the Creative Commons Attribution 4.0 License.
the Creative Commons Attribution 4.0 License.
Hydrological processes and permafrost regulate magnitude, source and chemical characteristics of dissolved organic carbon export in a peatland catchment of northeastern China
Yuedong Guo
Changchun Song
Wenwen Tan
Xianwei Wang
Yongzheng Lu
Permafrost thawing in peatlands has the potential to alter the catchment export of dissolved organic carbon (DOC), thus influencing the carbon balance and cycling in linked aquatic and ocean ecosystems. Peatlands along the southern margins of the Eurasian permafrost are relatively underexplored despite the considerable risks associated with permafrost degradation due to climate warming. This study examined dynamics of DOC export from a permafrost peatland catchment located in northeastern China during the 2012 to 2014 growing seasons. The estimated annual DOC loads varied greatly between 3211 and 19 022 kg yr−1, with a mean DOC yield of 4.7 g m−2 yr−1. Although the estimated DOC yield was in the lower range compared with other permafrost regions, it was still significant for the net carbon balance in the studied catchment. There were strong linkages between daily discharge and DOC concentrations in both wet and dry years, suggesting a transport-limited process of DOC delivery from the catchment. Discharge explained the majority of both seasonal and interannual variations of DOC concentrations, which made annual discharge a good indicator of total DOC load from the catchment. As indicated by three fluorescence indices, DOC source and chemical characteristics tracked the shift of flow paths during runoff processes closely. Interactions between the flow path and DOC chemical characteristics were greatly influenced by the seasonal thawing of the soil active layer. The deepening of the active layer due to climate warming likely increases the proportion of microbial-originated DOC in baseflow discharge.
- Article
(4957 KB) - Full-text XML
- BibTeX
- EndNote
Permafrost soils have acted as sinks for atmospheric carbon (C) since at least the late Pleistocene and serve as key sources of dissolved organic carbon (DOC) for linked aquatic and ocean ecosystems (Opsahl et al., 1999; Kicklighter et al., 2013). Because changes in the quantity and quality of exported DOC can greatly alter the energy cycles of the linked oceans, considerable progress has been made in recent years to better evaluate potential changes in DOC export patterns from permafrost regions (Townsend-Small et al., 2011; Vonk et al., 2013). However, uncertainties remain regarding the primary drivers and the fate of DOC due to complex interactions between hydrological and thermal dynamics as well as biochemical drivers (Olefeldt and Roulet, 2012; Kicklighter et al., 2013).
Significant losses of near-surface permafrost have been observed over the past century and such outcomes have induced considerable changes in hydrological processes and soil thermal regimes (Lyon et al., 2009; Lessels et al., 2015), in turn altering the magnitude and timing of terrestrial DOC export processes. The flow pathway is an important and well-documented regulator of DOC export from permafrost regions (Ågren et al., 2010; Guo et al., 2015). Owing to increased levels of hydrological access to previously frozen soils following permafrost degradation, DOC export was forecast to increase in Siberian rivers along a latitudinal transect (Frey and McClelland, 2009). However, permafrost degradation also increased the likelihood of interactions between subsurface flows and mineral soils, which should lead to considerable DOC absorption by fine soil particles and in turn a decrease in DOC export magnitude (Petrone et al., 2006; Striegl et al., 2005). There were significant disparities in DOC export concentrations and seasonal patterns between surface- and subsurface-dominated runoff processes in permafrost catchments (Laudon et al., 2011). The capacity for DOC export from permafrost soils was closely linked to lateral subsurface flow (Striegl et al., 2007; Lyon et al., 2010). Therefore, alterations to flow pathways during permafrost freeze–thaw cycles are some of the most important factors to consider in evaluating DOC export potential in a peatland.
Flow pathways also determine chemical composition of the DOC exported from permafrost catchments, which in turn can influence downstream DOC mineralization rates and carbon emissions from streams, lakes and oceans (Mann et al., 2012; Cory et al., 2014). DOC composition can be by flow pathways along the organic-mineral soil layer. Mineral soil particles preferentially absorbed dissolved organic matter high in aromatic components with large molecular weights or acidic functional groups and aromatic structures (Kalbitz et al., 2005). In contrast, hydrophilic fatty microbial products with low molecular weights were desorbed and released (Striegl et al., 2005). To date, a partial theoretical framework and methods have been developed to understand alterations in DOC chemical characteristics following permafrost degradation (Spencer et al., 2015). But uncertainties still exist in understanding the entire way hydrological processes affect the magnitude and chemical characteristics of DOC exported from permafrost peatland catchments.
Given the high spatial heterogeneity of peatlands and the complexity of hydrological processes in permafrost regions, it is important to understand the magnitude and controls on DOC export in different permafrost regions, especially in the south part of the Eurasian continent where limited research has been performed to date. Our study focused on dynamics of DOC release from the Fukuqi River, a tributary of the Amur River positioned along the northern slopes of the Great Xing'an Mountains in northeastern China. The Great Xing'an Mountains form an important barrier from Siberian cold air masses and monsoons of East Asia. The mean annual temperature of the area has increased on average by 0.3∘ every 10 years over the last 50 years, and the thickness of the active layer has increased by 20–40 cm on the southern slopes of the Great Xing'an Mountains from the 1970s to 2000 (Jin et al., 2000). However, few studies have focused on possible consequences of permafrost degradation in this region to date. This work thus investigated potential changes in DOC export patterns by answering the following questions.
-
What is the DOC load transported by discharge from the entire catchment?
-
What is the relationship between runoff processes and concentrations, sources and chemical characteristics of DOC?
2.1 Study area
Northern sections of the Great Xing'an Mountains in China are located along the southern margins of the continuous permafrost zone in Eurasia. The area represents the most remote region of the East Asia monsoon of the east Eurasian continent. The region includes approximately 8.245 × 103 km2 of natural wetlands, representing a major proportion of cold temperate wetlands and an important reservoir of soil carbon and usable water resources for northeastern China.
The Fukuqi River, a second-order branch of the Amur River, is located in the continuous permafrost of the northern slope of the Great Xing'an Mountains (Fig. 1). The catchment extends across an area of 287 km2, with an annual mean temperature of −4.2 ∘C and a mean annual precipitation of 425 mm (1959–2013). Peatland covers the flat river valley and ranges in altitude from 500 to 580 m. Mountains surround the peatland and have a much steeper slope than the peatland (Fig. 1). The peat layer, which is approximately 0.3–0.4 m thick, is composed of typical organic soil, with organic matter levels ranging from 40 to 60 % and with porosity levels ranging from 60 to 20 % near the surface. According to previous field surveys, the peatlands account for more than 90 % of the total carbon stock in the catchment but cover only about one-third of the total area. The maximum thaw depth of the active layer, ranging from 60 to 80 cm, usually occurs in early August. Below the peat soil layer is mineral soil with a much lower organic content (< 5 %) and soil porosity (< 10 %) than the upper peat soil. Sphagnum mosses (S.capillifolium, S. magellanicum) and sedges (Eriophorum vaginatum) are the dominant vegetation. The growing season is from May until late September. The upland mountains on both sides of the valley are extensively covered by mineral soil and gravels with little organic content due to the continuous logging and frequent fires during the past 60 years. The original coniferous forest has been replaced by planted young Pinus sylvestris var. mongolica. The maximum thaw depth of the forest ranges from 80 to 100 cm, which is slightly deeper than the peatland.
2.2 Sampling and monitoring program
Monitoring was conducted from early May to late September in 2012, 2013 and 2014. A gauging station to profile DOC concentrations and hydrological parameters was set for the lower reaches of the Fukuqi River (Fig. 1). Water samples were collected from the stream profile every 1–5 days with 200 mL polyethylene bottles. A higher sampling frequency was applied during flood events, whereas a lower sampling frequency was using during periods of low water. Soil porewater in the peatland was collected from three sites located 50–100 m away from the main river channel on 8 June, 30 June, 27 July and 25 August in 2013 (Fig. 1). When sampling, 100 mL samples of soil porewater were collected at 10 cm intervals along the active layer using ceramic soil porewater samplers (SIC20, Germany). Porewater was collected from the same three to five locations at each of the three sites for each sampling period. Due to the gradual thawing of the active layer during the growing seasons, the maximum sampling depths differed for each of the four sampling periods. The water samples were filtered through a 0.45 µm glass fiber membrane and stored in 4∘ in the dark for at most 7 days before analysis using a DOC analyzer (C-VCPH, Shimadzu, Japan) (Guo et al., 2014). The river began to freeze after September in each year, and flow under the ice was not detected during the winter.
Discharge (Q) from through the gauging profile was calculated by measuring water level and flow velocity automatically using a water level monitor (Odyssey, New Zealand, accuracy: ±2 mm) and a flow meter (Argonaut-ADV, USA, accuracy: ±0.01 m s−1). Air temperature and soil temperature at 0–1.0 m depth were also recorded by an automatic microclimate gauging tower (CS3000, Campbell, USA) set in the center part of the peatlands. Water level in the peatland was recorded continuously near the gauging tower with the same Odyssey monitor. The thaw depth of the peatland active layer was manually surveyed weekly with a 1.0 m stainless steel ruler (accuracy: 0.1 cm) at the same three sites. Information on the temperature (∘C), electrical conductivity (mS cm−1) and turbidity (NTU) in the sampling profile was logged continuously using a multi-parameter water quality sonde (6600EDS, YSI, USA). About one-fifth of the water quality data mainly in May and late September were lost because of equipment malfunction at low temperature. All the instruments were set to collect data every 6 h while they were being deployed.
2.3 Fluorescence measurements
Excitation-emission matrixes (EEMs) of the water samples were measured using a Hitachi F-7000 fluorescence spectrometer (Hitachi High Technologies, Japan) with a 50 W ozone-free Xenon arc lamp and R928P photomultiplier tube fitted as a detector. The spectrometer was set to collect signals using a 5 nm bandpass on excitation and emission monochromators at a canning speed of 3200 nm min−1. EEMs were recorded for excitation spectra of between 220 and 400 nm and for emission spectra of between 300 and 500 nm. To eliminate the inner-filter effect, samples were diluted with deionized water to a UV absorbance at λ= 254 nm of 0.2 absorbance units (cm−1). Milli-Q water blank EEMs were subtracted from the sample EEMs to eliminate Raman scatter peaks. Then, the EEMs were normalized to the area under the Raman scatter peak (excitation wavelength of 350 nm) of a Milli-Q water sample run the same day. The fluorescence intensities measured were reported in Raman Units (RU) in this study.
Three spectral indices were calculated from the EEMs to quantify chemical characteristics of the dissolved organic matter. The humification index (HIX) is defined as the ratio of the sum of λem= 435–480 nm to the sum of λem= 300–345 for excitation at 254 nm and quantifies the complexity and aromaticity of dissolved organic matter. High HIX values denote the presence of highly humified or more complex organic matter (Ohno, 2002). The fluorescence (FI) was the second index and is defined as the ratio of fluorescence emission intensities at 470 and 520 nm for excitation at 370 nm. The recommended FI for plant-derived organic matter is 1.3–1.4 and that for materials of microbial origin is 1.7–2.0 (McKnight et al., 2001). The third index we measured was the biological index (BIX), defined as the ratio of intensities at λem 380 and 430 nm for excitation at 310 nm. BIX ranges from 0.6 to 1.0 or greater generally, and it is a complementary index for evaluating the relative contributions of microbial-derived organic matter (Huguet et al., 2009). Lower values mean proportionately less microbial-derived organic matter.
2.4 Estimation of DOC load and yield
A web-based program LOADEST was used to estimate the DOC load for the three years (https://engineering.purdue.edu/mapserve/LOADEST/). LOADEST uses linear regression models to identify relationships between discharge and DOC concentrations and in turn to estimate the daily DOC load by applying the statistical method of adjusted maximum likelihood estimation, maximum likelihood estimation (MLE) and least absolute deviation. In total, eleven models are used in the program, and the best one was automatically selected to fit the data on the base of Akaike information criterion (Park et al., 2015). In our study, 36, 35 and 31 measurements were used to calculate DOC loads for the years 2012, 2013 and 2014 respectively. Loads were estimated using the MLE method according to the standard error (SE) and the distribution of the residuals. The DOC yield was calculated as the load divided by the entire catchment area.
2.5 Statistical analyses
The mean and the standard deviation of the DOC concentrations in the stream and soil porewater, and the three fluorescence indices were statistically analyzed with the Statistical Program for Social Sciences (SPSS) version 13.0 software. The relationship between the hydrological factors and the DOC concentration and the fluorescence indices was examined by a two-tailed Pearson correlation and regression analysis, where the p-values were calculated to test for significance. Analysis of covariance (ANCOVA) was also conducted to distinguish whether the relationships between discharge and the DOC characteristics (concentration and fluorescence indices) were statistically different for different years and whether there were other factors controlling the DOC characteristics besides discharge.
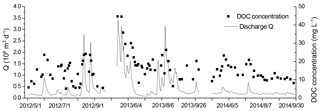
Figure 3Dissolved organic carbon (DOC) concentrations and discharge (Q) observed during the growing seasons of 2012 to 2014.
3.1 Environmental conditions
Substantial interannual and seasonal variations in precipitation were observed for the three years (Fig. 2). The total precipitation reached 202.5, 520.8 and 164 mm in 2012, 2013 and 2014, respectively. Based on our statistics on the regional climate dataset from 1970 to 2005, 2013 was an extremely wet year due to excessive rainfall occurring in the spring and summer. The total rainfall in 2012 was within a normal range, while that for 2014 indicated an extreme dry year. Probably owing to the abundant rainfall in 2013, the air temperature during the growing season in that year, with a mean value of 12.9∘, was lower than the mean temperatures of 2012 and 2014 (on average 13.7∘). However, mean values in all three years were within the average long-term range. We also found no significant differences in the maximum thaw depths of the soil active layer for the three years. The standing water levels in the peatland close to the stream channel declined gradually across the growing seasons, probably due to the deepening of active layer. Water levels in the peatland were always lower than the peat surface during the three years of sampling.
3.2 DOC concentrations and loads
DOC concentrations in the Fukuqi River fluctuated considerably with stream discharge during the three growing seasons (Fig. 3). The mean DOC concentration in 2013 was significantly larger than that in 2012 and 2014 (Table 1). Great seasonal variability was clearly observed for all of the three years, which mainly resulted from the varied rainfall patterns as shown in Fig. 2. In the three years of measurements, the maximum concentration of 44.7 mg L−1 was found in the early spring of 2013 and was accompanied by the peak flood occurring during the three years. The estimated DOC loads and yields for the three years varied greatly (Table 1). The total load, as well as the DOC yield in the wet year of 2013 was about 6 times that of the extreme dry year of 2014. The annual load and yield in 2012 differed greatly compared with 2014, but the estimated mean concentrations were quite similar. Monthly variability in loads was also found, with maximum values occurring either in May or August. The mean DOC load for the three years was 4.7 g m−2 yr−1. Several large floods contributed the majority of the load. Statistically, nine flood events (maximum discharge > 1.0 × 106 m3 d−1) were responsible for 81 % of the load, while five floods with a discharge level > 2.0 × 106 m3 d−1 accounted for 65 % of the total load.
Table 1Mean annual DOC loads, concentrations and yields estimated by the LOADEST program for 2012–2014.
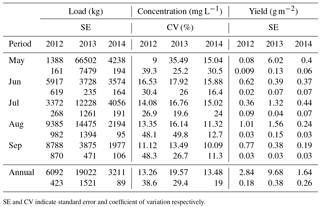
SE and CV indicate standard error and coefficient of variation respectively.
Significant positive correlations were found between DOC concentrations and discharge for all three growing seasons (Fig. 4). However, results of covariance analysis suggested that the adjusted mean DOC concentrations after eliminating the influence of discharge were statistically different for the three years. This result suggested an interannual variability in the linear relationship between DOC concentration and discharge (Table 2). As indicated by Adj. R2, about 60 % of the DOC variability could be explained by the discharge, and the percentage was very similar for the three years. However, the mean residuals for the three linear models were quite different. Tables 2 and 3 suggest that the greater mean residuals were accompanied by a wider concentration range. Large concentration fluctuations would lead to a decreased average predictive ability by discharge. DOC concentrations were positively related to turbidity and negatively related to conductivity (n= 68, p < 0.01) while no significant relationship was found between the concentrations and air temperature or the soil temperatures of the active layer.
Table 2Results of covariance analysis (ANCOVA) between discharge and the DOC concentrations for the 2012–2014 sampling periods.
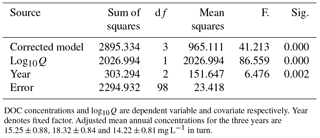
DOC concentrations and log10Q are dependent variable and covariate respectively. Year denotes fixed factor. Adjusted mean annual concentrations for the three years are 15.25 ± 0.88, 18.32 ± 0.84 and 14.22 ± 0.81 mg L−1 in turn.
3.3 Fluorescence indices
The three spectral indices varied considerably with discharge during the growing seasons (Fig. 5). There was a significant positive correlation between the HIX and logarithmic discharge but both FI and BIX were negatively correlated with discharge for the three years (Fig. 6). HIX ranged from 5.52 to 16.41 with an average value of 10.38, revealing a high proportion of more humified components in the stream discharge DOC. FI and BIX values ranged from 1.43 to 1.62 and from 0.46 to 0.63 with average values of 1.52 and 0.54, respectively. The FI values indicate that DOC originated both from plant-derived organic matter and from microbial-mediated organic matter, while the BIX value denotes the presence of a low proportion of young organic matter from biological sources in the discharge (see Huguet et al., 2009). All three indices were closely related to DOC concentrations and hydrological variables during the entire study period. Only HIX also showed a significant relationship with soil temperature (Table 4). In spite of the great variations during the three growing seasons, the mean annual values of the three indices did not differ statistically for the three years according to the covariance analysis, which eliminated the influence of discharge (Table 5).
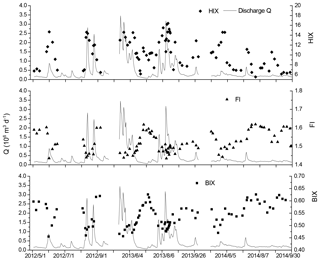
Figure 5Dynamics of the three spectral indices following discharge (Q) during the 2012–2014 sampling period.
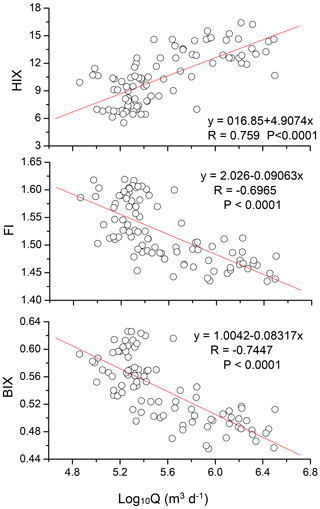
Figure 6Relationships between discharge (Q) and the three indices during the 2012–2014 sampling period.
Table 4Correlation analysis of the three fluorescence indices with hydrological and climatic factors.
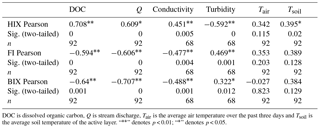
DOC is dissolved organic carbon, Q is stream discharge, Tair is the average air temperature over the past three days and Tsoil is the average soil temperature of the active layer. “” denotes p < 0.01; “*” denotes p < 0.05.
3.4 Concentrations and fluorescence indices of soil water
During the growing seasons of 2013, soil porewater DOC concentrations at the three points presented similar vertical profiles. Maximum DOC concentrations were typically found at the depth of 20–30 cm in the organic soil layer, while minimum values were found at the deeper mineral layer (Fig. 7). The vertical variation in DOC concentrations could be clearly observed when the active layer reached the maximum depth in the early autumn. However, no significant relationship was detected between DOC concentration and soil temperature at different depths. The DOC concentrations in the upper organic soil layer increased considerably from early to late June, but they did not change significantly from July to late August. Across the entire growing seasons, no significant relationship was found between the mean DOC concentration and the mean soil temperature in the whole profile (p > 0.05, n= 4).
The HIX, FI and BIX of soil porewater varied greatly with soil depth (Fig. 8). Pronounced changes in the three indices generally occurred at the depth where organic soil transitioned to the mineral soil. HIX values gradually decreased with depth, while FI and BIX increased with depth. The fluorescence indices in the upper organic soil layer changed significantly during the growing seasons. However, no consistency from spring to autumn was found. The mean values for the three indices were 16.62, 1.41 and 0.46, respectively, in 2013. The data indicated a much higher HIX level and a lower FI and BIX level in soil porewater than in the baseflow stream discharge. HIX values were significantly and positively correlated with DOC concentrations in soil porewater while FI and BIX were significantly but inversely correlated with DOC concentrations (n= 18, p < 0.01).
4.1 DOC concentrations and yield
DOC concentrations in boreal rivers have been reported to vary considerably according to differences in hydrology, soil type and topography (Andersson and Nyberg, 2008; Tunaley et al., 2016; Broder et al., 2017). Theoretically, the presence of organic soils in the catchment should contribute to higher DOC concentrations in connected rivers. However, no direct relationship between organic soil content and mean DOC concentration was extensively found across the boreal regions. In our study, the annual mean concentration, 15.4 mg L−1, was in the middle of the range of concentrations reported from boreal regions, from 1.5 to 35.3 mg L−1 (Yates et al., 2016; Avagyan et al., 2016). The DOC yield from our catchment was estimated at 4.7 g m−2 yr−1, which was in the lower range of estimates reported for a permafrost region, which ranged from 1 to 35 g m−1 yr−1 (Fraser et al., 2001; Dinsmore et al., 2010; Moody et al., 2016). The mean DOC yield in our catchment was less than the net DOC loss reported from UK lands (2.1–11.5 g m−2 yr−1) (Moody et al., 2013), but it was higher than in Finnish rivers (3.5 g m−2 yr−1) (Räike et al., 2012), in the Yukon River in Alaska (1.4–3.7 g m−2 yr−1) (Striegl et al., 2007) and in central Siberian rivers (2.8–4.7 g m−2 yr−1) (Prokushkin et al., 2011). Pan-Arctic rivers exported 32 Tg C yr−1 of DOC to the Arctic Ocean according to estimates by Kickighter et al. (2013), which indicated a mean yield of 5.1 g m−2 yr−1 from the north Eurasian permafrost. Our results indicated there was a slightly lower DOC yield in the southern part of the Eurasian permafrost. However, our data are representative only of the region in northeastern China. More field studies are needed to better estimate DOC loads from the entire south Eurasian permafrost region.
Miao (2014) estimated that the net ecosystem exchange (NEE) between peatland surfaces and the atmosphere using both carbon dioxide and methane fluxes was 30.59 ± 1.98 g m−2 yr−1 in the study catchment. Therefore, the estimated DOC yield in our study accounted roughly for 18.3 % of the net ecosystem carbon balance in the entire catchment. As the upland mountains, extensively covered by mineral soils, likely export little DOC to the stream compared with the peatland, the actual DOC yield based on the extent of the peatland will be much higher than 4.7 g m−2 yr−1. Therefore, the yield generated by our study is a very conservative estimate. Even so, our data still demonstrated the significant contribution of stream carbon export to the net peatland ecosystem carbon balance. Any disturbance altering DOC export processes and magnitudes will disrupt the balance between carbon sequestration and release in the Eurasia peatlands. The proportion of stream carbon export in our study was much higher than that in a northwest Russia river for which the DOC exported by streamflow accounted for 5.6–8.5 % of the total carbon sequestration in the peatlands (Avagyan et al., 2016), but it was close to a peat catchment in Scotland, in which DOC represented a loss of 24 % of NEE (Dinsmore et al., 2010).
4.2 Flow pathways, DOC sources and chemical characteristics
Peatlands in permafrost regions generally experience subsurface flows but not overland flows due to high rainfall infiltration into the thawed organic layer (Carey and Woo, 1997). In our study, the porosity of peat in the upper 40 cm layer was between 20 and 60 %, which would necessarily allow rapid and high infiltration of rainfall. The infiltrating rainfall was blocked from further vertical flow by the frozen soil, and as a result, it flowed laterally towards the stream. Lateral subsurface flow was an essential prerequisite for the positive relationship between discharge and the DOC concentrations to avoid the dilution effect which would result from overland flow (Guo et al., 2015).
The three fluorescence indices, HIX, FI, and BIX, exhibited considerable fluctuations during rainfall-runoff events in our study, implying shifts in DOC sources and chemical characteristics during subsurface flow. The three indices varied vertically within the organic soil layer up to the mineral layer. Vertical trends were maintained throughout the growing season of 2013 (Fig. 8). This pattern made the indices good indicators of DOC sources. Given the significant correlation between the indices and discharge, we concluded that the DOC released during flood periods originated mostly from the upper organic soil layer, whereas the DOC during recession and baseflow periods originated mainly from the lower mineral layer. Carey and Woo (2001) described permafrost soil as a two-layer flow system based on the difference in hydraulic conductivity between the upper organic soil and lower mineral soil: quickflow, defined as matrix flow or preferential flow in interconnecting soil pipes and rills, took place in the highly porous peat in the upper layer, while slowflow was laminar flow in the lower saturated mineral soils where flow velocities were orders of magnitude lower than quickflow. As the porosity declined exponentially in the transition from organic to mineral soil in the studied peatland, soil porewater in the upper organic soil with high concentrations of DOC should be transported by quickflow during floods, while baseflow conditions had much lower DOC concentrations from the deeper mineral soil.
Seasonal shifts in DOC sources and composition have been reported in previous studies in permafrost catchments (Spencer et al., 2008; O'Donnell et al., 2010). However, our results highlighted the importance of the shifts temporally during runoff event. In our study, the deepening of the soil active layer with thawing led to the presence of vertical discontinuity in hydraulic conductivity in the discharge-yield profile and to shifts in DOC sources and chemical characteristics during runoff. Because there were only a few rainfall events in 2014, we were able to identify the effects of the gradual deepening of the active layer during the growing season as the thawing proceeded. Significant increases in BIX and FI values during baseflow occurred from the spring to the autumn in 2014 (Fig. 4). This implied a concomitant increase in the proportion of microbial-derived DOC as the active layer deepened. During thawing of the active layer, the hydraulic residence time and the DOC mineralization rate, as well as physical adsorption in the mineral soil, would increase (Cronan and Aiken, 1985; Sebestyen et al., 2008), and this would alter DOC chemical characteristics under baseflow conditions. The fact that DOC in soil porewater exhibited higher HIX values and lower FI and BIX values compared to those in the baseflow discharge was direct proof of adsorption–mineralization in the mineral soil layer. DOC humification decreased and microbial-derived components increased when DOC was delivered by slowflow across the mineral soil. Our result is consistent with the study of Prokushkin et al. (2007) who also found higher levels of microbially transformed and/or derived material export due to the presence of a deeper active layer in the summer and autumn in Siberia. Changes in biochemical composition (decreases in the lignocellulose complex and increases in the hydrophilic fraction) were also confirmed by Kawahigashi et al. (2004). Based on these observations, a 9–11 % reduction in DOC load due to permafrost degradation was predicted in the Yukon River by 2050 (Walvoord and Striegl, 2007), and an increase in dissolved inorganic carbon was also hypothesized (Striegl et al., 2005). From these observations we infer that deepening of the active layer in a warming climate conceivably could reduce DOC export by baseflow as well as alter DOC chemical characteristics to more structure-simple microbial-derived components in the study region.
The HIX index for DOC showed no clear seasonal trend in the anomalously dry year of 2014. Even a minor flood caused a large increase in HIX, implying the sensitivity of DOC chemical characteristics to the shift in the primary flow path from quickflow to slowflow. The previous analysis had highlighted the importance of the seasonal thawing of the active layer to flow paths and DOC chemical characteristics. Covariance analysis showed that the discharge quantity was the sole factor leading to interannual variations in the DOC chemical characteristics. There was no significant difference in the maximum thaw depths of the active layer for any of the three years (Fig. 2), which was likely the reason why an interannual effect of active layer thawing could not be distinguished. In total, we conclude that there were different controlling factors on DOC chemical characteristics depending on different temporal scales. Long-term field investigations are crucially needed to evaluate the influence of permafrost thaw on prevailing flow paths and chemical characteristics of DOC in depth.
4.3 Discharge and DOC export
We found a significant positive relationship between DOC concentration and stream discharge, which is consistent with results observed in other permafrost regions (Hinton et al., 1998; Petrone et al., 2007; Balcarczyk et al., 2009; Koch et al., 2013). The positive relationship occurred in both the wet and the dry years, suggesting that DOC export from the studied catchment is a transport-limited process. Specifically, DOC transport capacity was mainly related to processes that controlled runoff, for example flow path, rate of runoff and lag time. As described earlier, the flow path shift was an equally important mechanism contributing to the positive relationship between discharge and DOC concentrations. Our conclusion is supported by both DOC model simulations (Neff and Asner, 2001; Wu et al., 2014) and field experiments (Tipping et al., 1999), reporting linear increases in DOC concentrations with increasing amounts of subsurface flow.
It may be hypothesized that DOC is being generated in the peatland in amounts large enough to make up for the loss of the DOC exported by successive floods in the growing seasons. It is noteworthy that DOC concentrations were consistently high in stream discharge during successive big floods in the autumn of 2012 and the spring of 2013. Meanwhile, the DOC concentrations in the organic soil layer of the peatland remained at a stable high level about 40 mg L−1 through the growing seasons. Successive rainfalls and seasonal temperature variations did not result in decreased concentrations in the peatland (Fig. 7). These data demonstrate the large DOC productive potential of the peat soil.
The regression slopes for the positive relationships between DOC and discharge showed large interannual variations (Fig. 4). The results of covariance analysis indicated that were other factors besides discharge quantity that underlay interannual variations. The high sensitivity of DOC production and degradation to temperature was well established (see Kalbitz et al., 2000; Moore et al., 2008). However, in our study no significant relationships were found between the mean temperature and the mean residual concentrations in the regression formulations for all three years (Table 3). Therefore temperature could not explain the residual variations. Precipitation also was shown to be of great importance for DOC dynamics in boreal peatlands (for example Olefeldt et al., 2013; Pumpanen et al., 2014). Discharge during flood events can mobilize large quantities of pre-event water stored in the riparian zone (Kirchner, 2003; Winterdahl et al., 2011), and both the time interval of two successive rainfalls and the quantity of the antecedent rainfall could influence DOC concentrations in the second flood. It is possible that rainfall frequency during the growing seasons could exert a cumulative effect on annual DOC dynamics. However, our data did not provide conclusive evidence and a more detailed and longer study is needed in this regard. Nevertheless, the positive relationship between annual discharge and annual mean DOC concentrations (p < 0.05, n= 3) (Fig. 9) provided a simple tool to estimate annual DOC load in the catchment.
Eurasian permafrost serves as an important potential carbon pool for the atmosphere and for linked aquatic and ocean ecosystems. Investigations of DOC responses in permafrost peatland could be used to predict the ecological consequences of climatic change in these regions. Our study investigated the loads and determinants of DOC export from a peatland catchment along the southern margins of Eurasian permafrost. The catchment exhibited a relatively low DOC load compared to other permafrost regions, and the yield estimates were an important contribution for estimating global fluvial carbon export. DOC export in our study catchment was a transport-limited process, as indicated by the positive correlation between discharge and DOC concentrations in both wet and dry years. Field investigations indicated that the source of the DOC and its chemical characteristics were greatly influenced by the flow path shifts between the upper organic soil layer and the lower mineral layer. The shifts were closely related to the vertical soil structure and seasonal thawing of the active layer. Deepening of the active layer following permafrost degradation would increase the content of microbial-originated DOC in baseflow discharge by increasing the relative contribution from the lower mineral soil layer to the DOC pool. Our study has provided limited field data on DOC dynamics in the southern region of Eurasian permafrost. Additional, more intensive studies are needed to improve our understanding and predictions of the dynamics of DOC under future climate change.
The data are not publicly accessible according to the requirements for confidentiality of hydrological data in Northeast China.
The authors declare that they have no conflict of interest.
This article is part of the special issue “Understanding and predicting Earth system and hydrological change in cold regions”. It is not associated with a conference.
The work was supported by the National Key Research and Development Program of
China (2016YFA0602303), the National Natural Science Foundation of China
(41571097, 41730643), the Key of Frontier Sciences, Chinese Academy of Sciences
(QYZDJ-SSW-DQC013), and the Research Program of Northeast Institute of Geography and
Agroecology, Chinese Academy of Sciences (IGA-135-05).
Edited by: Sean Carey
Reviewed by: David
Olefeldt and two anonymous referees
Ågren, A., Haei, M., Köhler, S. J., Bishop, K., and Laudon, H.: Regulation of stream water dissolved organic carbon (DOC) concentrations during snowmelt; the role of discharge, winter climate and memory effects, Biogeosciences, 7, 2901–2913, https://doi.org/10.5194/bg-7-2901-2010, 2010.
Andersson, J.-O. and Nyberg, L.: Spatial variation of wetlands and flux of dissolved organic carbon in boreal headwater streams, Hydrol. Process., 22, 1965–1975, 2008.
Avagyan, A., Runkle, B. R. K., Hennings, N., Haupt, H., Virtanen, T., and Kutzbach, L.: Dissolved organic matter dynamics during the spring snowmelt at a boreal river valley mire complex in Northwest Russia, Hydrol. Process., 30, 1727–1741, 2016.
Balcarczyk, K. L., Jones Jr., J. B., Jaffé, R., and Maie, N.: Stream dissolved organic matter bioavailability and composition in watersheds underlain with discontinuous permafrost, Biogeochemistry, 94, 255–270, 2009.
Broder, T., Knorr, K.-H., and Biester, H.: Changes in dissolved organic matter quality in a peatland and forest headwater stream as a function of seasonality and hydrologic conditions, Hydrol. Earth Syst. Sci., 21, 2035–2051, https://doi.org/10.5194/hess-21-2035-2017, 2017.
Carey, S. and Woo, M. K.: Snowmelt hydrology of two subarctic slopes, Southern Yukon, Canada, in: Proceedings of the Eleventh Northern Research Basins Symposium and Workshop (vol. 2), Prudhoe Bay/Fairbanks Alaska, The Water and Environmental Research Centre, University of Alaska, Fairbanks, 15–35, 1997.
Carey, S. K. and Woo, M. K.: Slope runoff processes and flow generation in a subartic, subalpine catchment, J. Hydrol., 253, 110–129, 2001.
Cory, R. M., Ward, C. P., Crump, B. C., and Kling, G. W.: Sunlight controls water column processing of carbon in arctic fresh waters, Science, 345, 925–928, 2014.
Cronan, C. S. and Aiken, G. R.: Chemistry and transport of soluble humic substances in forested watersheds of the Adirondack Park, New York, Geochim. Cosmochim. Ac., 49, 1697–1705, 1985.
Dinsmore, K. J., Billett, M. F., Skiba, U. M., Rees, R. M., Drewer, J., and Helfter, C.: Role of the aquatic pathway in the carbon and greenhouse gas budgets of a peatland catchment, Glob. Change Biol., 16, 2750–2762, 2010.
Fraser, C. J. D., Roulet, N. T., and Moore, T. R.: Hydrology and dissolved organic carbon biogeochemistry in an ombrotrophic bog, Hydrol. Process., 15, 3151–3166, 2001.
Frey, K. E. and McClelland, J. W.: Impacts of permafrost degradation on arctic river biogeochemistry, Hydrol. Process., 23, 169–182, 2009.
Guo, Y. D., Song, C. C., Wan, Z. M., Tan, W. W., Lu, Y. Z., and Qiao, T. H.: Effects of long-term land use change on dissolved carbon characteristics in the permafrost streams of northeast China, Environ. Sci.: Processes Impacts, 16, 2496–2506, 2014.
Guo, Y. D., Song, C. C., Wan, Z. M., Lu, Y. Z., Qiao, T. H., Tan, W. W., and Wang, L. L.: Dynamics of dissolved organic carbon release from a permafrost wetland catchment in northeast China, J. Hydrol., 531, 919–928, 2015.
Hinton, M. J., Schiff, S. L., and English, M. C.: Sources and flowpaths of dissolved organic carbon during storms in two forested watersheds of the Precambrian Shield, Biogeochemistry, 41, 175–197, https://doi.org/10.1023/A:1005903428956, 1998.
Huguet, A., Vacher, L., Relexans, S., Saubusse, S., Froidefond, J. M., and Parlanti, E.: Properties of fluorescent dissolved organic matter in the Gironde Estuary, Org. Geochem., 40, 706–719, 2009.
Jin, H. J., Li, S. X., Cheng, G. D., Wang, S. L., and Li, X.: Permafrost and climatic change in China, Global Planet. Change, 26, 387–404, 2000.
Kalbitz, K., Soliiger, S., Park, J. H., Michalzik, B., and Matzner, E.: Controls on the dynamics of dissolved organic matter in soils: A review, Soil Sci., 165, 277–304, 2000.
Kalbitz, K., Schwesig, D., Rethemeyer, J., and Matzner, E.: Stabilization of dissolved organic matter by sorption to the mineral soil, Soil Biol Biochem., 37, 1319–1331, 2005.
Kawahigashi, M., Kaiser, K., Kalbitz, K., Rodionov, A., and Guggenberger, G.: Dissolved organic matter in small streams along a gradient from discontinuous to continuous permafrost, Glob. Change Biol., 10, 1576–1586, 2004.
Kicklighter, D. W., Hayes, D. J., McClelland, J. W., Peterson, B. J., McGuire, A. D., and Melillo, J. M.: Insights and issues with simulating terrestrial DOC loading of Arctic river networks, Ecol. Appl., 23, 1817–1836, 2013.
Kirchner, J. W.: A double paradox in catchment hydrology and geochemistry, Hydrol. Process., 17, 871–874, 2003.
Koch, J. C., Runkel, R. L., Striegl, R., and McKnight, D. M.: Hydrologic controls on the transport and cycling of carbon and nitrogen in a boreal catchment underlain by continuous permafrost, J. Geophys. Res.-Biogeo., 118, 698–712, https://doi.org/10.1002/jgrg.20058, 2013.
Laudon, H., Berggren, M., Ågren, A., Buffam, I., Bishop, K., Grabs, T., Jansson, M., and Köhler, S.: Patterns and dynamics of dissolved organic carbon (DOC) in boreal streams: the role of processes, connectivity, and scaling, Ecosystems, 14, 880–893, 2011.
Lessels, J. S., Tetzlaff, D., Carey, S. K., Smith, P., and Soulsby, C.: A coupled hydrology-biogeochemistry model to simulate dissolved carbon exports from a permafrost-influenced catchment, Hydrol. Process., 29, 5383–5396, 2015.
Lyon, S. W., Destouni, G., Giesler, R., Humborg, C., Mörth, M., Seibert, J., Karlsson, J., and Troch, P. A.: Estimation of permafrost thawing rates in a sub-arctic catchment using recession flow analysis, Hydrol. Earth Syst. Sci., 13, 595–604, https://doi.org/10.5194/hess-13-595-2009, 2009.
Lyon, S. W., Mörth, M., Humborg, C., Giesler, R., and Destouni, G.: The relationship between subsurface hydrology and dissolved carbon fluxes for a sub-arctic catchment, Hydrol. Earth Syst. Sci., 14, 941–950, https://doi.org/10.5194/hess-14-941-2010, 2010.
Mann, P. J., Davydova, A., Zimov, N., Spencer, R. G. M., Davydov, S., Bulygina, K., Zimov, S., and Holmes, R. M.: Controls on the composition and liability of dissolved organic matter in Siberia's Kolyma river basin, J. Geophys. Res.-Biogeo., 117, G01028, https://doi.org/10.1029/2011JG001798, 2012.
McKnight, D. M., Boyer, E. W., Westerhoff, P. K., Doran, P. T., Kulbe, T., and Andersen, D. T.: Spectrofluorometric characterization of dissolved organic matter for indication of precursor organic material and aromaticity, Limnol. Oceanogr., 46, 38–48, 2001.
Miao, Y. Q.: Net ecosystem carbon fluxes of peatland in the continuous permafrost zone, Great Hinggan Mountains, Dissertation, University of Chinese Academy of Sciences, 120 pp., 2014 (in Chinese).
Moody, C. S., Worrall, F., Evas, C. D., and Jones, T. G.: The rate of loss of dissolved organic carbon (DOC) through a catchment, J. Hydrol., 492, 139–150, 2013.
Moody, C. S., Worrall, F., and Burt, T. P.: Identifying DOC gains and losses during a 20-year record in the Trout Beck catchment, Moor House, UK, Ecol. Indic., 68, 102–114, 2016.
Moore, T. R., Paré, D., and Boutin, R.: Production of dissolved organic carbon in Canadian forest soils, Ecosystems, 11, 740–751, 2008.
Neff, J. C. and Asner, G. P.: Dissolved organic carbon in terrestrial ecosystems: synthesis and a model, Ecosystems, 4, 29–48, 2001.
O'Donnell, J. A., Aiken, G. R., Kane, E. S., and Jones, J. B.: Source water controls on the character and origin of dissolved organic matter in streams of the Yukon River basin, Alaska, J. Geophys. Res., 115, G03025, https://doi.org/10.1029/2009JG001153, 2010.
Ohno, T.: Fluorescence inner-filtering correction for determining the humification index of dissolved organic matter, Environ. Sci. Technol., 36, 742–746, 2002.
Olefeldt, D., Roulet, N., Giesler, R., and Persson, A.: Total waterborne c arbon export and DOC composition from ten nested subarctic peatland catchments-importance of peatland cover, groundwater influence, and inter-annual variability of precipitation patterns, Hydrol. Process., 27, 2280–2294, 2013.
Opsahl, S., Benner R., and Amon R. M. W.: Major flux of terrigenous dissolved organic matter through the Arctic Ocean, Limnol. Oceanogr., 44, 2017–2023, 1999.
Olefeldt, D. and Roulet, N. T.: Effects of permafrost and hydrology on the composition and transport of dissolved organic carbon in a subarctic peatland complex, J. Geophys. Res., 117, G01005, https://doi.org/10.1029/2011JG001819, 2012.
Park, Y. S., Engel, B. A., Frankenberger, J., and Hwang, H.: A-web-based tool to estimate pollutant loading uing LOADEST, Water, 7, 4858–4868, 2015.
Petrone, K. C., Jones, J. B., Hinzman, L. D., and Boone, R. D.: Seasonal export of carbon, nitrogen, and major solutes from Alaskan catchments with discontinuous permafrost, J. Geophys. Res., 111, G02020, https://doi.org/10.1029/2005JG000055, 2006.
Petrone, K. C., Hinzman, L. D., Shibata, H., Jones, J. B., and Boone, R.: The influence of fire and permafrost on sub-arctic stream chemistry during storms, Hydrol. Process., 21, 423–434, 2007.
Prokushkin, A. S., Gleixner, G., McDowell, W. H., Ruehlow, S., and Schulze, E. D.: Source and substrate-specific export of dissolved organic matter from permafrost-dominated forested watershed in central Siberia, Global Biogeochem. Cy., 103, 109–124, 2007.
Prokushkin, A. S., Pokrovsky, O. S., Shirokova, L. S., Korets, M. A., Viers, J., Prokushkin, S. G., Amon, R. M. W., Guggenberger, G., and McDowell, W. H.: Sources and the flux pattern of dissolved carbon in rivers of the Yenisey basin draining the Central Siberian Plateau, Environ. Res. Lett., 6, 045212, https://doi.org/10.1088/1748-9326/6/4/045212, 2011.
Pumpanen, J., Linden, A., Miettinen, H., Kolari, P., Ilvesniemi, H., Mammarella, I., Hari, P., Nikinmaa, E., Heinonsalo, J., Back, J., Ojala, A., Berninger, F., and Vesala, T.: Precipitation and net ecosystem exchange are the most important drivers of DOC flux in upland boreal catchments, J. Geophys. Res.-Biogeo., 119, 1861–1878, 2014.
Räike, A., Kortelainen, P., Mattsson, T., David N., and Thomas, D. N.: 36 year trends in dissolved organic carbon export from Finnish rivers to the Baltic Sea, Sci. Total Environ., 435–436, 188–201, 2012.
Sebestyen, S. D., Boyer, E. W., Shanley, J. B., Kendall, C., Doctor, D. H., Aiken, G. R., and Ohte, N.: Sources, transformations and hydrological processes that control stream nitrate and dissolved organic matter concentrations during snowmelt in an upland forest, Water Resour. Res., 44, W12410, https://doi.org/10.1029/2008WR006983, 2008.
Spencer, R. G., Mann, P. J., Dittmar, T., Eglinton, T. I., McIntyre, C., Holmes, R. M., Zimov, N., and Stubbins, A.: Detecting the signature of permafrost thaw in Arctic rivers, Geophys. Res. Lett., 42, 2830–2835, 2015.
Spencer, R. G. M., Aiken, G. R., Wickland, K. P., Striegl, R. G., and Hernes, P. J.: Seasonal and spatial variability in dissolved organic matter quantity and composition from the Yukon River basin, Alaska, Global Biogeochem. Cy., 22, GB4002, https://doi.org/10.1029/2008GB003231, 2008.
Striegl, R. G., Aiken, G. R., Dornblaser, M. M., Raymond, P. A., and Wickland, K. P.: A decrease in discharge-normalized DOC export by the Yukon River during summer through autumn, Geophys. Res. Lett., 32, L21413, https://doi.org/10.1029/2005GL024413, 2005.
Striegl, R. G., Dornblaser, M. M., Aiken, G. R., Wickland, K. P., and Raymond, P. A.: Carbon export and cycling by the Yukon, Tanana, and Porcupine rivers, Alaska 2001–2005, Water Resour. Res., 43, W02411, https://doi.org/10.1029/2006WR005201, 2007.
Tipping, E., Woof, C., Rigg, E., Harrison, A. F., Inneson, P., Taylor, K., Benham, D., Poskitt, J., Rowland, A. P., Bol, R., and Harkness, D. D.: Climatic influences on the leaching of dissolved organic matter from upland UK moorland soils, investigated by a field manipulation experiment, Environ. Inter., 25, 83–95, 1999.
Townsend-Small, A., McClelland, J. W., Max Holmes, R., and Peterson, B. J.: Seasonal and hydrologic drivers of dissolved organic matter and nutrients in the upper Kuparuk River, Alaskan Arctic, Biogeochemistry, 103, 109–124, 2011.
Tunaley, C., Tetzlaff, D., Lessels, J., and Soulsby, C.: Linking highfrequency DOC dynamics to the age of connected water sources, Water Resour. Res., 52, 5232–5247, 2016.
Vonk, J. E., Mann, P. J., Davydov, S., Davydova, A., Spencer, R. G. M., Schade, J., Sobczak, W. V., Zimov, N., Zimov, S., Bulygina, E., and Eglinton, T. I.: High biolability of ancient permafrost carbon upon thaw, Geophys. Res. Lett., 40, 2689–2693, 2013.
Walvoord, M. A. and Striegl, R. G.: Increased groundwater to stream discharge from permafrost thawing in the Yukon River basin: Potential impacts on lateral export of carbon and nitrogen, Geophys. Res. Lett., 34, L12402, https://doi.org/10.1029/2007GL030216, 2007.
Winterdahl, M., Futter, M., Köhler, S., Laudon, H., Seibert, J., and Kevin, B. K.: Riparian soil temperature modification of the relationship between flow and dissolved organic carbon concentration in a boreal stream, Water Resour. Res., 47, W08532, https://doi.org/10.1029/2010WR010235, 2011.
Wu, H., Peng, C., Moore, T. R., Hua, D., Li, C., Zhu, Q., Peichl, M., Arain, M. A., and Guo, Z.: Modeling dissolved organic carbon in temperate forest soils: TRIPLEX-DOC model development and validation, Geosci. Model Dev., 7, 867–881, https://doi.org/10.5194/gmd-7-867-2014, 2014.
Yates, C. A., Johnes, P. J., and Spencer, R. G. M.: Assessing the drivers of dissolved organic matter export from two contrasting lowland catchments, U.K, Sci. Total Environ., 569–570, 1330–1340, 2016.