), with the highest mean concentrations also occurring in the eastern part of the study area (Fig. 1a) where one site had 100 % of samples in exceedance (Fig. 1b). Ali concentrations exceed 100 µg L−1 (approximately 7 times the threshold) at three sites (Table S1.2). Our Ali concentrations are consistent with the 6.9–230 µg L−1 range of Ali concentrations measured across NSA by Dennis and Clair (2012) and are higher than concentrations measured in Norway from 1987 to 2010 (5–30 µg L−1; Hesthagen et al., 2016).
The percentage of Al not complexed by DOC (% Ali∕Ald) ranges from a minimum of 0.6 % to a maximum of 50 %, with a median value of 10.7 %, across all sites. These findings are similar to those reported in NSA by Dennis and Clair (2012) with respect to the proportion of Ali in total aluminium (Alt; min. = 4 %, max. =70.1 %, median =12.4 %), and less than those found by Lacroix (1989; over 90 % Alo∕Ald). These speciation results are also quite similar to other diverse environments, such as acid sulfate soil environments in Australia (Simpson et al., 2014). However, even when the percentage of Ali∕Ald is low, Ali concentrations remain well above the thresholds for toxicity (Supplement Figs. S2.2–S2.11). Similarly to our findings, previous studies show that Ali∕Ald is low during base flow (Bailey et al., 1995; Murdoch and Stoddard, 1992; Schofield et al., 1985; Figs. S2.2–S2.11 in this paper).
The highest concentrations of Ali (> 100 µg L−1) occurred in early summer (late June or early July in 2016–2018) when Ald, Ca, and DOC concentrations had not yet reached their annual peak (Table S1.2). The spring/summer extreme events occurred on the first exceptionally warm days (> 21 ∘C) of the year, in dry conditions, and when the proportion of Alo∕Ald was low (decreasing to approximately 60 %–70 % from higher levels of around 80 %–90 %; Figs. S2.2–S2.11). pH was not abnormally low during these events (ranging from 4.8 to 6.13), Ca concentrations were low (less than or equal to 800 µg L−1), and DOC concentrations ranged from 15 to 21 mg L−1.
At the sites with the longest and most frequent data collection (MR and MPB), Ali concentrations exceed the toxic threshold in consecutive samples for months at a time, particularly in the late summer (Fig. S2.1).
3.2 Potential Ali drivers
GLMM results reveal, via multiple fixed effect model combinations, that DOC and Tw are the most significant predictors of Ali concentrations (Table S1.3). When both DOC and Tw were included in the GLMM model, the resulting AIC was markedly lower, indicating that the inclusion of both parameters provided better predictive potential than DOC or Tw alone. Furthermore, the interaction between DOC and Tw was not significant in the model, provided a nominally lower AIC, and the correlation between the effects was low (−0.378), indicating that distinct processes are responsible for Ali concentrations. The strength of the Tw relationship is likely due to the role that increased temperature plays in activating biological drivers that mobilize Al (Hendershot et al., 1986).
Thus, the GLMM results show that DOC is positively correlated with Ali in the study area, in contrast to the standard conceptualization that DOC is inversely correlated with Ali; this suggests that the increased recruitment of Al in soils by DOC may outweigh the protective effects of DOC in freshwater, which is consistent with observations from other studies (e.g., Campbell et al., 1992; Kopáček et al., 2006).
Linear regressions show that Ald is significantly (α=0.05) and positively correlated with Ali at 7 of the 10 study sites (ALD, KB, LR, MB, MPB, MR, and PMB; Fig. 2, Table S1.5). Ca is significantly and positively correlated with Ali at two sites (MPB and MR; Fig. 2, Table S1.5). The positive relationship between Ca and Ali is the opposite of what was expected (following Rotteveel and Sterling, 2019). We hypothesize that this is due to the two study sites having very low Ca concentrations (mean concentrations below 1 mg L−1), below which soil water Ca concentrations are too low to retard Al release.
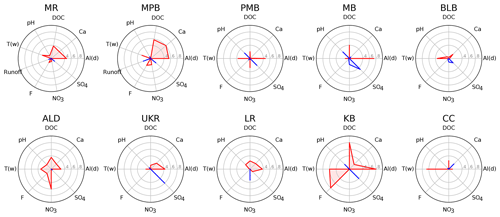
Figure 2Correlation among water chemistry parameters and Ali concentration, where red polygons and lines indicate a positive correlation with Ali, and blue polygons and lines indicate a negative correlation with Ali. One Ali outlier was removed for MR (a value of 2 µg L−1 from 30 April 2015). Correlation data are listed in Table S1.5.
Discharge is significantly and negatively correlated with Ali at one site MPB (Fig. 2, Table S1.5), which is in contrast with previous observations that Ali concentrations are positively correlated with discharge (Hooper and Shoemaker, 1985; Neal et al., 1986; Seip et al., 1989; Sullivan et al., 1986). Runoff data are available for only two of the study sites (MR and MPB); more runoff data are needed to improve our understanding of the relation between runoff and Ali in NSA.
We did not observe the negative association between pH and Ali that has been observed in previous studies (Campbell et al., 1992; Kopáček et al., 2006) – pH is negatively correlated with Ali at 4 of the 10 sites, but none of these relationships are statistically significant (Fig. 2, Table S1.5). The lack of a significant correlation may be due in part to other mechanisms that could potentially cloud the strength of the inverse relationship between pH and Al, such as increased DOC solubility at higher pH levels that leads to increased Al solubility in soils (Lydersen, 1990), pH buffering by Al in the lower pH range (Tomlinson, 1990), and the limited pH range in the dataset. We did observe a statistically significant positive relationship between pH and Ali∕Ald (Table S1.4); thus, it seems that pH may play a more important role in determining the proportion of different Al species rather than the absolute value of Ali present in stream water in chronically acidified conditions such as those found Nova Scotia. F− has also been found to be a complexing agent that affects the speciation of Al at low pH levels and relatively high concentrations of F− (> 1 mg L−1; Berger et al., 2015). The concentrations of F− at the study sites are mostly below this threshold (mean across all sites of 0.045 mg L−1); however, there is still a significant positive effect of F− on Ali concentrations at two sites (KB and MPB; Fig. 2, Table S1.5). and are also potential complexing ligands of Al; however, we did not observe any correlation between Ali and either of these parameters, except for a significant negative correlation between and Ali at MB.
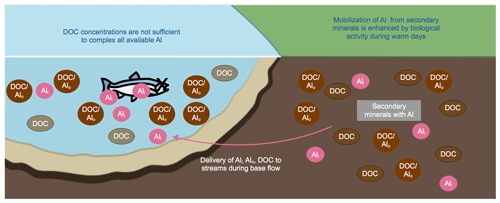
Figure 3Conceptual model of a new mechanism that can produce high concentrations of Ali in freshwater systems. Warm days increase biological activity that helps to mobilize Al from secondary minerals and enhances production of DOC; this, in turn, reduces pH and decreases Al saturation in soil solution by forming Al-organic complexes, thereby maintaining the Al concentration gradient away from secondary minerals. In rivers, the amount of DOC is insufficient to protect the fish from the amount of Ali mobilized in soils.
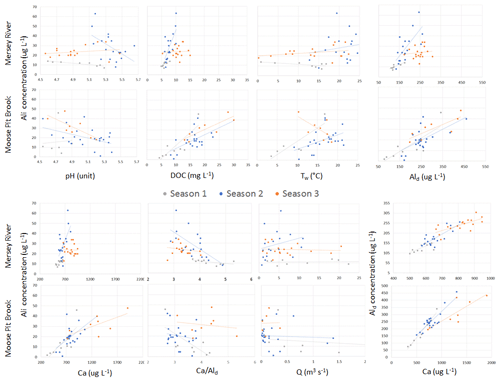
Figure 4Scatterplot relationships among water chemistry parameters for seasons 1, 2, and 3 at MR and MPB. R2 values are listed in Table S1.7. One runoff outlier was removed for MR (a value of 17 m3 s−1 from 22 April 2015). One runoff outlier was removed for MPB (a value of 35 m3 s−1 from 22 April 2015).
3.2.1 Possible seasonal groupings of Ali in NSA
At the two sites with the most samples, MPB and MR, groupings of data are visible that are temporally contiguous, suggesting seasonally dependent Ali behaviour (Fig. 4). This is supported by stronger linear correlations (r2) among variables when grouped by “season” (Table 2); for example, for the correlation between pH and Ali at MR, the r2 value improves from 0.02 for year-round data (Fig. S2.12) to up to 0.78 in season 1 (Fig. 4). The transition dates between the seasons are similar for the two catchments, but they are not the same (Table S1.2) and vary by year. Here, we propose an initial characterization of potential “seasons”; more research is needed to test these hypotheses on seasonal divisions and their drivers using larger datasets to test for statistical significance among the potential seasonal groupings.
Table 2Ali relations with other stream chemistry parameters separated by possible seasons. Dark shading represents an r2 value greater than 0.6. Medium shading represents an r2 value between 0.2 and 0.6. Light shading represents an r2 value between 0.0 and 0.2. Green indicates a negative relation. Orange indicates a positive relation.
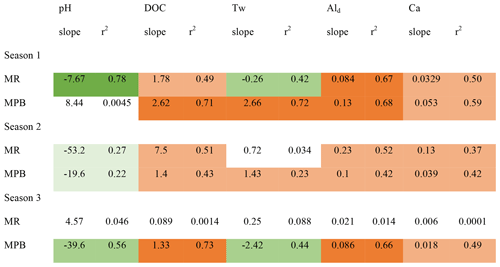
Season 1 (approximately April/May) is coincident with snowmelt runoff and is characterized by relatively low concentrations of Ali (2–46 µg L−1), a low pH (4.5–5.3), and lower concentrations of most constituents, including DOC, as well as cold temperatures (< 4 ∘C). During this season, Ali is strongly coupled with pH, DOC, Ald, and Ca in MR, but less so in MPB. A possible explanation for this is that season 1 is dominated by snowmelt hydrology in which cation exchange between soil and discharge occurs less efficiently, which has been attributed to ice and frozen soil potentially limiting water contact time with soil (Christophersen et al., 1990). It is important to note that we likely did not capture the first flush effect of increased Ali as has been noted in other studies (e.g., Hendershot et al., 1996). The onset of season 2 (approximately late June) is characterized by increasing Ali concentrations, temperature, and DOC. Ali and pH values are higher in this season, and Ali becomes strongly negatively correlated with pH as pH increases to the lower threshold for gibbsite. In MR in season 2, Ali has a strong positive relationship with DOC. The highest observed Ali concentrations of the year occur in season 2 (Fig. 3). Ali relations are weak in MR in season 3 (approximately September through March), which is likely due to the lower frequency of measurements during the winter. Season 3 in MR has the highest concentrations of dissolved constituents (Ald, Ca, and DOC), whereas only Ca has the highest concentrations in MPB.
With the inclusion of season as the random effect in the GLMM analysis (Table S1.6), limited data remains to undertake a robust comparative analysis, but it is included nonetheless to highlight the seasonal impacts that can be garnered from the limited dataset. pH and Tw were omitted due to singularities. These results reinforce that DOC concentrations are associated with Ali concentrations on a seasonal basis; however, more data are required to ascertain the effects of Tw on seasonal Ali concentrations.
In contrast with the conceptualization that peak Ali concentrations occur during storm flow (e.g., Campbell et al., 1992; Kopáček et al., 2000; Neal et al., 1986; Rodushkin et al., 1995), our data show elevated Ali concentrations during base flow conditions. These results suggest a new pathway for the generation of elevated Ali concentrations that is associated with base flow, warmer summer temperatures, and high DOC concentrations and is, thus, likely more chronic in nature. Nilsson (1985) suggested that this flow path has important consequences for Al concentrations in Swedish catchments. We hypothesize that this pathway is caused by increased temperatures resulting in higher levels of biological activity that mobilize Al in soils (Fig. 3; following Nilsson and Bergkvist, 1983). Biological activity further generates DOC that mobilizes Al to drainage water during summer base flow (Fig. 3). Other cases of increased Ali concentrations occurring during low flow and warming temperatures can be found in the literature in locations such as Ontario and Quebec (Hendershot et al., 1986, 1996) and in Virginia, USA (Cozzarelli et al., 1987).
3.3 Ecological implications
While the summer peak in Ali that we observed in NSA does not coincide with the smoltification period, continued exposure throughout the year may still negatively affect salmon populations, as the accumulation of Ali on gills reduces marine and freshwater salmon survival (Kroglund et al., 2007; Kroglund and Staurnes, 1999; Staurnes et al., 1996; Gibson et al., 2011).
In addition, elevated Ali concentrations appearing during low flow in the summer months suggest a more chronic delivery of elevated Ali to rivers, for which increases in the length and severity of droughts and heat waves due to climate change may further exacerbate effects on aquatic life. Because many peak Ali concentrations occur on the first exceptionally warm day in late spring, springtime warming associated with climate change may cause Ali peaks to occur earlier, thereby increasing the chance of the peak Ali concentrations overlapping with the smoltification season and emergence of salmon fry, which are the most vulnerable life stages of Atlantic salmon (e.g., Farmer, 2000), although the phenology of the smolt run is expected to similarly advance earlier in the year.
Our 4-year study of stream chemistry in NSA has two important findings. First, high DOC concentrations in rivers may not protect aquatic life against Ali as previously thought; our GLMM analysis suggests rather the opposite: higher DOC concentrations drive higher levels of Ali, even possibly on a seasonal basis. Thus, our study reveals that despite high DOC levels, widespread and persistent toxic concentrations of Ali in NSA freshwater systems pose a risk to aquatic, and potentially terrestrial, life. Second, our study highlights an overlooked hydrological pathway that is associated with high Ali concentrations – base flow – suggesting a chronically acidified/aluminium dynamic, in addition to episodic Ali peaks associated with storm flow. This base flow pathway demonstrates that Ali concentrations are chronically elevated during warmer summer months and that this Al pathway may, thus, be exacerbated by atmospheric warming. Our results suggest that the recent 88 % to 99 % population decline of the Southern Uplands Atlantic salmon population in NSA (Gibson et al., 2011) may be partially attributable to Ali, which is in contrast to earlier studies that downplayed the role of Ali in Atlantic salmon mortality (Lacroix and Townsend, 1987). These high Ali concentrations in NSA highlight the need to increase our understanding of the influence of Ali on both terrestrial and aquatic ecosystems as well as its implications for biodiversity. The catchments with the highest Ali levels had particularly low Ca levels; this is cause for concern, as Ca protects against Ali toxicity, and highlights the coincident threats of Ca depletion and elevated Al. Recent work has identified globally widespread low levels and declines in Ca (Weyhenmeyer et al., 2019), raising the question of what other regions may also have Ali levels exceeding toxic thresholds. The serious potential consequences of high Ali highlight the importance of actions to further reduce acid emissions and deposition, as critical loads are still exceeded across the province (Keys, 2015), and to adapt forest management practices to avoid base cation removal and depletion. The addition of base cations through liming and enhanced weathering of soils and freshwater may accelerate recovery from acidification.
All of the data presented and discussed in this paper are provided in the Supplement.
The supplement related to this article is available online at: https://doi.org/10.5194/hess-24-4763-2020-supplement.
SMS conceived the idea and led the writing of the paper. SM led the field data collection. SM and TAC designed the protocol for Ali sampling, assisted with data analysis, and helped with writing the article. LR performed spatial and statistical analysis, produced figures, and assisted with sample collection and draft writing. KH assisted with data analysis, figure production and editing, and contributed to drafting the paper. NLO led the GLMM analyses and contributed to the paper. TAC provided information on analytical and field sampling methods and helped with the selection of sampling sites. EAH contributed field samples, assisted with data analysis, and contributed to the article.
The authors declare that they have no conflict of interest.
The two anonymous referees contributed suggestions that improved the paper. The Atlantic Salmon Conservation Foundation, the Atlantic Canada Opportunities Agency, the Nova Scotia Salmon Association, and Fisheries and Oceans Canada provided financial support for the field data collection and the laboratory analyses. Marley Geddes, Siobhan Takla, Franz Heubach, Lorena Heubach, Emily Bibeau, and Ryan Currie provided field assistance.
Financial support was received from the National Science and Engineering Council of Canada (grant no. RGPIN06958-19), the Nova Scotia Salmon Association, Fisheries and Oceans Canada, the Atlantic Canada Opportunities Agency, and the Atlantic Salmon Conservation Foundation (research grant).
This paper was edited by Matthew Hipsey and reviewed by two anonymous referees.
Akaike, H.: A new look at statistical-model identification, IEEE Trans. Autom. Control, 19, 716–723, 1974.
Bailey, S. W., Driscoll, C. T., and Hornbeck, J. W.: Acid-base chemistry and aluminum transport in an acidic watershed and pond in New Hampshire, Biogeochemistry, 28, 69–91, 1995.
Baldigo, B. P. and Lawrence, G. B.: Composition of fish communities in relation to stream acidification and habitat in the Neversink River, New York, T. Am. Fish. Soc., 129, 60–76, 2000.
Bates, D., Maechler, M., Bolker, B., and Walker, S.: Fitting linear mixed-effects models using lme4, J. Stat. Soft., 67, 1–48, https://doi.org/10.18637/jss.v067.i01, 2015.
Beneš, F., Horecký, J., Senoo, T., Kamasová, L., Lamačová, A., Tátosová, J., Hardekopf, D. W., and Stuchlík, E.: Evidence for responses in water chemistry and macroinvertebrates in a strongly acidified mountain stream, Biologia, 72, 1049–1058, 2017.
Berger, T., Mathurin, F. A., Gustafsson, J. P., Peltola, P., and Åström, M. E.: The impact of fluoride on Al abundance and speciation in boreal streams, Chem. Geol., 409, 118–124, 2015.
Bolker, B. M., Brooks, M. E., Clark, C. J., Geange, S. W., Poulsen, J. R., Stevens, M. H. H., and White, J.-S. S.: Generalized linear mixed models: a practical guide for ecology and evolution, Trends Ecol. Evol., 24, 127–135, 2009.
Boudot, J. P., Becquer, T., Merlet, D., and Rouiller, J.: Aluminium toxicity in declining forests: a general overview with a seasonal assessment in a silver fir forest in the Vosges mountains (France), in: Annales des Sciences Forestières, 1994.
Brown, D.: Effect of calcium and aluminum concentrations on the survival of brown trout (Salmo trutta) at low pH, B. Environ. Contam. Tox., 30, 582–587, 1983.
Buchanan, C., Smith, Z., and Nagel, A.: Long-Term Water Quality Trends in USEPA Region 3 (Mid-Atlantic), United States Environmental Protection Agency, Rockville, MD, 2017.
Burns, D. A., McHale, M. R., Driscoll, C. T., and Roy, K. M.: Response of surface water chemistry to reduced levels of acid precipitation: comparison of trends in two regions of New York, USA, Hydrol. Process., 20, 1611–1627, 2006.
Campbell, P. G., Hansen, H. J., Dubreuil, B., and Nelson, W. O.: Geochemistry of Quebec north shore salmon rivers during snowmelt: organic acid pulse and aluminum mobilization, Can. J. Fish. Aquat. Sci., 49, 1938–1952, 1992.
Chew, H., Johnston, L. M., Craig, D., and Inch, K.: Aluminum contamination of groundwater: spring melt in Chalk River and Turkey Lakes Watersheds–preliminary results, Can. J. Fish. Aquat. Sci., 45, s66–s71, 1988.
Christophersen, N., Vogt, R. D., Neal, C., Anderson, H. A., Ferrier, R. C., Miller, J. D., and Seip, H. M.: Controlling mechanisms for stream water chemistry at the Pristine Ingabekken Site in mid-Norway: Some implications for acidification models, Water Resour. Res., 26, 59–67, 1990.
Clair, T. A., Dennis, I. F., Scruton, D. A., and Gilliss, M.: Freshwater acidification research in Atlantic Canada: a review of results and predictions for the future, Environ. Rev., 15, 153–167, 2007.
Clair, T. A., Dennis, I. F., Vet, R., and Laudon, H.: Long-term trends in catchment organic carbon and nitrogen exports from three acidified catchments in Nova Scotia, Canada, Biogeochemistry, 87, 83–97, 2008.
Clair, T. A., Dennis, I. F., and Vet, R.: Water chemistry and dissolved organic carbon trends in lakes from Canada's Atlantic Provinces: no recovery from acidification measured after 25 years of lake monitoring, Can. J. Fish. Aquat. Sci., 68, 663–674, 2011.
Cozzarelli, I. M., Herman, J. S., and Parnell Jr, R. A.: The mobilization of aluminum in a natural soil system: effects of hydrologic pathways, Water Resour. Res., 23, 859–874, 1987.
Davies, J., Jenkins, A., Monteith, D. T., Evans, C. D., and Cooper, D. M.: Trends in surface water chemistry of acidified UK freshwaters, 1988–2002, Environ. Pollut., 137, 27–39, 2005.
Dennis, I. F. and Clair, T. A.: The distribution of dissolved aluminum in Atlantic salmon (Salmo salar) rivers of Atlantic Canada and its potential effect on aquatic populations, Can. J. Fish. Aquat. Sci., 69, 1174–1183, 2012.
Driscoll, C. T. and Schecher, W. D.: The chemistry of aluminum in the environment, Environ. Geochem. Health, 12, 28–49, 1990.
Driscoll, C. T., Baker, J. P., Bisogni, J. J., and Schofield, C. L.: Effect of aluminium speciation on fish in dilute acidified waters, Nature, 284, 161–164, 1980.
Edzwald, J. K. and Van Benschoten, J. E.: Aluminum coagulation of natural organic matter, in: Chemical water and wastewater treatment, Springer, 341–359, 1990.
Erlandsson, M., Cory, N., Köhler, S., and Bishop, K.: Direct and indirect effects of increasing dissolved organic carbon levels on pH in lakes recovering from acidification, J. Geophys. Res.-Biogeo., 115, G03004, https://doi.org/10.1029/2009JG001082, 2010.
Evans, C. D., Cullen, J. M., Alewell, C., Kopácek, J., Marchetto, A., Moldan, F., Prechtel, A., Rogora, M., Veselý, J., and Wright, R.: Recovery from acidification in European surface waters, Hydrol. Earth Syst. Sci., 5, 283–298, https://doi.org/10.5194/hess-5-283-2001, 2001.
Exley, C., Chappell, J. S., and Birchall, J. D.: A mechanism for acute aluminium toxicity in fish, J. Theor. Biol., 151, 417–428, 1991.
Farmer, G. J.: Effects of low environmental pH on Atlantic salmon (Salmo salar L.) in Nova Scotia, Department of Fisheries and Oceans Canada, Dartmouth, N.S., Canada, 2000.
Fernandez, I. J., Rustad, L. E., Norton, S. A., Kahl, J. S., and Cosby, B. J.: Experimental acidification causes soil base-cation depletion at the Bear Brook Watershed in Maine, Soil Sci. Soc. Am. J., 67, 1909–1919, 2003.
Freedman, B. and Clair, T. A.: Ion mass balances and seasonal fluxes from four acidic brownwater streams in Nova Scotia, Can. J. Fish. Aquat. Sci., 44, 538–548, 1987.
Gensemer, R. W. and Playle, R. C.: The bioavailability and toxicity of aluminum in aquatic environments, Critical Reviews in Environmental Science and Technology, 29, 315–450, https://doi.org/10.1080/10643389991259245, 1999.
Gensemer, R. W., Gondek, J. C., Rodriquez, P. H., Arbildua, J. J., Stubblefield, W. A., Cardwell, A. S., Santore, R. C., Ryan, A. C., Adams, W. J., and Nordheim, E.: Evaluating the effects of pH, hardness, and dissolved organic carbon on the toxicity of aluminum to freshwater aquatic organisms under circumneutral conditions, Environ. Toxicol. Chem., 37, 49–60, 2018.
Gibson, A. J. F., Bowlby, H. D., Hardie, D. C., and O'Reilly, P. T.: Populations on the brink: low abundance of Southern Upland Atlantic salmon in Nova Scotia, Canada, N. Am. J. Fish. Manage., 31, 733–741, 2011.
Ginn, B. K., Cumming, B. F., and Smol, J. P.: Assessing pH changes since pre-industrial times in 51 low-alkalinity lakes in Nova Scotia, Canada, Can. J. Fish. Aquat. Sci., 64, 1043–1054, 2007.
Gorham, E., Underwood, J. K., Martini, F. B., and Ogden III, J. G.: Natural and anthropogenic causes of lake acidification in Nova Scotia, Nature, 324, 451–453, 1986.
Helliweli, S., Batley, G. E., Florence, T. M., and Lumsden, B. C.: Speciation and toxicity of aluminium in a model fresh water, Environ. Technol., 4, 141–144, 1983.
Hendershot, W. H., Dufresne, A., Lalande, H., and Courchesne, F.: Temporal variation in aluminum speciation and concentration during snowmelt, in: Acidic Precipitation, Springer, 1285–1291, 1986.
Hendershot, W. H., Courchense, F., and Jeffries, D. S.: Aluminum geochemistry at the catchment scale in watersheds influenced by acidic precipitation. In Sposito, G., The Environmental Chemistry of Aluminum, Lewis Publishers, NY, 1996.
Henriksen, A., Skogheim, O. K., and Rosseland, B. O.: Episodic changes in pH and aluminium-speciation kill fish in a Norwegian salmon river, Vatten, 40, 255–260, 1984.
Hesthagen, T. and Hansen, L. P.: Estimates of the annual loss of Atlantic salmon, Salmo salar L., in Norway due to acidification, Aquacult. Res., 22, 85–92, 1991.
Hesthagen, T., Fiske, P., and Saksgård, R.: Recovery of young brown trout (Salmo trutta) in acidified streams: What are the critical values for acid-neutralizing capacity?, Atmos. Environ., 146, 236–244, 2016.
Hindar, A.: Recommended liming strategies for salmon rivers in Nova Scotia, Canada, NIVA, 2001.
Hooper, R. P. and Shoemaker, C. A.: Aluminum mobilization in an acidic headwater stream: temporal variation and mineral dissolution disequilibria, Science, 229, 463–465, 1985.
Houle, D., Ouimet, R., Couture, S., and Gagnon, C.: Base cation reservoirs in soil control the buffering capacity of lakes in forested catchments, Can. J. Fish. Aquat. Sci., 63, 471–474, 2006.
Howells, G., Dalziel, T., Reader, J. P., and Solbe, J. F.: EIFAC water quality criteria for European freshwater fish: report on aluminium, Chem. Ecol., 4, 117–173, 1990.
Jansen, B., Nierop, K. G., and Verstraten, J. M.: Mobility of Fe (II), Fe (III) and Al in acidic forest soils mediated by dissolved organic matter: influence of solution pH and metal/organic carbon ratios, Geoderma, 113, 323–340, 2003.
Kerekes, J., Beauchamp, S., Tordon, R., Tremblay, C., and Pollock, T.: Organic versus anthropogenic acidity in tributaries of the Kejimkujik watersheds in western Nova Scotia, Water Air Soil Pollut., 31, 165–1793, 1986.
Keys, K.: Acid Deposition and Base Cation Depletion in Northeastern Forest Soils: a Review with Focus on Nova Scotia Conditions, Dalhousie University, Halifax, N.S., Canada, 2015.
Kopáček, J., Hejzlar, J., and Porcal, P.: Seasonal patterns in chemistry of tributaries to Plesne and Čertovo lakes in the 1998 hydrological year, Silva Gabreta, 4, 105–116, 2000.
Kopáček, J., Turek, J., Hejzlar, J., Kaňa, J., and Porcal, P.: Element fluxes in watershed-lake ecosystems recovering from acidification: Čertovo Lake, the Bohemian Forest, 2001–2005, Biologia, 61, S41–S426, 2006.
Kroglund, F. and Staurnes, M.: Water quality requirements of smolting Atlantic salmon (Salmo salar) in limed acid rivers, Can. J. Fish. Aquat. Sci., 56, 2078–2086, 1999.
Kroglund, F., Finstad, B., Stefansson, S. O., Nilsen, T. O., Kristensen, T., Rosseland, B. O., Teien, H. C., and Salbu, B.: Exposure to moderate acid water and aluminum reduces Atlantic salmon post-smolt survival, Aquaculture, 273, 360–373, 2007.
Lacoul, P., Freedman, B., and Clair, T.: Effects of acidification on aquatic biota in Atlantic Canada, Environ. Rev., 19, 429–460, 2011.
Lacroix, G. L.: Ecological and physiological responses of Atlantic salmon in acidic organic rivers of Nova Scotia, Canada, Water Air Soil Pollut., 46, 375–386, 1989.
Lacroix, G. L. and Kan, K. T.: Speciation of Aluminum in Acidic Rivers of Nova Scotia Supporting Atlantic Salmon: A Methodological Evaluation, Canadian Technical Report of Fisheries and Aquatic Sciences, Department of Fisheries and Oceans Canada, St. Andrews, NB, 1986.
Lacroix, G. L. and Townsend, D. R.: Responses of juvenile Atlantic salmon (Salmo salar) to episodic increases in acidity of Nova Scotia rivers, Can. J. Fish. Aquat. Sci., 44, 1475–1484, 1987.
Langan, S. J. and Wilson, M. J.: Predicting the regional occurrence of acid surface waters in Scotland using an approach based on geology, soils and land use, J. Hydrol., 138, 515–528, 1992.
Lawrence, G. B., Dukett, J. E., Houck, N., Snyder, P., and Capone, C.: Increases in dissolved organic carbon accelerate loss of toxic Al in Adirondack lakes recovering from acidification, Environ. Sci. Technol., 47, 7095–7100, 2013.
LaZerte, B. D.: Forms of aqueous aluminum in acidified catchments of central Ontario: a methodological analysis, Can. J. Fish. Aquat. Sci., 41, 766–776, 1984.
Lydersen, E.: The solubility and hydrolysis of aqueous aluminium hydroxides in dilute fresh waters at different temperatures, Hydrol. Res., 21, 195–204, 1990.
McCormick, S. D., Lerner, D. T., Monette, M. Y., Nieves-Puigdoller, K., Kelly, J. T., and Björnsson, B. T.: Taking it with you when you go: how perturbations to the freshwater environment, including temperature, dams, and contaminants, affect marine survival of salmon, in: American Fisheries Society Symposium, 2009.
Monette, M. Y. and McCormick, S. D.: Impacts of short-term acid and aluminum exposure on Atlantic salmon (Salmo salar) physiology: a direct comparison of parr and smolts, Aquat. Toxicol., 86, 216–226, 2008.
Monteith, D. T., Evans, C. D., Henrys, P. A., Simpson, G. L., and Malcolm, I. A.: Trends in the hydrochemistry of acid-sensitive surface waters in the UK 1988–2008, Ecol. Indic., 37, 287–303, 2014.
Mulder, J., Christophersen, N., Hauhs, M., Vogt, R. D., Andersen, S., and Andersen, D. O.: Water flow paths and hydrochemical controls in the Birkenes catchment as inferred from a rainstorm high in seasalts, Water Resour. Res., 26, 611–622, 1990.
Murdoch, P. S. and Stoddard, J. L.: The role of nitrate in the acidification of streams in the Catskill Mountains of New York, Water Resour. Res., 28, 2707–2720, 1992.
Neal, C., Smith, C. J., Walls, J., and Dunn, C. S.: Major, minor and trace element mobility in the acidic upland forested catchment of the upper River Severn, Mid Wales, J. Geol. Soc., 143, 635–648, 1986.
Neville, C. M.: Physiological response of juvenile rainbow trout, Salmo gairdneri, to acid and aluminum–prediction of field responses from laboratory data, Can. J. Fish. Aquat. Sci., 42, 2004–2019, 1985.
Nilsen, T. O., Ebbesson, L. O., Handeland, S. O., Kroglund, F., Finstad, B., Angotzi, A. R., and Stefansson, S. O.: Atlantic salmon (Salmo salar L.) smolts require more than two weeks to recover from acidic water and aluminium exposure, Aquat. Toxicol., 142, 33–44, 2013.
Nilsen, T. O., Ebbesson, L. O., Kverneland, O. G., Kroglund, F., Finstad, B., and Stefansson, S. O.: Effects of acidic water and aluminum exposure on gill Na, K-ATPase α-subunit isoforms, enzyme activity, physiology and return rates in Atlantic salmon (Salmo salar L.), Aquat. Toxicol., 97, 250–259, 2010.
Nilsson, S. I.: Budgets of aluminium species, iron and manganese in the Lake Gårdsjön catchment in SW Sweden, Ecol. Bull., 37, 120–132, 1985.
Nilsson, S. I. and Bergkvist, B. O.: Aluminium chemistry and acidification processes in a shallow podzol on the Swedish west coast, Water Air Soil Pollut., 20, 311–329, 1983.
Norton, S. A., Kahl, J. S., Henriksen, A., and Wright, R. F.: Buffering of pH Depressions by Sediments in Streams and Lakes, in: Acidic Precipitation, Advances in Environmental Science, edited by: Norton, S. A., Lindberg, S. E., and Page, A. L., vol 4. Springer, New York, NY, https://doi.org/10.1007/978-1-4612-4456-1_5, 1990.
Parent, L. and Campbell, P. G. C.: Aluminum bioavailability to the green alga Chlorella pyrenoidosa in acidified synthetic soft water, Environ. Toxicol. Chem., 13, 587–598, 1994.
Parrish, D. L., Behnke, R. J., Gephard, S. R., McCormick, S. D., and Reeves, G. H.: Why aren't there more Atlantic salmon (Salmo salar)?, Can. J. Fish. Aquat. Sci., 55, 281–287, 1998.
Poléo, A. B.: Aluminium polymerization – a mechanism of acute toxicity of aqueous aluminium to fish, Aquat. Toxicol., 31, 347–356, 1995.
R Core Team.: R: A language and environment for statistical computing. R Foundation for Statistical Computing, Vienna Austria, available at: https://www.r-project.org/ (last access: 7 August 2020), 2019.
Raudenbush, S. W., Yang, M.-L., and Yosef, M.: Maximum likelihood for generalized linear models with nested random effects via high-order, multivariate Laplace approximation, J. Comput. Graph. Stat., 9, 141–157, 2000.
Regish, A. M., Kelly, J. T., O'Dea, M. F., and McCormick, S. D.: Sensitivity of Na /K -ATPase isoforms to acid and aluminum explains differential effects on Atlantic salmon osmoregulation in fresh water and seawater, Can. J. Fish. Aquat. Sci., 75, 1319–1328, 2018.
Rodushkin, I., Moiseenko, T., and Kudravsjeva, L.: Aluminium in the surface waters of the Kola Peninsula, Russia, Sci. Total Environ., 163, 55–59, 1995.
Rosseland, B. O., Eldhuset, T. D., and Staurnes, M.: Environmental effects of aluminium, Environ. Geochem. Health, 12, 17–27, 1990.
Rotteveel, L. and Sterling, S.: Five Aluminum Seasonality Regimes Identified in Chronically Acidified Rivers of Nova Scotia, Environ. Sci. Technol., 54, 807–817, 2019.
Schlesinger, W. H. and Bernhardt, E. S.: Biogeochemistry: an analysis of global change, Academic Press, Amsterdam, the Netherlands, 2013.
Schofield, C. L. and Trojnar, J. R.: Aluminum toxicity to brook trout (Salvelinusfontinalis) in acidified waters, in: Polluted rain, Springer, 341–366, 1980.
Schofield, C. L., Galloway, J. N., and Hendry, G. R.: Surface water chemistry in the ILWAS basins, Water Air Soil Pollut., 26, 403–423, 1985.
Seip, H. M., Andersen, D. O., Christophersen, N., Sullivan, T. J., and Vogt, R. D.: Variations in concentrations of aqueous aluminium and other chemical species during hydrological episodes at Birkenes, southernmost Norway, J. Hydrol., 108, 387–405, 1989.
Simpson, S. L., Vardanega, C. R., Jarolimek, C., Jolley, D. F., Angel, B. M., and Mosley, L. M.: Metal speciation and potential bioavailability changes during discharge and neutralisation of acidic drainage water, Chemosphere, 103, 172–180, 2014.
Skjelkvåle, B. L., Stoddard, J. L., Jeffries, D. S., Tørseth, K., Høgåsen, T., Bowman, J., Mannio, J., Monteith, D. T., Mosello, R., and Rogora, M.: Regional scale evidence for improvements in surface water chemistry 1990–2001, Environ. Pollut., 137, 165–176, 2005.
Staurnes, M., Hansen, L. P., Fugelli, K., and Haraldstad, Ø: Short-term exposure to acid water impairs osmoregulation, seawater tolerance, and subsequent marine survival of smolts of Altantic salmon (Salmo salar), Can. J. Fish. Aquat. Sci., 53, 1695–1704, 1996.
Stoddard, J. L., Jeffries, D. S., Lükewille, A., Clair, T. A., Dillon, P. J., Driscoll, C. T., Forsius, M., Johannessen, M., Kahl, J. S., and Kellogg, J. H.: Regional trends in aquatic recovery from acidification in North America and Europe, Nature, 401, 575, 1999.
Summers, P. W. and Whelpdale, D. M.: Acid precipitation in Canada, Water Air Soil Pollut., 6, 447–455, 1976.
Sullivan, T. J., Christophersen, N., Muniz, I. P., Seip, H. M., and Sullivan, P. D.: Aqueous aluminium chemistry response to episodic increases in discharge, Nature, 323, 324–327, 1986.
Tipping, E.: Acid-sensitive waters of the English Lake District: a steady-state model of streamwater chemistry in the upper Duddon catchment, Environ. Pollut., 60, 181–208, 1989.
Tomlinson, G. H., Tomlinson, F. L., Grennfelt, P., Httl, R., Httermann, A., Mehne, B. M., and von Tiedemann, S.: Effects of acid deposition on the forests of Europe and North America, CRC Press Inc., 281 pp., 1990.
Vogt, R. D. and Muniz, I. P.: Soil and stream water chemistry in a pristine and boggy site in mid-Norway, Hydrobiologia, 348, 19–38, 1997.
Walker, W. J., Cronan, C. S., and Bloom, P. R.: Aluminum solubility in organic soil horizons from northern and southern forested watersheds, Soil Sci. Soc. Am. J., 54, 369–374, 1990.
Warby, R. A., Johnson, C. E., and Driscoll, C. T.: Chemical recovery of surface waters across the northeastern United States from reduced inputs of acidic deposition: 1984–2001, Environ. Sci. Technol., 39, 6548–6554, 2005.
Warby, R. A., Johnson, C. E., and Driscoll, C. T.: Continuing acidification of organic soils across the northeastern USA: 1984–2001, Soil Sci. Soc. Am. J., 73, 274–284, 2009.
Watmough, S. A., Eimers, C., and Baker, S.: Impediments to recovery from acid deposition, Atmos. Environ., 146, 15–27, 2016.
Watt, W. D.: A summary of the impact of acid rain on Atlantic salmon (Salmo salar) in Canada, Water Air Soil Pollut., 35, 27–35, 1987.
Watt, W. D., Scott, C. D., Zamora, P. J., and White, W. J.: Acid toxicity levels in Nova Scotian rivers have not declined in synchrony with the decline in sulfate levels, Water Air Soil Pollut., 118, 203–229, 2000.
Wauer, G. and Teien, H.: Risk of acute toxicity for fish during aluminium application to hardwater lakes, Sci. Total Environ., 408, 4020–4025, 2010.
Weyhenmeyer, G. A., Hartmann, J., Hessen, D. O., Kopáček, J., Hejzlar, J., Jacquet, S., Hamilton, S. K., Verburg, P., Leach, T. H., Schmid, M., and Flaim, G.: Widespread diminishing anthropogenic effects on calcium in freshwaters, Sci. Rep.-UK, 18, 10450, https://doi.org/10.1038/s41598-019-46838-w, 2019.
Whitfield, C. J., Aherne, J., Watmough, S. A., Dillon, P. J., and Clair, T. A.: Recovery from acidification in Nova Scotia: temporal trends and critical loads for 20 headwater lakes, Can. J. Fish. Aquat. Sci., 63, 1504–1514, 2006.
Whitfield, C. J., Aherne, J., Dillon, P. J., and Watmough, S. A.: Modelling acidification, recovery and target loads for headwater catchments in Nova Scotia, Canada, Hydrol. Earth Syst. Sci., 11, 951–963, https://doi.org/10.5194/hess-11-951-2007, 2007.
Witters, H. E., Van Puymbroeck, S., Vangenechten, J., and Vanderborght, O.: The effect of humic substances on the toxicity of aluminium to adult rainbow trout, Oncorhynchus mykiss (Walbaum), J. Fish Biol., 37, 43–53, 1990.