the Creative Commons Attribution 4.0 License.
the Creative Commons Attribution 4.0 License.
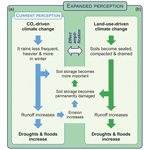
HESS Opinions: Floods and droughts – are land use, soil management, and landscape hydrology more significant drivers than increasing CO2?
Karl Auerswald
Juergen Geist
John N. Quinton
Peter Fiener
Floods, droughts, and heatwaves are increasing globally. This is typically attributed to CO2-driven climate change. However, at the global scale, CO2-driven climate change neither reduces precipitation nor adequately explains droughts despite the modest increase in evapotranspiration due to temperature rise. Past land-use changes, particularly soil sealing, compaction, and drainage, are likely to be more significant for water losses resulting from runoff and leading to flooding and water scarcity. The importance of these processes is generally poorly addressed in modelling because hydrological models rarely reflect lateral fluxes in the atmosphere, on the soil surface, and in the soil. Land use is only considered in coarse categories, and neighbourhood effects and feedback mechanisms are neglected. However, even if models fail and we cannot create landscape experiments, there is sufficient evidence that land use is a key driver of the problem and of the solution to mitigate floods, droughts, and heatwaves. Addressing land-use changes is imperative as they persist even with zero net CO2 emissions, making the world more vulnerable.
- Article
(6815 KB) - Full-text XML
-
Supplement
(667 KB) - BibTeX
- EndNote
Reports of severe storms; catastrophic floods like the Simbach event in southern Germany (Brandhuber et al., 2017; Mayr et al., 2020); and tragic events such as the Ahr Valley floods in western Germany, which caused over 150 casualties (Mohr et al., 2023), are increasingly common. These occurrences, alongside water shortages, droughts, and heatwaves (Ciais et al., 2005; Miralles et al., 2019), suggest a significant alteration in landscape hydrology, often attributed to CO2-driven climate change.
In public discourse, explanations for these climate-driven changes often revolve around statements such as “the soil dries out because of the heat” or “… because air humidity is so low,” as seen in the German Drought Monitor (https://www.ufz.de/index.php?en=37937, last access: 12 April 2025; Boeing et al., 2022). While these statements correlate with observations, they offer only circular reasoning, lacking a causal explanation. This can easily be recognized because the logic of the sentences can be reversed and still holds: “it is so hot because the soil is dry” (García-García et al., 2023). Even the plausible sentence “the soil is dry because it has not rained for a long time” is at least partly circular reasoning since terrestrial evapotranspiration globally contributes 40 % to terrestrial precipitation, with 57 % of the terrestrial evapotranspiration being recycled (van der Ent et al., 2010). Recycling ratios are higher in summer, when oceans are cold relative to the land, and in areas of low precipitation (van der Ent and Tuinenburg, 2017). Moisture recycling becomes especially large where large, intact woodland exists, extending recycling over thousands of square kilometres (Makarieva et al., 2013a, 2014). These values of moisture recycling, although large, are strongly biased towards minimum values because they only consider falling precipitation (snow, hail, and rain) while neglecting occult precipitation (dew, fog, rime), which, often, is of local, recycled origin (Kaseke et al., 2017). Occult precipitation can be significant and can reach several hundred millimetres per year (Zimmermann and Zimmermann, 2002; Ingraham and Mark, 2000; Migała et al., 2002; Jacobs et al., 2006).
To differentiate between spurious and causal relations, it is crucial to consider the fact that the water and energy balances are interconnected, sharing evapotranspiration as a common variable (Allen et al., 1998).
The water balance equation in its simplest version is
with P indicating precipitation; ET indicating evapotranspiration or latent heat when considered in relation to the energy balance; Q indicating runoff, either laterally (surface or subsurface runoff) or vertically (groundwater recharge); and ΔS indicating the variable filling of the soil store. The equation applies to all scales even though the relative importance of the different terms changes with the temporal and spatial scale.
The simplest version of the energy balance without lateral energy fluxes is expressed as follows:
The variables indicate incipient shortwave radiation (Rs); albedo (α), which is the fraction of reflected shortwave radiation; the net effect of incoming and outgoing long-wave radiation (Rnl); the enthalpy of evaporation (λ); the sensible heat flux (H); and the soil heat flux (G).
CO2-driven climate change directly impacts only Rnl in Eq. (2), while land use intentionally influences ET, Q, ΔS, α, and G, affecting both Eqs. (1) and (2) if we ignore the many cascading effects that follow in both cases. Albedo, for instance, is about 30 % larger for a straw-covered surface than for a bare-soil surface (Sharratt and Campbell, 1994). A straw cover would allow every farmer to preserve soil moisture for crops because less energy from shortwave radiation would be available to drive evapotranspiration. For France, it was estimated that, during the centennial European heatwave in August 2003, if the farmers had left the straw from grain harvest on the soil rather than tilling it in, the change of albedo would have lowered the temperature country-wide by, on average, 2 K (Davin et al., 2014). This heatwave was the deadliest natural disaster in Europe in the last few centuries, with the death toll exceeding 70 000 in Europe and about 20 000 in France alone (Robine et al., 2008). Further direct effects of straw cover would have contributed to a shorter, less intense drought due to lower soil humidity efflux; lower capillary rise to the evaporating surface due to the physical barrier by the straw cover; better infiltration during heavy rain due to less surface crusting; less erosion; and more dew formation due to better thermal isolation, reducing the soil heat flux during the night. Given the strong influence of soil and soil use over the water and energy balances, there is potential to compensate for some of the adverse effects that CO2 increases have on terrestrial environments. However, this option is not commonly used. Instead, land use is another principal driver of floods, droughts, and heatwaves.
In this opinion paper, we compare the effects of CO2-driven and land-use-driven climate change on floods and droughts and highlight the significant, but rarely considered, effects of land management on landscape hydrology. Floods encompass flash floods and fluvial floods. We will illustrate this for Bavaria (southern Germany) for two reasons. (i) We have access to a large consistent monitoring and modelling data set covering climate, land use and management, and hydrology, and (ii) the region represents a typical central European setting with predominantly agricultural land use (cropland and livestock farming) while also featuring extensive forest, urban areas, and protected natural reserves. We argue that the effects shown here for southern Germany will occur globally, although there will be regional differences in land-use setting and agricultural management, for example, differences in agricultural machinery weights, the soil sealing area, or the road density. A short description of the example area can be found in Sect. S1 in the Supplement.
Globally, an increase in annual precipitation of 2 % K−1 to 3 % K−1 temperature increase can be expected (Lambert and Webb, 2008; Roderick et al., 2014; Bürger et al., 2014; Skliris et al., 2016). This value results from a 7 % K−1 increase in the moisture-carrying capacity of the air, called the Clausius–Clapeyron (CC) rate, and from the constant energy provided by the radiation driving evapotranspiration. Individual rains require a saturated atmosphere following the CC rate, and, during intense rains, the CC rate can even be exceeded. The increase in rainfall intensity can reach 14 % K−1, referred to as the super-CC rate (Westra et al., 2014). This effect is particularly pronounced at temperatures ranging from 12 to 22 °C (Lenderink and van Meijgaard, 2008). The increase in rainfall intensity exceeding the CC rate can be attributed to an intensification of cloud dynamics (Loriaux et al., 2013; Westra et al., 2014), sometimes coupled with the transition from stratiform to convective regimes (Haerter and Berg, 2009). This increase in rain intensity arises from higher surface temperatures and, in particular, from the higher release of sensible heat during condensation of moister air. This additional energy augments the updraught strength of the convective cell (Loriaux et al., 2013; Westra et al., 2014), leading to an increased influx of moist air, which is further enhanced due to the loss of vapour volume due to rain condensation (Makarieva et al., 2013b).
In addition to the general trends of rising temperature and increasing precipitation, many studies suggest that the unusually persistent and amplified disturbances in the jet stream are associated with persistent extreme weather events leading to floods or droughts. These persistent events have been related to high-amplitude quasi-stationary atmospheric Rossby waves resulting from quasi-resonant amplification. However, there is considerable variation among climate models regarding this effect. Some predict a near-tripling of quasi-resonant amplification events by the end of the century, while others predict a potential decrease (Mann et al., 2018).
In Bavaria, the changes in air temperature during the last 7 decades have been pronounced as annual mean temperature has risen from about 7.5 to 9 °C. Temperatures started to change circa 1980 and increased almost linearly (Fig. 1). Spatially, this increase was uniform. The temporal changes in precipitation, evapotranspiration, direct runoff, and groundwater recharge were minor compared to the spatial variation and were without a clear trend (Fig. 1). Between 1950 and 2015, modelled groundwater recharge decreased by a total of 22 mm yr−1 (i.e. on average, it decreased by 0.33 m yr−1 every over the last 65 years), and actual evapotranspiration decreased by a total of 7 mm yr−1, while precipitation decreased by a total of 53 mm yr−1. These changes occurred spatially and relatively uniformly, implying that the relative changes were larger in the drier areas.
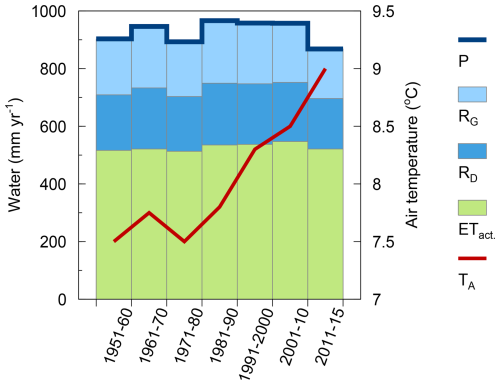
Figure 1Change in air temperature (TA), falling precipitation (P), actual evapotranspiration (ETact), direct runoff (RD), and groundwater recharge (RG) during the past 7 decades and averaged over the entirety of Bavaria (approximate area of 71 000 km2); the data were compiled from Baumeister et al. (2017), who applied a hydrological model (GWN-BW) specifically designed to calculate groundwater recharge. Like almost all hydrological models, the model considers only coarse land-use classes and does not account for the changes that occurred within the land uses. Furthermore, it does not consider lateral interactions between land uses (e.g. oasis and clothesline effects on evapotranspiration, run-on infiltration, and interflow). Hence, the model results almost entirely depict meteorological changes in space and time.
Only a marginal decrease could be detected in summer rainfall between 1950 and 2020 (Fig. 2). Since 1900, the five driest summers (in ascending order: 1911, 1904, 1952, 2003, 1947) and the five wettest summers (in descending order: 1926, 1924, 1966, 1954, 1948) show no CO2-related pattern. Winter precipitation has increased slightly since 1950. Due to the opposing trends of summer and winter and the unaltered spring and autumn rainfall, annual precipitation has hardly changed (ByStMUV, 2021). Future climate projections for RCP8.5 until 2050 also do not suggest alarming changes in summer rainfall (RCP denotes the representative concentration pathway, and RCP8.5 reflects business as usual; for RCP scenarios, see van Vuuren et al., 2011). Furthermore, in the latest analysis of return periods of heavy rainfall by the German Weather Service, covering 65 years until 2020, stationarity still had to be assumed for all 22 analysed event durations, spanning from 5 min to 7 d, despite the application of sophisticated statistical tools to detect a CO2-driven climate change signal (Haberlandt et al., 2023; Willems et al., 2023; Shehu et al., 2023). These minor changes in annual rainfall, seasonal rainfall, or event rainfall do not align with the severity of floods and droughts experienced.
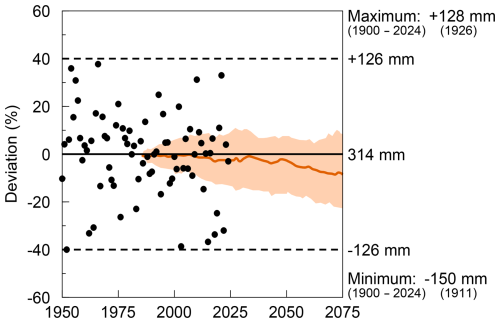
Figure 2Deviations of summer rainfall (June, July, August) in Bavaria (approximate area of 71 000 km2) from the average of 1971 to 2000 (being equal to the average of 1900 to 2024); black circles and black curves show measurements, while the orange-shaded area and orange curve show the bandwidth and the median of the projections for RCP8.5; data are redrawn from ByStMUV (2021); these are complemented by the years 2020 to 2024, taken from DWD (2025), which was also used by ByStMUV (2021).
The only measured parameter except for air temperature, which already exhibits a CO2-driven climate change signal, is annual rain erosivity (Fiener et al., 2013; Auerswald et al., 2019a). Erosivity is not a hydrological parameter but quantifies the ability of rains to detach soil particles and transport them. It is the product of the amount and intensity of rain exceeding certain thresholds (Wischmeier, 1959; Wischmeier and Smith, 1958). As such, it is more sensitive to changes in cloud dynamics but also to the transition of snow to rain than the amount of rain, which is important for hydrology. Rainfall erosivity has doubled in Germany since the 1960s (Auerswald et al., 2019a, b; Winterrath 2023), and projections for RCP8.5 indicate further increases (Auerswald et al., 2019c; Fig. 3a). Also, new convection-permitting climate simulations show a similar trend (Uber et al., 2024).
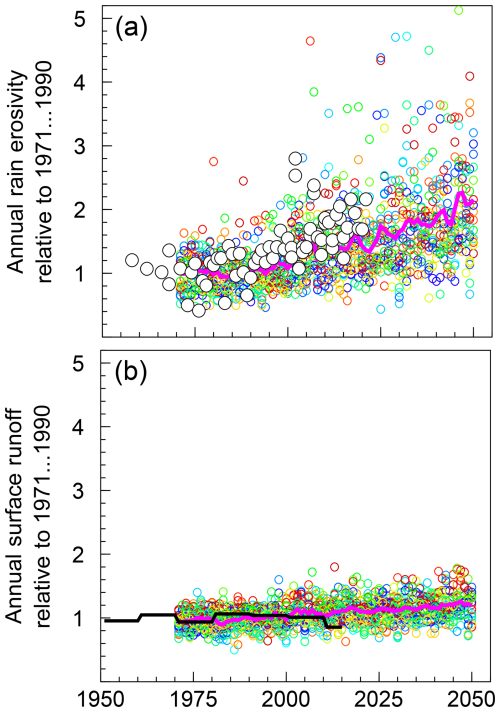
Figure 3Change in annual rain erosivity (a) and surface runoff (b) relative to the mean from 1971 to 1990. Coloured symbols (taken from Auerswald et al., 2019c) were calculated from an ensemble of 10 projections approved by the German Weather Service following RCP8.5. Each colour indicates one of the 10 climate projections. See the Appendix for the climate projections, methods, and covered area. The magenta line is a 3-year moving average of 10 projections (thus being equivalent to a 30-year normal period). The black symbols (a) show the measured erosivities taken from Auerswald et al. (2019a) and Winterrath (2023). The black line (b) shows the 10-year means of a full hydrologic model based on measured meteorological data (taken from Baumeister et al., 2017; for other hydrologic parameters resulting from this model, see Fig. 1).
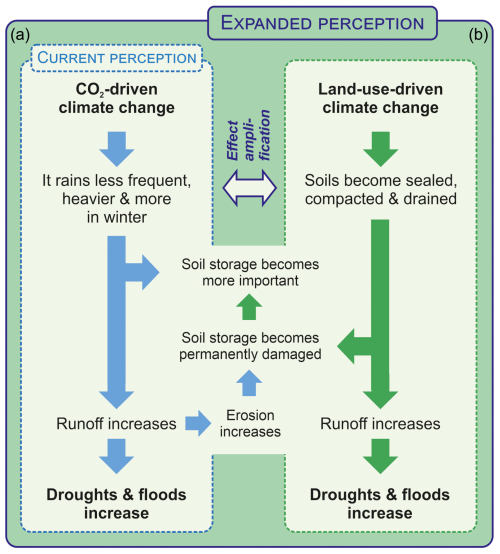
Figure 4Current perception of the influence of CO2-driven climate change on rain and subsequent droughts and floods (a) and expanded perception, including land-use-driven climate change (b), and their interactions.
Thus, under climatic conditions typical for central Europe until now, the CO2-driven climate change has mainly caused an increase in rain erosivity, eventually promoting soil crusting and, in turn, causing infiltration-excess runoff that contributes to flooding. Subsequently, drought may result because the runoff does not reach the soil store, and, in the long-term, the soils' storage capacity is impaired due to increasing erosion (Fig. 4a shows the conceptual mechanism). This reduces evapotranspiration and temperature increases. The increasing temperature amplifies the drought: the so-called “event self-intensification”” (Miralles et al., 2019). As the drier area heats up more than neighbouring well-watered areas, the higher temperature and air of low humidity spread to these nearby areas and cause them to transpire more until they also run short of water. Thus, the area with reduced evapotranspiration grows and may finally spread over an entire continent, described as “event self-propagation” by Miralles et al. (2019). Even though persistent large-scale circulation anomalies are critical for the initiation of drought and heatwaves (Miralles et al., 2019), the length of time required for such anomalies to initiate droughts or heatwaves will depend on the moisture storage on the ground and whether or not it is sufficient to bridge the anomaly.
Modelling suggests that other mechanisms besides CO2-driven climate change must significantly contribute to floods and drought. Studies modelling surface runoff with the curve number model of the USA Soil Conservation Service (Woodward et al., 2002; NRCS, 2004) while assuming otherwise time-invariant soil and land-use conditions (see Sect. S2 in the Supplement) yielded a 20 % increase in yearly surface runoff by 2050 (Fig. 3b), while, for the same projections, erosivity is expected to increase by 100 % (Fig. 3a). This expected runoff increase appears to be moderate compared to the interannual variation. Hydrological modelling using measured meteorological data between 1950 and 2015 confirms that changes in annual surface runoff driven by changes in meteorological conditions are minor (Fig. 1) and do not support the notion that CO2-driven climate change is the main cause of the increasing frequency of floods and droughts. Also, the CO2-driven increase in evapotranspiration due to rising temperatures cannot explain droughts because evapotranspiration rises only modestly by 2 % K−1 to 3 % K−1 temperature increase (Lambert and Webb, 2008; Roderick et al., 2014; Bürger et al., 2014; Skliris et al., 2016). Therefore, a 2 K temperature rise would increase evapotranspiration by only 4 % to 6 %, which should be buffered by functioning soils in humid areas. Consequently, hydrological modelling indicated no increase in evapotranspiration during the last 7 decades (Baumeister et al., 2017; Fig. 1). At present, CO2-driven climate change's influence on the loss of water by surface runoff or on evapotranspiration does not explain why floods and droughts are already severe today.
Land use has significantly changed over the past 2 centuries, particularly since World War II. Many soils have been sealed by pavement or roofing; in addition, agricultural soils have been compacted, and drainage systems have been introduced. Sealing, compaction, and drainage lead to rapid water runoff and flooding as less water enters the soil (Fig. 3b). This decreases evapotranspiration and increases temperature. The effects appear to be almost identical to those induced by the CO2-driven climate change. Thus, these effects can easily be misinterpreted with regard to their causes and require closer examination.
3.1 Soil sealing
Excluding farm and forest roads, each inhabitant in Bavaria is jointly responsible for sealing approximately 330 m2 of soil (Üreyen and Thiel, 2017), which, in total, accounts for about 6.0 % of the total land area. Although this percentage may not seem substantial at first glance, it has significant implications. Assuming all rainfall runs off from this area, an average annual precipitation of 938 mm yr−1 (Baumeister et al., 2017) means that, on average, 56 mm yr−1 is directly converted to runoff equal to the mean precipitation in March. Notably, this precipitation loss exceeds what can be expected from CO2-driven climate change (compare to Fig. 1). Except for the small amount of water left on sealed surfaces after a rain, sealed surfaces do not contribute to evaporation but rather partition their radiant energy uptake almost exclusively into sensible heat (Oke, 1982). Thus, 6 % of the overall mean actual evapotranspiration of 528 mm yr−1 (Baumeister et al., 2017) results in a calculated evaporation loss of 32 mm yr−1. To put this into perspective, the energy required to evaporate 1 mm of water (1 L m−2) can heat the atmosphere above 1 m2 of ground approximately by 10 K to a height of around 200 m. Consequently, a loss of 32 mm of evaporation would theoretically lead to a 320 K increase in temperature across a 200 m high air column over Bavaria. However, this extreme scenario does not occur due to increased evapotranspiration from surrounding non-sealed areas (Zipper et al., 2017), known as the oasis effect (Allen et al., 1998) or the micro-oasis effect (Oke, 1982). Eddy diffusion or advection transfers energy from the sealed surface to adjoining areas (Calder, 1949; McNaughton, 1976; Klaassen and Claussen, 1995). Evapotranspiration in the surroundings of sealed surfaces can thus exceed even potential evapotranspiration due to the advection of warmer, drier air. This increase in evapotranspiration could be as much as 30 % (Oke, 1982) or even 50 % (Panin et al., 1998) and can be distributed over larger areas: in eddy covariance measurements, fetch areas of 100 times the instrument height are often assumed, but this grossly underestimates travel distances (Leclerc and Thurtell, 1990; Savage et al., 1996). Travel distances of eddy diffusion determined with tracers can be larger than 3 km (Drivas and Shair, 1974), while theoretical considerations show that the advective exchange can be substantial at up to 20 km or more (McNaughton, 1976). At shorter distances, evapotranspiration caused by advection can even be 90 % of the total evapotranspiration in some cases (Prueger et al., 1996). However, the effects on evaporation must not necessarily be greatest near a dry surface because stomatal conductivity decreases pronouncedly with an increasing vapour pressure deficit (Grossiord et al., 2020). Thus, the compensation may extend over large distances due to reduced stomatal conductivity near the source of dry air (Baldocchi and Rao, 1995). Even in rough vegetation like forests, where momentum fluxes decrease rapidly, heat fluxes extend over much larger distances (Klaassen et al., 2002). The reduced stomatal conductance under a high vapour pressure deficit leads to a cascade of subsequent impacts, including reduced photosynthesis and growth and higher risks of carbon starvation and hydraulic failure (Grossiord et al., 2020). This may also explain why yields are often reduced along roads (Raatz et al., 2019).
Consequently, agricultural land and forests must provide additional water for evapotranspiration to compensate for this societally induced increase in evaporative demand. It is known that the cooling effect of the vegetated area may extend up to 2 km into a built-up area (Yan et al., 2018). Thus, it can be safely assumed that at least most of the sensible heat produced by sealed surfaces in the countryside, where sealed surfaces occur as narrow street bands, isolated buildings, or small villages, is dissipated to the neighbouring vegetated areas. The old references given above (e.g. Calder, 1949; McNaughton, 1976) show that we have known the effects of advection and lateral interaction for many decades; however, except when in combination with the urban-heat-island effect or eddy covariance measurements, they are hardly considered in landscape planning or hydrological modelling.
Soils with limited water storage capacity may not meet this additional demand for evapotranspiration, particularly during dry years. This will lead to reduced evapotranspiration from these soils, resulting in increased temperatures above these soils. The remaining area has to compensate even more for the advective energy resulting from reduced evapotranspiration. The area showing a water shortage thus grows (event self-propagation). Additionally, it becomes warmer, and the effect intensifies. A heatwave and a drought may result only because no action was taken to compensate for the adverse effects of soil sealing.
Natural systems also contain areas that generate runoff and may experience droughts. Typically, every landscape includes wet depressions where this runoff accumulates, acting as buffers during dry spells. Unfortunately, many of these wet areas have been systematically drained, and no compensatory wetlands have been established to offset the increased generation of runoff due to soil sealing. Soil sealing, mainly at the expense of cropland, created the pressure to drain wet grassland and convert it to cropland (van der Ploeg et al., 1999, 2000; see Sect. S1.3).
Furthermore, sealed areas impede groundwater recharge. A sealing of 6 % reduces the overall mean groundwater recharge (206 m yr−1; Baumeister et al., 2017) by 12 mm yr−1. Neighbouring areas, if they compensate for the loss of 32 mm yr−1 in evapotranspiration (Blumröder et al., 2021; Herbst et al., 2007), will, in consequence, recharge 32 mm yr−1 less groundwater. Ultimately, this may lead to a calculated 44 mm yr−1 decrease in groundwater recharge if vegetated surfaces compensate for the entire loss of evaporation caused by sealed surfaces. A loss of 6 % of the area by sealing could thus potentially be converted to a loss of 21 % in groundwater recharge. This agrees with the declining water tables observed in many aquifers. Between 2000 and 2020, approximately 20 % of the 1600 monitored aquifers in Bavaria experienced a significant decline in water levels, while another 20 % declined slightly (Joeres et al., 2022).
3.2 Drainage
Landscape hydrology (Fig. 5) comprises the coupling of vertical fluxes (precipitation, infiltration, evapotranspiration, groundwater recharge) with lateral fluxes (surface and subsurface runoff, groundwater flow, air moisture transport). Although frequently neglected or overlooked in hydrological models, the lateral fluxes are crucial for exchanging water within a landscape (Arnault et al., 2021). Surface runoff often infiltrates while traversing the landscape (run-on infiltration; Woolhiser et al., 1996), contributing to groundwater recharge (Carroll et al., 2019; Fiener and Auerswald, 2003). Subsurface flow (interflow) laterally percolates through the soil, supplying water to lower slopes for extended periods without rain, eventually contributing to groundwater recharge (Carroll et al., 2019). This enables lower slopes to continue evaporating, even in rainless periods, increasing air humidity and thus alleviating water stress, including in upslope areas. Groundwater flow sustains riparian areas and rivers, both of which enhance air humidity and act as buffers (Auerswald et al., 2019d).
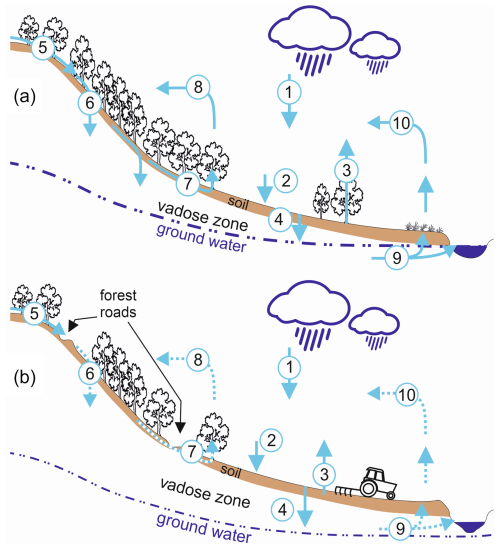
Figure 5Schematic representation: (a) intact landscape hydrology and (b) under contemporary conditions where lateral water flows have been strongly disturbed (dashed lines) by infrastructure and other interventions and where the groundwater was lowered (1 – precipitation, 2 – infiltration, 3 – evapotranspiration, 4 – groundwater recharge, 5 – surface runoff, 6 – run-on infiltration, 7 – interflow, 8 – air moisture transport, 9 – groundwater flow, 10 – groundwater-borne evapotranspiration and air moisture transport).
These buffering fluxes, vital for reducing local climatic extremes (Ripl, 2003), have been substantially reduced. This reduction may stem from limited awareness of their existence and of the features that enhance and restrict them. The creation of field and property boundaries may restrict fluxes between neighbouring lands. For example, roadside ditches form a dense drainage network today. While the total stream length in Bavaria is 100 000 km (1.42 km km−2; LfU, 2024), the length of public roads is 141 800 km (2.01 km km−2; ByStMWBV, 2018), and the length of farm roads is 200 000 km (2.83 km km−2; Anonym, 2018). Due to construction regulations (FGSV, 2021), roads are usually accompanied by roadside ditches on one or both sides. Thus, the drainage network created by roadside ditches is 3 to 6 times as long as the natural drainage network. It collects runoff over short distances, preventing it from entering neighbouring fields with available infiltration capacity. Run-on infiltration, a valuable process in heterogeneous landscapes, is often hindered (Fiener et al., 2011), and groundwater recharge is reduced as runoff is now often directly and effectively transferred to river courses (Fig. 5), meaning there is limited scope for the retention of sediment and other pollutants.
Furthermore, runoff is accelerated and conveyed downstream alongside the road to the next settlement, increasing the risk of flooding. The flow velocity in ditches can be up to 20 times greater than in shallow runoff across fields and grasslands (the typical flow velocity in fields is ∼0.1 m s−1, while roadside ditches may reach 2 m s−1; Seibert and Auerswald, 2020). Peak runoff directly correlates with flow velocity, exacerbating flood risks (Gericke and Smithers, 2014). The homogenization of landscapes further amplifies the issue. Thus, flooding the next village becomes more likely (Bronstert et al., 2018). The fact that surface runoff is generated, which cannot be avoided entirely, even in natural systems, is not the main problem. The problem was amplified by creating an efficient runoff drainage system through ditches and pipes and by homogenizing landscapes, thereby amplifying peak runoff even at a constant runoff volume.
In addition to surface runoff, subsurface runoff is also conveyed by drainage systems. Even in the driest regions of Bavaria, comprehensive subsurface drainage via tiles was carried out with government support, which is well documented in some regions (Fig. 6). It is challenging to envision significant groundwater recharge occurring under such extensive tile drainage systems. Drainage will not only affect the drained areas; it can also cause higher water losses due to evapotranspiration driven by advection on the neighbouring undrained areas (Baldocchi et al., 2016; Klaassen and Claussen, 1995). According to Tetzlaff et al. (2010), 23 % of the agricultural land in Germany has been artificially drained, and drainage runoff in the southern part of Bavaria can be up to 500 mm yr−1 (Wolters et al., 2023). In consequence, after subtracting the water lost by artificial drainage, the remaining precipitation in the south, which has the highest rainfall in Bavaria, is as low as the total precipitation in northern Bavaria, where the rainfall is lowest.
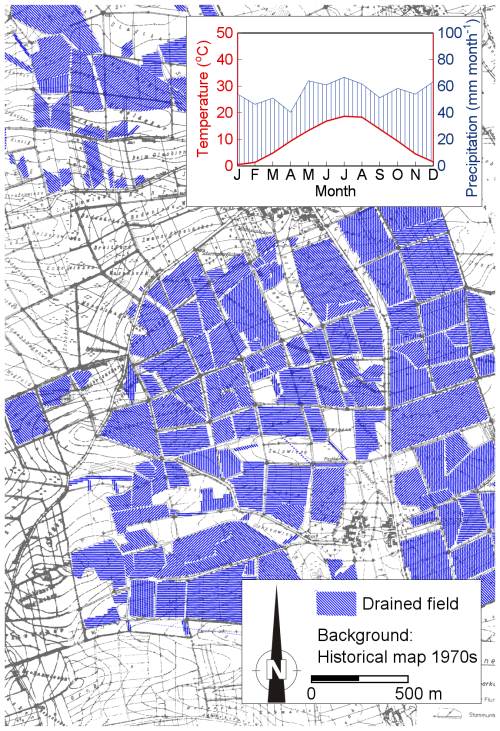
Figure 6Extensive application of tile drainage (blue-shaded fields) can be found even in landscapes with rainfall far below the Bavarian average. The inset is a Walter–Lieth climate diagram for the meteorological normal period from 1991 to 2020 (mean annual precipitation of 670 mm yr−1). The background shows the original plan from the 1970s for the water association of Oberscheckenbach for the village of Gumpenhofen in the county of Rothenburg ob der Tauber, Middle Franconia, on which tile lines were redrawn in blue.
Artificial drainage is not limited to agricultural land; forests have also been drained. These projects, some dating back over a century, are often forgotten, yet the drains remain effective (Tempel, 2006). In recent decades, the extensive network of truckable forest roads has initiated an unintentional forest-wide drainage system. Running perpendicular to the main slope, these roads cut deeply into slopes. As forest soils are prone to high subsurface runoff due to their high infiltration capacity, but contrasting hydraulic conductivities between different horizons (Hümann, 2012; Nordmann, 2011), exfiltration at road cuts can occur. This exfiltrated runoff is then drained via road ditches and bypasses the area downslope of the road (Wigmosta et al., 2002). The mean density of truckable forest roads in Germany is 4.5 km km−2 (BMEL, 2021), restricting surface runoff to short distances (on average 220 m, but this is shorter in steep terrain).
Another cause of additional drainage is the lowering of the groundwater tables. This made drainage by tiles and ditches possible in some areas, but it also led to a decoupling of the rivers and their riparian areas (Auerswald et al., 2019d; Fig. 4) and caused many small rivers to disappear (Reckendorfer et al., 2013; Zerbe, 2023), while the remaining areas suffer increasingly from drought and heatwaves. This poses a major threat to biodiversity (Drainas et al., 2023; Cushway et al., 2024). Riparian areas connected to the groundwater serve as sources of air moisture even in prolonged drought periods, while, after groundwater lowering, coarse-textured riparian areas, associated with river gravel deposits, dry out first and then may act as the nucleus of an expanding drought. This effect of dry riparian areas is accelerated when river courses are straightened, leading to increasing transport capacity and, hence, initiating river incision.
3.3 Soil compaction
The weight of agricultural and forestry machinery has steadily increased since World War II. This upward trend continues unabated. For instance, the wheel load of grain harvesters available in a specific year increased linearly from 2 to 7 t between 1960 and 2000, with no discernible slowing of the trend (Keller et al., 2019). While this increase in weight was offset at the soil surface by expanding the tire contact area, maintaining nearly constant contact area pressure, subsoil compaction increased (Keller and Or, 2022). Subsoil compaction is primarily determined by wheel load, becoming largely unavoidable if the wheel load exceeds 3 to 5 t (Soane et al., 1980; Håkansson and Reeder, 1994; Schjønning et al., 2012). Since the 1990s, such wheel loads have been common in all major agricultural machinery (Keller and Or, 2022). In a recent (2011–2018) German-wide survey along an 8 km × 8 km grid analysing 16 778 soil samples, 51 % of the arable land had root restrictions caused by compaction (Schneider and Don, 2019). This value is similar to the 43 % of over-compacted soils found in the Netherlands (Brus and van den Akker, 2018).
Remarkably, the rapid increase in agricultural yields observed between 1880 and 1990, culminating in a 5-fold increase (Mahlerwein, 2016), with the rise being fastest between 1960 and 1990, suddenly stopped in 1990, when the wheel loads of major agricultural machinery exceeded 5 t. Despite significant advancements in plant breeding (Guarin et al., 2022), no further yield increases were achieved for 3 decades. This is also observed also in other countries that had similar developments in agricultural machinery weights (Keller et al., 2019).
The stagnation in crop yields can be attributed, at least in part, to the slowdown in root growth, even under slight subsoil compaction (Bengough et al., 2011). Upon tillering, gramineous crops produce nodal roots, starting close to the soil surface; similarly, legumes produce basal roots (Tajima, 2021). These shoot-borne roots dominate water uptake (Krassovsky, 1926). Based on measured bulk densities, root growth models indicate that it took roots 2 to 3 weeks to reach a depth of 50 cm in the 1960s. Today, it takes over 2 months to cover the same depth (Keller et al., 2019). Consequently, restricted subsoil exploration forces plants to extract all their water needs from the topsoil. This situation mimics meteorological drought conditions because water depletion of the topsoil increases temperatures and reduces air humidity, although it is of physiological origin. This likely results in a misleading interpretation of the origin of the drought.
However, compaction might not only result in a higher drought risk but may also impede water percolation and increase the susceptibility to waterlogging (Hartmann et al., 2012). The unforeseen extreme wheat failure in France in 2016, which even exceeded the yield loss experienced during the centennial drought in 2003, was mainly caused by anoxia during a cool and wet May. Such conditions are expected to become more frequent due to CO2-driven climate change (Ben-Ari et al., 2018; Nóia Júnior et al., 2023). Impeded percolation by subsoil compaction also causes saturation-excess surface runoff and soil loss when the soil above the compacted layer becomes saturated (Verbist et al., 2007).
3.4 Hedgerows
Hedgerows, which owe their existence to agriculture, have largely been lost in Bavaria, like elsewhere in western Europe (Forman and Baudry, 1984; Meeus, 1993). The positive impact of hedgerows on agricultural yields has long been recognized (e.g. Wendt, 1951) and is supported by numerous studies (Sudmeyer et al., 2007; Veste et al., 2020). The primary mechanism involves reducing wind velocity close to the soil surface, which decreases evapotranspiration. Calculations specific to eastern Germany suggest that evapotranspiration can be reduced by nearly 100 mm yr−1 over a distance equivalent to 25 times the height of the hedge (Funk et al., 2022). This compensation effectively counteracts increasing fluctuations in precipitation due to CO2 effects on climate.
Furthermore, hedgerows influence the diurnal variation in air temperature (Forman and Baudry, 1984). Nights become cooler, reducing plant respiration (Ryan, 1991) and promoting dew formation (Monteith, 1957). Conversely, daytime temperatures rise, enhancing quantum yield efficiency and assimilate gain (Ehleringer and Bjorkman, 1977). Despite these benefits, many hedgerows have been removed. This may be attributed to a misinterpretation of yield gains, which are maximized at a distance roughly 5 times the hedge height. The diminishing yield as distance to the hedge decreases can be misconstrued as yield loss caused by the hedge, while it actually represents diminishing yield gains.
Land-use impacts, such as wheel tracks or soil sealing, occur on a much smaller spatial scale than meteorological phenomena like rain cells or atmospheric pressure systems. However, land-use practices also affect vast areas due to the widespread standardization of methods, regional specialization, and the increasing size of agricultural fields. Wheel tracks, although narrow compared to meteorological impacts, typically cover entire fields. Analysis indicates that fields experience 10 to 20 wheel passes per year, leaving almost no gaps between wheeled areas. Less than 10 % of the field area is wheeled less frequently, and around 10 % is wheeled more often (Augustin et al., 2020). Given that wheeling patterns vary with crop type and the persistence of subsoil compaction, along with the fact that the load is supported by an increasing area with increasing depth, a largely uniform and comprehensive coverage by wheel tracks can be assumed for a crop rotation. Furthermore, similar agricultural machinery is employed across industrialized nations. The increasing weight of machinery is not confined to specific crops or production systems but affects cropland, grassland (Horn et al., 2020), and forested areas (Cambi et al., 2015), impacting both conventional and organic farms. This trend is driven by market forces that influence all forms of production, leading manufacturers to scale up the size of all types of machinery (Keller et al., 2019). The differences are, thus, only gradual or due to the use of older machinery. Given that these mechanisms have been in operation for decades and that critical wheel loads exceeding 5 t have been surpassed since the 1990s, it is reasonable to assume that widespread exposure to high wheel loads has led to extensive subsoil compaction.
A similar argument applies to other localized impacts like soil sealing. The focus in the scientific literature on the urban-heat-island effect can create a misleading impression, stemming from an anthropocentric perspective and the concentration of humans in urban areas. From a hydrological perspective, soil sealing is more problematic in rural areas, where more roads and infrastructure are required to support a smaller population density and where houses have fewer floors than in cities. As a result, the sealed areas per inhabitant are up to 40 times larger in rural counties than in metropolitan counties even without consideration of farm and forest roads (Üreyen and Thiel, 2017). Thus, land-use impacts like subsoil compaction or sealing can affect areas comparable to or even larger than atmospheric pressure systems.
A fundamental assumption in field-scale and farm-scale experiments is that farmers have the capacity to implement improved land management practices. However, this perspective requires reconsideration in light of global market mechanisms and the large-scale and overarching changes, such as the increasing weight of agricultural and forestry machinery. Even the most conscientious farmer will be unable to buy light machinery if it is unavailable in the market (Keller et al., 2019). Furthermore, farmers may often remain unaware of these limitations due to a lack of unbiased, industry-independent guidance (Schnyder et al., 2019). As the scale of these impacts grows so does the need for expanded institutional accountability.
Rain cells or pressure systems move over a location within hours or days, subsoil compaction can persist for years or even centuries, tile drainage can remain functional for many decades or longer, and soil sealing is rarely reversed. Hence, on a temporal scale, these influences of land use last considerably longer than meteorological phenomena.
Furthermore, the pace of land-use change is far more rapid than changes in atmospheric CO2 concentration. While it has taken nearly 3 centuries to increase pre-industrial CO2 levels by 50 % (https://climate.nasa.gov/vital-signs/carbon-dioxide, last access: 12 April 2025), the weight of grain harvesters doubled in just 1 decade between 1960 and 1970 (Keller et al., 2019). As a result, we have had much more time to anticipate and understand the effects of CO2, while the large-scale effects of rapid land-use change remain poorly understood. Current research tends to focus on the short-term, localized impacts of land-use changes, such as the effects of soil compaction on crop yield or water loss in individual fields. However, the broader, synchronized changes in land use across regions and continents, which affect much larger areas than individual fields, are only beginning to be explored.
Land use intervenes in multiple ways with the water and energy budget. This should allow us, at least in a temperate climate, to cushion the adverse effects of the CO2-driven climate change and to compensate for the adverse effects of land-use changes of the past.
Sealing is the most significant intervention in soil functioning. This does not only refer to cities where the urban-heat-island effect directly affects humans. Cities are responsible for only a small yet concentrated share of the total sealed surface. The five largest cities in Bavaria contribute only 10 % to the total sealed surface (Esch et al., 2007), illustrating the importance of sealed surfaces for peri-urban and rural areas. Action against sealing is urgently required. Possibilities are manifold. They include unsealing paved surfaces (e.g. parking lots), installing photovoltaics above sealed surfaces to remove some of the solar energy as electricity instead of converting it into latent and sensible heat, and greening (green roofs, tree alleys). Since technical measures usually replace only one soil function at a time, combining measures may be necessary (simultaneous unsealing, greening, and photovoltaic on parking lots). In addition to sponge cities, sponge towns, sponge villages, and sponge landscapes are required. As the effect of a sealed surface on the water and energy budget is universal, the remedies will also have to be universal. Hence, the responsibility rests on everybody, not only on a few city planners.
The landscape requires more hedges or structures similar to hedges. Again, many measures are available, such as solar fences, agroforestry, or tree alleys, all of which can reduce wind velocity. In particular, heavy-traffic roads, which cannot be unsealed, should be accompanied by hedges or tree rows to mitigate their climate-adverse effects. This insight was already gained by Napoleon Bonaparte 200 years ago, who let trees be planted along roads to improve the microclimate for his marching soldiers (Balmer, 2022).
In agriculture, two requirements are most important: lowering wheel loads and improving soil cover by means of living or dead plant material. These will directly affect soil functioning with regard to water and energy balances and increase C storage in soil and/or contribute to CO2 sequestration. Both requirements can be met with many economically advantageous solutions (e.g. Auerswald et al., 2000). The most important obstacle to their adaptation appears to be the lack of industry-independent advice to farmers (Schnyder et al., 2019).
Irrigation appears not to be an eligible remedy as it may reduce water shortages in the irrigated field but could not solve anoxia or runoff problems; instead, it increases their likelihood. The damage is usually greater than the benefit. Root zone storage capacity decreases with irrigation (van Oorschot et al., 2024), elevating water demand, and water storage capacity may decrease due to irrigation-induced erosion (Batista et al., 2023). Irrigation will always increase water consumption and, in turn, water scarcity. The only exception is irrigation using water that cannot be infiltrated, e.g. from sealed surfaces, and that is stored and used in dry periods (example: https://www.vin-aqua.de/, last access: 12 April 2025).
A climate-friendly land use is possible. However, changes are required in so many places that governmental measures like laws or subsidies can never achieve this. Instead, a paradigm change is necessary. The old food security paradigm is subordinate now because it can only be accomplished with climate resilience. The old paradigm of economic efficiency is outdated because efficiency and resilience are mutually exclusive.
Undoubtedly, measures against climate change through the reduction of CO2 emissions are essential and have become a global-policy target. However, exclusively focusing on this goal ignores other important mitigation measures that urgently need to be realized. As illustrated here, restoring hydrologically functional landscapes and soils should be considered to be equally important to mitigate climate change, especially concerning extremes such as floods, droughts, and heatwaves, and to preserve the foundation of food and life. The question of whether land use or CO2 is a more significant driver of floods and drought deserves more attention even though a simple answer will never be possible.
Measures of reducing soil sealing and compaction and retaining water in structurally rich landscapes (sponge landscapes and sponge cities) can all have pronounced climatic effects. Since they also comprise quick and simple measures (e.g. not incorporating straw after the grain harvest), which deliver a measurable cooling of a few kelvin, they offer a greater level of acceptance by the public compared to measures requiring personal lifestyle changes. Policymakers and planners should, therefore, emphasize soil functioning and water retention in climate change policy action.
The most significant challenge may rest on hydrological science, where we largely neglect lateral interactions happening in the atmosphere, on soil surfaces, and in soils. We poorly address lateral phenomena like advection in the atmosphere, run-on infiltration, or subsurface flows. Physical experiments designed to analyse the influence of lateral interactions at the landscape scale are almost impossible to conduct as extensive areas would have to be included, manipulated, and replicated to fulfil statistical requirements. Hence, we rely on modelling. However, it is also the case that our models often disregard these lateral effects. Field size and neighbourhood hardly play a role in many model calculations like evaporation. Land use is typically considered in broad categories like forestland, grassland, cropland, and urban land. We use parameter values derived decades ago, which hardly reflect the unprecedented changes that have occurred within each land-use category during the last decades. For instance, no hydrological model requires agricultural machinery weight and can reflect its effects. Soil properties are treated as constants in most hydrological models, although we know from a multitude of experiments that land use modifies them. Meteorological parameters, such as temperature, humidity, wind, and rainfall, are employed as external controls even though they are influenced by the system, thereby engendering feedback mechanisms. We use models that consider a strongly limited number of controls to find, through circular reasoning, that these controls are important. Moreover, most models use similar or even identical controls instead of complementing each other.
Even the presently strongest CO2-driven climate change effect in rural areas apart from the temperature increase, the pronounced increase in rain erosivity, which causes soil crusting and infiltration-excess runoff, is hardly captured by hydrological models. Most of them only implement percolation-excess runoff (e.g. via the Green–Ampt approach) but do not consider the drivers of crusting.
Comprehensively considering all effects in modelling is hindered by data limitations, computational time constraints, and the unfavourable behaviour of models that consider feedback mechanisms such as increasing complexity, instability, and exponential growth or oscillations. Consequently, it is crucial to acknowledge our limited understanding of land-use effects. Any conclusions regarding the impact of land use based on modelling must be drawn cautiously, regardless of the apparent certainty of modelling results. In turn, considering meteorological changes only in the light of greenhouse gases is biased by the same limitations.
The data used for the figures in this paper can be found at https://doi.org/10.17635/lancaster/researchdata/718 (Auerswald et al., 2025).
The supplement related to this article is available online at https://doi.org/10.5194/hess-29-2185-2025-supplement.
KA led the conceptualization of the paper, led the writing, and drafted the figures. PF contributed to the figures and to the writing of the paper. JG and JQ contributed to the writing of the paper.
The contact author has declared that none of the authors has any competing interests.
Publisher’s note: Copernicus Publications remains neutral with regard to jurisdictional claims made in the text, published maps, institutional affiliations, or any other geographical representation in this paper. While Copernicus Publications makes every effort to include appropriate place names, the final responsibility lies with the authors.
John N. Quinton received support from the Natural Environment Research Council (grant award no. NERC NE/R004722/1).
This paper was edited by Thom Bogaard and reviewed by Adriaan J. (Ryan) Teuling and one anonymous referee.
Allen, R. G., Pereira, L. S., Raes, D., and Smith, M.: Crop evapotranspiration – Guidelines for computing crop water requirements, FAO Irrigation and Drainage Paper, 56, FAO, Rome, 300(9), D05109, https://www.fao.org/4/x0490e/x0490e00.htm (last access: 12 April 2025), 1998.
Anonym: Ländliches Wegenetz [Rural road network], Schriftenreihe der Arge Landentwicklung, 26, 108–109, https://www.landentwicklung.de/fileadmin/sites/Landentwicklung/Dateien/Publikationen/Schriftenreihe_ArgeLandentwicklung/Heft_26_40_Jahre_Bund-Laender-Arbeitsgemeinschaft_Nachhaltige_Landentwicklung.pdf (last access: 12 April 2025), 2018.
Arnault, J., Fersch, B., Rummler, T., Zhang Z., Quenum G.M., Wei J., Graf M., Laux P., and Kunstmann, H.: Lateral terrestrial water flow contribution to summer precipitation at continental scale – A comparison between Europe and West Africa with WRF-Hydro-tag ensembles, Hydrol. Process., 35, e14183, https://doi.org/10.1002/hyp.14183, 2021.
Auerswald, K., Fischer, F. K., Winterrath, T., and Brandhuber, R.: Rain erosivity map for Germany derived from contiguous radar rain data, Hydrol. Earth Syst. Sci., 23, 1819–1832, https://doi.org/10.5194/hess-23-1819-2019, 2019a.
Auerswald, K., Fischer, F., Winterrath, T., Elhaus, D., Maier, H., and Brandhuber, R.: Klimabedingte Veränderung der Regenerosivität seit 1960 und Konsequenzen für Bodenabtragsschätzungen [Climate-change-induced changes in rain erosivity and consequences of soil loss estimation], in: Bodenschutz, Ergänzbares Handbuch der Maßnahmen und Empfehlungen für Schutz, Pflege und Sanierung von Böden, Landschaft und Grundwasser (Loseblattsammlung), edited by: Bachmann, G., König, W., and Utermann, J., Berlin, Erich Schmidt Verlag, 4090, 21 pp., ISBN 978-3-503-02718-7, 2019b.
Auerswald, K., Fischer, F., and Winterrath, T.: R-Faktor – Regenerosivität, in: Pilotstudie “Klimawirkungskarten Bayern” [Pilot study “Climate-effect maps for Bavaria”], UmweltSpezial, Bayerisches Landesamt für Umwelt, Augsburg, 61–69, https://www.bestellen.bayern.de/application/applstarter?APPL=eshop&DIR=eshop&ACTIONxSETVAL(artdtl.htm,APGxNODENR:1325,AARTxNR:lfu_klima_00168,AARTxNODENR:356404,USERxBODYURL:artdtl.htm,KATALOG:StMUG,AKATxNAME:StMUG,ALLE:x)=X, (last access: 12 April 2025), 2019c.
Auerswald, K., Moyle, P., Seibert, S. P., and Geist, J.: HESS Opinions: Socio-economic and ecological trade-offs of flood management – benefits of a transdisciplinary approach, Hydrol. Earth Syst. Sci., 23, 1035–1044, https://doi.org/10.5194/hess-23-1035-2019, 2019d.
Auerswald, K., Albrecht, H., Kainz, M., and Pfadenhauer, J.: Principles of sustainable landuse systems developed and evaluated by the Munich Research Alliance on Agroecosystems (FAM), Petermann. Geogr. Mitt., 144, 16–25, https://www.researchgate.net/publication/261647600_Principles_of_sustainable_land-use_systems_developed_and_evaluated_by_the_Munich_Research_Alliance_on_Agro-Ecosystems_FAM#fullTextFileContent (last access: 12 April 2025), 2000.
Auerswald, K., Geist, J., Fiener, P., and Quinton, J.: Data behind “Floods and droughts – are land use, soil management, and landscape hydrology more significant drivers than increasing CO2?”, Lancaster University [data set], https://doi.org/10.17635/lancaster/researchdata/718, 2025.
Augustin, K., Kuhwald, M., Brunotte, J., and Duttmann, R.: Wheel load and wheel pass frequency as indicators for soil compaction risk: A four-year analysis of traffic intensity at field scale, Geoscience, 10, 292, https://doi.org/10.3390/geosciences10080292, 2020.
Baldocchi, D., Knox, S., Dronova, I., Verfaillie, J., Oikawa, P., Sturtevant, C., Matthes J. H., and Detto. M.: The impact of expanding flooded land area on the annual evaporation of rice, Agr. Forest Meteorol., 223, 181–193, https://doi.org/10.1016/j.agrformet.2016.04.001, 2016.
Baldocchi, D. D. and Rao, K. S.: Intra-field variability of scalar flux densities across a transition between a desert and an irrigated potato field, Bound.-Lay. Meteorol., 76, 109–136, 1995.
Balmer, G.: Baumreihen mit vielen Funktionen [Tree rows with many functions], https://blog.nationalmuseum.ch/2022/07/alleen/ (last access: 30 July 2023), 2022.
Batista, P., Baptista, V., Wilken, F., Seufferheld, K., Quinton, J. N., and Fiener, P.: First evidence of widespread, severe soil erosion underneath centre-pivot irrigation systems, Sci. Total Environ., 888, 164119, https://doi.org/10.1016/j.scitotenv.2023.164119, 2023.
Baumeister, C., Gudera, T., Hergesell, M., Kampf, J., Kopp, B., Neumann, J., Schwebler, W., and Wingering M.: Entwicklung von Bodenwasserhaushalt und Grundwasserneubildung in Baden-Württemberg, Bayern, Rheinland-Pfalz und Hessen (1951–2015) [Development of soil hydrology and groundwater recharge Baden-Wuerttemberg, Bavaria, Rhineland-Palatinate and Hesse], KLIWA-Berichte, 21, 102 pp., https://www.kliwa.de/_download/KLIWAHeft21.pdf (last access: 8 March 2024), 2017.
Ben-Ari, T., Boé, J., Ciais, P., Lecerf, R., Van der Velde, M., and Makowski, D.: Causes and implications of the unforeseen 2016 extreme yield loss in the breadbasket of France, Nat. Commun., 9, 1627, https://doi.org/10.1038/s41467-018-04087-x, 2018.
Bengough, A. G., McKenzie, B. M., Hallett, P. D., and Valentine, T. A.: Root elongation, water stress, and mechanical impedance: A review of limiting stresses and beneficial root tip traits, J. Ex. Bot., 62, 59–68, 2011.
Blumröder, J. S., May, F., Härdtle, W., and Ibisch, P. L.: Forestry contributed to warming of forest ecosystems in northern Germany during the extreme summers of 2018 and 2019, Ecological Solutions Evidence, 2, e12087, https://doi.org/10.1002/2688-8319.12087, 2021.
Boeing, F., Rakovec, O., Kumar, R., Samaniego, L., Schrön, M., Hildebrandt, A., Rebmann, C., Thober, S., Müller, S., Zacharias, S., Bogena, H., Schneider, K., Kiese, R., Attinger, S., and Marx, A.: High-resolution drought simulations and comparison to soil moisture observations in Germany, Hydrol. Earth Syst. Sci., 26, 5137–5161, https://doi.org/10.5194/hess-26-5137-2022, 2022.
BMEL: Waldbericht der Bundesregierung 2021 [Forest Report of the Federal Government], Bundesministerium für Ernährung und Landwirtschaft, 84 pp., https://www.bmel.de/SharedDocs/Downloads/DE/Broschueren/waldbericht2021.pdf?___blob=publicationFile&v=9 (last access: 30 July 2023), 2021.
Brandhuber, R., Treisch, M., Fischer, F., Kistler, M., Maier, H., and Auerswald K.: Starkregen, Bodenerosion, Sturzfluten – Beobachtungen und Analysen im Mai/Juni 2016 [Heavy rainfall, soil erosion, flash floods – Observations and analyses in May/June 2016], Schriftenreihe der Bayerischen Landesanstalt für Landwirtschaft, 2–2017, 121 pp., https://www.lfl.bayern.de/mam/cms07/publikationen/daten/schriftenreihe/starkregen-bodenerosion_sturzfluten_lfl-schriftenreihe.pdf (last access: 12 April 2025), 2017.
Bronstert, A., Agarwal, A., Boessenkool, B., Crisologo, I., Fischer, M., Heistermann, M., Köhn-Reich, L., López-Tarazón, J.A., Moran, T., Ozturk, U., Reinhardt-Imjela, C., and Wendi, D.: Forensic hydro-meteorological analysis of an extreme flash flood: The 2016-05-29 event in Braunsbach, SW Germany, Sci. Total Environ., 630, 977–991, https://doi.org/10.1016/j.scitotenv.2018.02.241, 2018.
Brus, D. J. and van den Akker, J. J. H.: How serious a problem is subsoil compaction in the Netherlands? A survey based on probability sampling, SOIL, 4, 37–45, https://doi.org/10.5194/soil-4-37-2018, 2018.
Bürger, G., Heistermann, M., and Bronstert, A.: Towards subdaily rainfall disaggregation via Clausius–Clapeyron, J. Hydrometeorol., 15, 1303–1311, https://doi.org/10.1175/JHM-D-13-0161.1, 2014.
ByStMWBV: Roads and Bridges in Bavaria, Bayerisches Staatsministerium für Wohnen, Bau und Verkehr, München, https://www.stmb.bayern.de/assets/stmi/vum/strasse/sub_2018_englisch.pdf (last access: 27 March 2024), 2018.
ByStMUV: Klima-Report Bayern 2021 [Climate Report for Bavaria 2021], Bayerisches Staatsministerium für Umwelt und Verbraucherschutz, München, 196 pp., https://www.bestellen.bayern.de/application/applstarter?APPL=eshop&DIR=eshop&ACTIONxSETVAL(artdtl.htm,APGxNODENR:1325,AARTxNR:stmuv_klima_012,AARTxNODENR:358070,USERxBODYURL:artdtl.htm,KATALOG:StMUG,AKATxNAME:StMUG,ALLE:x)=X (last access: 12 April 2025), 2021.
Calder, K. L.: Eddy diffusion and evaporation in flow over aerodynamically smooth and rough surfaces: A treatment based on laboratory laws of turbulent flow with special reference to conditions in the lower atmosphere, Q. J. Mech. Appl. Math., 2, 153–176, 1949.
Cambi, M., Certini, G., Neri, F., and Marchi, E.: The impact of heavy traffic on forest soils: A review, Forest Ecol. Manag., 338, 124–138, https://doi.org/10.1016/j.foreco.2014.11.022, 2015.
Carroll, R. W. H., Deems, J. S., Niswonger, R., Schumer, R., and Williams, K. H.: The importance of interflow to groundwater recharge in a snowmelt-dominated headwater basin, Geophys. Res. Lett., 46, 5899–5908, https://doi.org/10.1029/2019GL082447, 2019.
Ciais, P., Reichstein, M., Viovy, N., Granier, A., Ogee, J., Allard, V., Aubinet, M., Buchmann, N., Bernhofer, C., Carrara, A., Chevallier, F., De Noblet, N., Friend, A. D., Friedlingstein, P., Grunwald, T., Heinesch, B., Keronen, P., Knohl, A., Krinner, G., Loustau, D., Manca, G., Metteucci, G., Miglietta, F., Ourcival, J. M., Papale, D., Pilegaard, K., Rambal, S., Seufert, G., Soussana, J. F., Sanz, M. J., Schulze, E. D., Vesala, T., and Valentini, R.: Europe-wide reduction in primary productivity caused by the heat and drought in 2003, Nature, 437, 529–533, 2005.
Cushway, K. C., Geist, J., and Schwalb, A. N.: Surviving global change: a review of the impacts of drought and dewatering on freshwater mussels, Biol. Rev., 100, 275–307, https://doi.org/10.1111/brv.13142, 2024.
Davin, E. L., Seneviratne, S. I., Ciais, P., and Wang, T.: Preferential cooling of hot extremes from cropland albedo management, P. Natl. Acad. Sci. USA, 111, 9757–9761, https://doi.org/10.1073/pnas.1317323111, 2014.
Drainas, K., Kaule, L., Mohr, S., Uniyal, B., Wild, R., and Geist, J.: Predicting stream water temperature with artificial neural networks based on open-access data, Hydrol. Process., 37, e14991, https://doi.org/10.1002/hyp.14991, 2023.
Drivas, P. J. and Shair, F. H.: Dispersion of an instantaneous cross-wind line source of tracer released from an urban highway, Atmos. Environ., 8, 475–485, 1974.
DWD: Zeitreihen fuer Gebietsmittel fuer Bundeslaender und Kombinationen von Bundeslaender [Time series of spatial averages of federal states and combinations of federal states], Deutscher Wetterdienst (German Weather Service), https://opendata.dwd.de/climate_environment/CDC/regional_averages_DE/monthly/precipitation/regional_averages_rr_06.txt (last access: 10 February 2025), 2025.
Ehleringer, J. R. and Bjorkman, O.: Quantum yields for CO2 uptake in C3 and C4 plants - dependence on temperature, CO2, and O2 concentration, Plant Physiol., 59, 86–90, 1977.
Esch, T., Schorcht, G., and Thiel, M.: Satellitengestützte Erfassung der Bodenversiegelung in Bayern [Satellite-assisted Recording of Soil Sealing in Bavaria], Bayerisches Landesamt für Umwelt, Augsburg, Germany, 17 pp., https://www.bestellen.bayern.de/application/applstarter?APPL=eshop&DIR=eshop&ACTIONxSETVAL(artdtl.htm,APGxNODENR:193925,AARTxNR:lfu_all_00054,AARTxNODENR:194197,USERxBODYURL:artdtl.htm,KATALOG:StMUG,AKATxNAME:StMUG,ALLE:x)=X (last access: 12 April 2025), 2007.
FGSV: Richtlinien für die Anlage von Straßen, Teil Entwässerung (RAS-Ew) [Recommendations for Road Construction; Part Dainage], Forschungsgesellschaft für Straßen- und Verkehrswesen, Arbeitsgruppe Erd- und Grundbau, FGSV Verlag GmbH, Köln, 96 pp., ISBN 978-3-86446-299-3, 2021.
Fiener, P. and Auerswald, K.: Concept and effects of a multi-purpose grassed waterway, Soil Use Manage., 19, 65–72, https://doi.org/10.1111/j.1475-2743.2003.tb00281.x, 2003.
Fiener, P., Auerswald, K., and Van Oost, K.: Spatio-temporal patterns in land use and management affecting surface runoff response of agricultural catchments – a review, Earth-Sci. Rev., 106, 92–104, https://doi.org/10.1016/j.earscirev.2011.01.004, 2011.
Fiener, P., Neuhaus, P., and Botschek, J.: Long-term trends in rainfall erosivity – analysis of high resolution precipitation time series (1937–2007) from Western Germany, Agr. Forest Meteorol., 171–172, 115–123, 2013.
Forman, R. T. T. and Baudry, J.: Hedgerows and hedgerow networks in landscape ecology, Environ. Manage., 8, 495–510, 1984.
Funk, R., Völker, L., Kestel, F., Veste, M., and Hahn, T.: Der Einfluss von Hecken auf Wind und Mikroklima [Influence of Hedges on Wind and Microclimate], Leibniz-Zentrum für Agrarlandschaftsforschung (ZALF), Müncheberg, Germany, https://doi.org/10.13140/RG.2.2.25302.93769, 2022.
García-García, A., Cuesta-Valero, F.J., Miralles, D.G., Mahecha, M. D., Quaas, J., Reichstein, M., Zscheischler, J., and Peng, J.: Soil heat extremes can outpace air temperature extremes, Nat. Clim. Change, 13, 1237–1241, https://doi.org/10.1038/s41558-023-01812-3, 2023.
Gericke, O. J. and Smithers, J. C.: Review of methods used to estimate catchment response time for the purpose of peak discharge estimation, Hydrolog. Sci. J., 59, 1935–1971, https://doi.org/10.1080/02626667.2013.866712, 2014.
Grossiord, C., Buckley, T. N., Cernusak, L. A., Novick, K. A., Poulter, B., Siegwolf, R. T. W., Sperry, J. S., and McDowell, N. G.: Plant responses to rising vapor pressure deficit, New Phytol., 226, 1550–1566, https://doi.org/10.1111/nph.16485, 2020.
Guarin, J. R., Martre, P., Ewert, F., Webber, H., Dueri, S., Calderini, D., Reynolds, M., Molero, G., Miralles, D., Garcia, G., Slafer, G., Giunta, F., Pequeno, D. N. L., Stella, T., Ahmed, M., Alderman, P. D., Basso, B., Berger, A. G., Bindi, M., Bracho-Mujica, G., Cammarano, D., Chen, Y., Dumont, B., Rezaei, E. E., Fereres, E., Ferrise, R., Gaiser, T., Gao, Y., Garcia-Vila, M., Gayler, S., Hochman, Z., Hoogenboom, G., Hunt, L. A., Kersebaum, K. C., Nendel, C., Olesen, J. E., Palosuo, T., Priesack, E., Pullens, J. W. M., Rodríguez A., Rötter, R. R., Ruiz Ramos, M., Semenov, M. A., Senapati, N., Siebert, S., Srivastava, A. K., Stöckle, C., Supit, I., Tao, F., Thorburn, P., Wang, E., Weber, T. K. D., Xiao, L., Zhang, Z., Zhao, C., Zhao, J., Zhao, Z., Zhu, Y., and Asseng, S.: Evidence for increasing global wheat yield potential, Environ. Res. Lett., 17, 124045, https://doi.org/10.1088/1748-9326/aca77c, 2022.
Haberlandt, U., Shehu, B., Thiele, L., Willems, W., Stockel, H., Deutschländer, T., Junghänel, T., and Ostermöller, J.: Methodische Untersuchungen für eine Neufassung der regionalisierten Starkregenstatistik KOSTRA-DWD [Methodological investigations for updating the regionalised extreme rainfall statistics KOSTRA-DWD], Hydrol. Wasserbewirts., 67, 138–159, https://doi.org/10.5675/HyWa_2023.3_1, 2023.
Haerter, J. O. and Berg, P.: Unexpected rise in extreme precipitation caused by a shift in rain type?, Nat. Geosci., 2, 372–373, 2009.
Hartmann, P., Zink, A., Fleige, H., and Horn, R.: Effect of compaction, tillage and climate change on soil water balance of arable Luvisols in Northwest Germany, Soil Till. Res., 124, 211–218, 2012.
Håkansson, I. and Reeder, R. C.: Subsoil compaction by vehicles with high axle load – Extent, persistence and crop response, Soil Till. Res., 29, 277–304, 1994.
Herbst, M., Roberts, J. M., Rosier, P. T. W., Taylor, M. E., and Gowing, D. J.: Edge effects and forest water use: A field study in a mixed deciduous woodland, Forest Ecol. Manag., 250, 176–186, https://doi.org/10.1016/j.foreco.2007.05.013, 2007.
Horn, R., Mordhorst, A., Fleige, H., Zimmermann, I., Burbaum, B., Filipinski, M., and Cordsen, E.: Soil type and land use effects on tensorial properties of saturated hydraulic conductivity in northern Germany, Eur. J. Soil Sci., 71, 179–189, https://doi.org/10.1111/ejss.12864, 2020.
Hümann, M.: Abflussgeschehen unter Wald – Validierung und Weiterentwicklung eines GIS-basierten Tools zur Erstellung von Abflussprozesskarten auf forstlich genutzten Standorten [Runoff from Forests – Validation and Further Development of a GIS Tool to Produce Runoff-Process Maps for Forested Sites], PhD thesis, Universität Trier, 223 pp., https://ubt.opus.hbz-nrw.de/files/542/Dissertation_HAmann_OPUS.pdf (last access: 12 April 2025), 2012.
Ingraham, N. L. and Mark, A. F.: Isotopic assessment of the hydrologic importance of fog deposition on tall snow tussock grass on southern New Zealand uplands, Austral Ecol., 25, 402–408, https://doi.org/10.1046/j.1442-9993.2000.01052.x, 2000.
Jacobs, A. F. G., Heusinkveld, B. G., Kruit, R. J. W., and Berkowicz, S. M.: Contribution of dew to the water budget of a grassland area in the Netherlands, Water Resour. Res., 42, W03415, https://doi.org/10.1029/2005WR004055, 2006.
Joeres, A., Steeger, G., Huth, K., Donheiser, M., and Wörpel S.: Wo in Deutschland das Grundwasser sinkt [Where the groundwater is declining in Germany], https://correctiv.org/aktuelles/kampf-um-wasser/2022/10/25/klimawandel-grundwasser-in-deutschland-sinkt/ (last access: 12 April 2025), 2022.
Kaseke, K. F., Wang, L., and Seely, M. K.: Nonrainfall water origins and formation mechanisms, Science Advances, 3, e1603131, https://doi.org/10.1126/sciadv.1603131, 2017.
Keller, T. and Or, D.: Farm vehicles approaching weights of sauropods exceed safe mechanical limits for soil functioning, P. Natl. Acad. Sci. USA, 119, e2117699119, https://doi.org/10.1073/pnas.2117699119, 2022.
Keller, T., Sandin, M., Colombi, T., Horn, R., and Or, D.: Historical increase in agricultural machinery weights enhanced soil stress levels and adversely affected soil functioning, Soil Till. Res., 194, 10429, https://doi.org/10.1016/j.still.2019.104293, 2019.
Klaassen, W. and Claussen, M.: Landscape variability and surface flux parameterization in climate models, Agr. Forest Meteorol., 73, 181–188, 1995.
Klaassen, W., Van Breugel, P. B., Moors, E. J., and Nieveen, J. P.: Increased heat fluxes near a forest edge, Theor. Appl. Climatol., 72, 231–243, 2002.
Krassovsky, I.: Physiological activity of the seminal and nodal roots of crop plants, Soil Sci., 21, 307–325, 1926.
Lambert, F. H. and Webb, M. J.: Dependency of global mean precipitation on surface temperature, Geophys. Res. Lett., 35, L16706, https://doi.org/10.1029/2008GL034838, 2008.
Leclerc, M. Y. and Thurtell, G. W.: Footprint prediction of scalar fluxes using a Markovian analysis, Bound.-Lay. Meteorol., 52, 247–258, 1990.
Lenderink, G. and van Meijgaard, E.: Increase in hourly precipitation extremes beyond expectations from temperature changes, Nat. Geosci., 1, 511–514, https://doi.org/10.1038/ngeo262, 2008.
LfU: Fachlicher Hintergrund [Technical Background], Bayerisches Landesamt für Umwelt, https://www.lfu.bayern.de/wasser/gewaesserverzeichnisse/fachlicher_hintergrund/index.htm (last access: 15 March 2024), 2024.
Loriaux, J. M., Lenderink, G., De Roode, S. R., and Siebesma, A. P.: Understanding convective extreme precipitation scaling using observations and an entraining plume model, J. Atmos. Sci., 70, 3641–3655, https://doi.org/10.1175/JAS-D-12-0317.1, 2013.
Mahlerwein, G.: Die Moderne 1880–2010. Grundzüge der Agrargeschichte, Band 3 [Modern Times 1880–2010. Outline of Agri-History, volume 3], 248 pp., Böhlau Verlag, Köln, ISBN 978-3-412-22228-4, 2016.
Makarieva, A. M., Gorshkov, V. G., and Li, B.: Revisiting forest impact on atmospheric water vapor transport and precipitation, Theor. Appl. Climatol., 111, 79–96, https://doi.org/10.1007/s00704-012-0643-9, 2013a.
Makarieva, A. M., Gorshkov, V. G., Sheil, D., Nobre, A. D., and Li, B.-L.: Where do winds come from? A new theory on how water vapor condensation influences atmospheric pressure and dynamics, Atmos. Chem. Phys., 13, 1039–1056, https://doi.org/10.5194/acp-13-1039-2013, 2013b.
Makarieva, A. M., Gorshkov, V. G., Sheil, D., Nobre, A. D., Bunyard, P., and Li, B.: Why does air passage over forest yield more rain? Examining the coupling between rainfall, pressure, and atmospheric moisture content, J. Hydrometeorol., 15, 411–426, https://doi.org/10.1175/JHM-D-12-0190.1, 2014.
Mann, M. E., Rahmstorf, S., Kornhuber, K., Steinman, B. A., Miller, S. K., Petri, S., and Coumou, D.: Projected changes in persistent extreme summer weather events: The role of quasi-resonant amplification, Science Advances, 4, eaat3272, https://doi.org/10.1126/sciadv.aat3272, 2018.
Mayr, B., Thaler, T., and Hübl, J.: Successful small-scale household relocation after a millennial flood event in Simbach, Germany 2016, Water-Switzerland, 12, 156, https://doi.org/10.3390/w12010156, 2020.
McNaughton, K. G.: Evaporation and advection II: evaporation downwind of a boundary separating regions having different surface resistances and available energies, Q. J. Roy. Meteor. Soc., 102, 193–202, 1976.
Meeus, J. H. A.: The transformation of agricultural landscapes in Western Europe, Sci. Total Environ., 129, 171–190, https://doi.org/10.1016/0048-9697(93)90169-7, 1993.
Migała, K., Liebersbach, J., and Sobik, M.: Rime in the Giant Mts. (The Sudetes, Poland), Atmos. Res., 64, 63–73, 2002.
Miralles, D. G., Gentine, P., Seneviratne, S. I., and Teuling, A. J.: Land–atmospheric feedbacks during droughts and heatwaves: state of the science and current challenges, Ann. NY Acad. Sci., 1436, 19–35, https://doi.org/10.1111/nyas.13912, 2019.
Mohr, S., Ehret, U., Kunz, M., Ludwig, P., Caldas-Alvarez, A., Daniell, J. E., Ehmele, F., Feldmann, H., Franca, M. J., Gattke, C., Hundhausen, M., Knippertz, P., Küpfer, K., Mühr, B., Pinto, J. G., Quinting, J., Schäfer, A. M., Scheibel, M., Seidel, F., and Wisotzky, C.: A multi-disciplinary analysis of the exceptional flood event of July 2021 in central Europe – Part 1: Event description and analysis, Nat. Hazards Earth Syst. Sci., 23, 525–551, https://doi.org/10.5194/nhess-23-525-2023, 2023.
Monteith, J. L.: Dew, Q. J. Roy. Meteor. Soc., 83, 322–341, https://doi.org/10.1002/qj.49708335706, 1957.
Nóia Júnior, R. de S., Deswarte, J.-C., Cohan, J.-P., Martre, P., van der Velde, M., Lecerf, R., Webber, H., Ewert, F., Ruane, A.C., Slafer, G.A., and Asseng, S.: The extreme 2016 wheat yield failure in France, Glob. Change Biol., 29, 3130–3146, https://doi.org/10.1111/gcb.16662, 2023.
Nordmann, B.: Einfluss der Forstwirtschaft auf den vorbeugenden Hochwasserschutz – Integrale Klassifizierung abflusssensitiver Waldflächen [Influence of Forestry on Precautionary Flood Protection – Integral Classification of Runoff-Sensitive Forest Areas (in German)], PhD thesis, Technische Universität München, 242 pp., https://mediatum.ub.tum.de/1006969 (last access: 12 April 2025), 2011.
NRCS: Estimation of direct runoff from storm rainfall, in: National Engineering Handbook. Part 630 Hydrology, chapter 10, Natural Resources Conservation Service (NRCS), United States Department of Agriculture, 79 pp., https://irrigationtoolbox.com/NEH/Part630_Hydrology/H_210_630_10.pdf (last access: 12 April 2025), 2004.
Oke, T. R.: The energetic basis of the urban heat island, Q. J. Roy. Meteor. Soc., 108, 1–24, 1982.
Panin, G., Tetzlaff, G., and Raabe, A.: Inhomogeneity of the land surface and problems in the parameterization of surface fluxes in natural conditions, Theor. Appl. Climatol., 60, 163–178, https://doi.org/10.1007/s007040050041, 1998.
Prueger, J. H., Hipps, L. E., and Cooper, D. I.: Evaporation and the development of the local boundary layer over an irrigated surface in an arid region, Agr. Forest Meteorol., 78, 223–237, 1996.
Raatz, L., Bacchi, N., Pirhofer Walzl, K., Glemnitz M., Müller, M. E. H., Joshi, J., and Scherber, C.: How much do we really lose? – Yield losses in the proximity of natural landscape elements in agricultural landscapes, Ecol. Evol., 9, 7838–7848, https://doi.org/10.1002/ece3.5370, 2019.
Reckendorfer, W., Böttiger, M., Funk, A., and Hein, T.: The development of abandoned side-channels: ecological implications and future perspectives, in: 5th Symposium Conference Volume for Research in Protected Areas, 639–642, https://www.researchgate.net/publication/237150599_The_development_of_abandoned_side-channels_ecological_implications_and_future_perspectives/citations (last access: 12 April 2025), 2013.
Ripl, W.: Water: the bloodstream of the biosphere, Philos. T. R. Soc. B, 358, 1921–1934, https://doi.org/10.1098/rstb.2003.1378, 2003.
Robine, J.-M., Cheung, S. L. K, Le Roy, S., Van Oyen, H., Griffiths, C., Michel, J.-P., and Herrmann, F. R.: Death toll exceeded 70,000 in Europe during the summer of 2003, C. R. Biol., 331, 171–178, https://doi.org/10.1016/j.crvi.2007.12.001, 2008.
Roderick, M. L., Sun, F., Lim, W. H., and Farquhar, G. D.: A general framework for understanding the response of the water cycle to global warming over land and ocean, Hydrol. Earth Syst. Sci., 18, 1575–1589, https://doi.org/10.5194/hess-18-1575-2014, 2014.
Ryan, M. G.: Effects of climate change on plant respiration, Ecol. Appl., 1, 157–167, 1991.
Savage, M. J., McInnes, K. J., and Heilman, J. L.: The “footprints” of eddy correlation sensible heat flux density, and other micrometeorological measurements, South African J. Sci., 92, 137–142, 1996.
Schjønning, P., Lamandé, M., Keller, T., Pedersen, J., and Stettler, M.: Rules of thumb for minimizing subsoil compaction, Soil Use Manage., 28, 378–393, 2012.
Schneider, F. and Don, A.: Root-restricting layers in German agricultural soils. Part I: extent and cause, Plant Soil, 442, 433–451, https:doi.org/10.1007/sII104-019-04185-9, 2019.
Schnyder, H., Auerswald, K., Geist, J., and Heissenhuber, A.: Farmers need independent and holistic advice, Nature, 571, 326, https://doi.org/10.1038/d41586-019-02165-8, 2019.
Seibert, S. and Auerswald, K.: Hochwasserminderung im ländlichen Raum – Ein Handbuch zur quantitativen Planung [Flood Mitigation in Rural Areas – a Handbook for Quantitative Planning], Springer Verlag, https://doi.org/10.1007/978-3-662-61033-6, 2020.
Shehu, B., Willems, W., Stockel, H., Thiele, L.-B., and Haberlandt, U.: Regionalisation of rainfall depth–duration–frequency curves with different data types in Germany, Hydrol. Earth Syst. Sci., 27, 1109–1132, https://doi.org/10.5194/hess-27-1109-2023, 2023.
Sharratt, B. S. and Campbell, G. S.: Radiation balance of a soil-straw surface modified by straw color, Agron. J., 86, 200–203, https://doi.org/10.2134/agronj1994.00021962008600010035x, 1994.
Skliris, N., Zika, J., Nurser, G., Josey, S. A., and Marsh, R.: Global water cycle amplifying at less than the Clausius-Clapeyron rate, Sci. Rep.-UK, 6, 38752, https://doi.org/10.1038/srep38752, 2016.
Soane, B. D., Blackwell, P. S., Dickson, J. W., and Painter, D. J.: Compaction by agricultural vehicles: A review II. Compaction under tyres and other running gear, Soil Till. Res., 1, 373–400, https://doi.org/10.1016/0167-1987(80)90039-2, 1980.
Sudmeyer, R., Bicknell, D., and Coles, N.: Tree windbreaks in the Wheatbelt, Bulletin, 4723, Department of Agriculture and Food, Western Australia, 28 pp., https://library.dpird.wa.gov.au/cgi/viewcontent.cgi?article=1196&context=bulletins (last access: 12 April 2025), 2007.
Tajima, R.: Importance of individual root traits to understand crop root system in agronomic and environmental contexts, Breed Sci., 71, 13–19, https://doi.org/10.1270/jsbbs.20095, 2021.
Tempel, M.: Abflussverhalten kleiner, forstlich genutzter Bacheinzugsgebiete am Beispiel des Einzugsgebietes des Oberen Gräfenbaches im Soonwald/Hunsrück [Runoff Behavior of Small, Forested Creek Catchments Using the Example of the Upper Graefenbach in the Soonwald/Hunsrueck], PhD thesis, Johannes Gutenberg-Universität Mainz, https://openscience.ub.uni-mainz.de/bitstreams/4490e295-eff8-4026-9760-e33c4b2835d6/download (last access: 12 April 2025), 2006.
Tetzlaff, B., Kuhr, P., and Wendland, F.: National inventory of artificially drained lands in Germany, CIGR XVIIth World Congress, Québec City, Canada, 13–17 June, 10 pp., https://doi.org/10.13031/2013.32112, 2010.
Uber, M., Haller, M., Brendel, C., Hillebrand, G., and Hoffmann, T.: Past, present and future rainfall erosivity in central Europe based on convection-permitting climate simulations, Hydrol. Earth Syst. Sci., 28, 87–102, https://doi.org/10.5194/hess-28-87-2024, 2024.
Üreyen, S. and Thiel, M.: Satellitengestützte Erfassung der Bodenversiegelung in Bayern [Satellite-Assisted Recording of Soil Sealing in Bavaria], Bayerisches Landesamt für Umwelt, Augsburg, Germany, 71 pp., https://www.lfu.bayern.de/umweltkommunal/flaechenmanagement/bodenversiegelung/index.htm (last access: 12 April 2025), 2017.
van der Ent, R. J. and Tuinenburg, O. A.: The residence time of water in the atmosphere revisited, Hydrol. Earth Syst. Sci., 21, 779–790, https://doi.org/10.5194/hess-21-779-2017, 2017.
van der Ent, R. J., Savenije, H. H. G., Schaefli, B., and Steele-Dunne, S. C.: Origin and fate of atmospheric moisture over continents, Water Resour. Res., 46, W09525, https://doi.org/10.1029/2010WR009127, 2010.
van der Ploeg, R. R., Ehlers, W., and Sieker, F.: Floods and other possible adverse environmental effects of meadowland area decline in former West Germany, Naturwissenschaften, 86, 313–319, https://doi.org/10.1007/s001140050623, 1999.
van der Ploeg R. R., Hermsmeyer D., and Bachmann J.: Post-war changes in land use in former West Germany and the increased number of inland floods, in: Flood Issues in Contemporary Water Management, edited by: Marsalek, J., Watt, W. E., Zeman, E., and Sieker, F., Kluwer Academic Publishers, Dordrecht, ISBN 978-0-7923-6452-8, 2000.
van Oorschot, F., van der Ent, R. J., Alessandri, A., and Hrachowitz, M.: Influence of irrigation on root zone storage capacity estimation, Hydrol. Earth Syst. Sci., 28, 2313–2328, https://doi.org/10.5194/hess-28-2313-2024, 2024.
van Vuuren, D. P., Edmonds, J., Kainuma, M., Riahi, K., Thomson, A., Hibbard, K., Hurtt, G. C., Kram, T., Krey, V., Lamarque, J.-F., Masui, T., Meinshausen, M., Nakicenovic, N., Smith, S. J., and Rose, S. K.: The representative concentration pathways: an overview, Climatic Change, 109, 5–31, https://doi.org/10.1007/s10584-011-0148-z, 2011.
Verbist, K., Cornelis, W. M., Schiettecatte, W., Oltenfreiter, G., Van Meirvenne, M., and Gabriels, D.: The influence of a compacted plow sole on saturation excess runoff, Soil Till. Res., 96, 292–302, https://doi.org/10.1016/j.still.2007.07.002, 2007.
Veste, M., Littmann, T., Kunneke, A., du Toit, B., and Seifert, T.: Windbreaks as part of climate-smart landscapes reduce evapotranspiration in vineyards, Western Cape Province, South Africa, Plant Soil Environ., 66, 119–127, https://doi.org/10.17221/616/2019-PSE, 2020.
Wendt, H.: Der Einfluß der Hecken auf den landwirtschaftlichen Ertrag [The influence of hedgerows on the agricultural yield], Erdkunde 5, 115–125, 1951.
Westra, S., Fowler, H. J., Evans, J. P., Alexander, L. V., Berg, P., Johnson, F., Kendon, E. J., Lenderink, G., and Roberts, N. M.: Future changes to the intensity and frequency of short-duration extreme rainfall, Rev. Geophys., 52, 522–555, https://doi.org/10.1002/2014RG000464, 2014.
Wigmosta, M. S., Nijssen, B., and Storck, P.: The distributed hydrology soil vegetation model, in: Mathematical Models of Small Watershed Hydrology and Applications 1, edited by: Singh, V. P. and Frevert, D. K., Water Resouce Publications, Littleton, 7–42, https://www.pnnl.gov/sites/default/files/media/file/The-distributed-hydrology-soil-vegetation-model.pdf (last access: 12 April 2025), 2002.
Willems, W., Stockel, H., Haberlandt, U., Shehu, B., Junghänel, T., Ostermöller, J., and Deutschländer T.: Betrachtungen zur Instationarität extremer Niederschläge in Deutschland [Reflection on the instationarity of extreme rainfalls in Germany], Hydrol. Wasserbewirts., 67, 151–159, 2023.
Winterrath, T.: Jährlicher (2001–2019) R-Faktor [N/h/yr] auf Basis der stündlichen Niederschlagszeitreihen der RADKLIM-Version 2017.002 [R Factor [N/h/yr] Based on Hourly Rainfall Series of RADKLIM, Version 2017.002], https://opendata.dwd.de/climate_environment/CDC/grids_germany/annual/erosivity/precip_radklim/2017_002/ (last access: 15 May 2023), 2023.
Wischmeier, W. H.: A rainfall erosion index for a universal soil-loss equation, Soil Sci. Soc. Am. Pro., 23, 246–249, 1959.
Wischmeier, W. H. and Smith, D. D.: Rainfall energy and its relationship to soil loss, EOS T. Am. Geophys. Un., 39, 285–291, 1958.
Wolters, T., McNamara, I., Tetzlaff, B., and Wendland, F.: Germany-wide high-resolution water balance modelling to characterise runoff components as input pathways for the analysis of nutrient fluxes, Water-Switzerland, 15, 3468, https://doi.org/10.3390/w15193468, 2023.
Woodward, D. E., Hawkins, R. H., and Quan, Q. D.: Curve number method: Origins, applications and limitations, in: Hydrologic Modeling for the 21st Century: 2nd Federal Interagency Hydrologic Modeling Conf., Las Vegas, NV, https://www.studypool.com/documents/7310093/curve-number-method-origins-applications-and-limitations (last access: 12 April 2025), 2002.
Woolhiser, D. A., Smith, R. E., and Giraldez, J. V.: Effects of spatial variability of saturated hydraulic conductivity on Hortonian overland flow, Water Resour. Res., 32, 671–678, 1996.
Yan, H., Wu, F., and Dong, L.: Influence of a large urban park on the local urban thermal environment, Sci. Total Environ., 622–623, 882–891, 2018.
Zerbe, S.: Rivers and floodplains, in: Restoration of Ecosystems – Bridging Nature and Humans, Springer, https://doi.org/10.1007/978-3-662-65658-7, 2023.
Zimmermann, L. and Zimmermann, F.: Fog deposition to Norway spruce stands at high elevations sites in the eastern Erzgebirge (Germany), J. Hydrol., 256, 166–175, 2002.
Zipper, S. C., Schatz, J., Kucharik, C. J., and Loheide, S. P.: Urban heat island-induced increases in evapotranspirative demand, Geophys. Res. Lett., 44, 873–881, 2017.