the Creative Commons Attribution 4.0 License.
the Creative Commons Attribution 4.0 License.
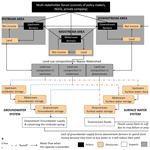
The H2Ours game to explore water use, resources and sustainability: connecting issues in two landscapes in Indonesia
Lisa Tanika
Rika Ratna Sari
Arief Lukman Hakim
Meine van Noordwijk
Marielos Peña-Claros
Beria Leimona
Edi Purwanto
Erika N. Speelman
Restoring hydrological functions affected by economic development trajectories faces social and economic challenges. Given that stakeholders often only have a partial understanding of functioning socio-hydrological systems, it is expected that knowledge sharing will help them to become more aware of the consequences of their land use choices and options to manage water collectively. This facilitates the collective learning tools needed to represent the essential social and technical aspects of a socio-hydrological system in simple terms. However, data-driven simplification can lead to very site-specific models that are difficult to adapt to different conditions. To address these issues, this study aims to develop a highly adaptable serious game based on process understanding to make it easily applicable to any situation and to facilitate co-learning among stakeholders regarding complex socio-hydrological problems. We developed and tested a serious game that revolves around a simple water balance and economic accounting, with environmental and financial consequences for land users. The game is based on process understanding of the system, allowing for both relevant site specificity and generic replicability. Here, we describe the development of the Water: Use, Resources and Sustainability (H2Ours) game and explore its capacity to visualise, discuss and explore issues at the landscape level. The H2Ours game was designed using a combination of the Actors, Resources, Dynamics and Interaction (ARDI) and Drivers, Pressure, State, Impact and Responses (DPSIR) frameworks. The design steps for constructing the game led to a generic version and two localised versions for two different landscapes in Indonesia: a mountain slope to lowland paddy landscape impacting groundwater availability in East Java and a peatland with drainage rewetting, oil palm conversion and fire as issues triggering responses in West Kalimantan. Based on an evaluation referring to credibility, salience and legitimacy criteria, the H2Ours game met its purpose as a tool for knowledge transfer, learning and action triggering. We discuss the steps that can lead to re-designing and adaptation of the game to other landscapes and their policy-relevant issues.
- Article
(10667 KB) - Full-text XML
- BibTeX
- EndNote
A recent call for collective action by the Global Commission on the Economics of Water (Mazzucato et al., 2023) asked for a turning of the tide, shifting from exploitation, overuse and waste of freshwater resources to stewardship, wise use and socio-hydrological restoration. To achieve this shift, better understanding is needed of the relations between the social and hydrological systems and how these relations vary over time and space (D'Odorico et al., 2019). For example, many locations experience hydrological problems due to changes in the use of land and water to meet food production and other domestic and industrial needs (Djuwansyah, 2018). These uses often affect negatively the ability of water systems to retain their hydrological functions, which results in an increase in water demand (Rosa et al., 2018), leading ultimately to degradation of the water system. Consequently, hydrological restoration aims to re-establish or restore the hydrological functions and to avoid further hydrological degradation by managing water resources sustainably and/or eliminating the causal factors of degradation (Zhao et al., 2016).
Four interacting knowledge-to-action steps are needed to determine adequate strategies for socio-hydrological restoration (van Noordwijk, 2018). These steps are understanding (technical agenda setting based on the social relevance of environmental issues), commitment to goals (social understanding of urgency), operationalisation of means of implementation based on a common but differentiated responsibility (in a socio-ecological context) and innovation for better solutions (through monitoring and learning). Consequently, the first step for any restoration planning is developing a shared understanding of how the above- and below-ground ecosystem structure and the climate generate the hydrological functions and underpin the range of ecosystem services provided (van Noordwijk et al., 2022). Furthermore, the interactions between ecological and technical aspects and socio-economic conditions in a landscape (e.g. land tenure, the existence of regulations and incentive–disincentive mechanisms) make the socio-hydrological systems even more complex. Unfortunately, the lacking knowledge transfer between and within different groups of stakeholders often blocks the commitment, operationalisation and innovation stages of successful restoration (Creed et al., 2018).
Learning leads to gaining new information, knowledge and predictive ability and ultimately to scenario development and knowledgeable decisions. However, providing information alone is not a catalyst that can trigger the associated knowledge of the action chain (Marini et al., 2018). Therefore, “services” that facilitate active learning and “experiences” that provide a social context for technical aspects are needed for collective learning beyond knowledge transfer. In the “learning” literature, there is a consensus that people learn more quickly through experiential learning where they can actively explore, engage with processes and then reflect on what happened during the exploration (Kolb and Kolb, 2005; Fanning and Gaba, 2007; Kolbe et al., 2015). Thus, we need tools that can show how a socio-hydrological system works as a whole and allow people to see and experience the consequences of decisions made, in order to strengthen knowledge sharing and to facilitate collective decision-making. Two tools are being increasingly used in this context: hydrological modelling (Guo et al., 2021; Tsai et al., 2021) and serious gaming (Rossano et al., 2017; Feng et al., 2018; Ferguson et al., 2020). Hydrological modelling focuses on converting data to information, knowledge and understanding of technical aspects, and it is used to simulate various land use change scenarios and to quantify the likely consequences of various water management practices (Singh and Kumar, 2017). In contrast, serious gaming focuses on relating knowledge and understanding of social and technical aspects to enhance the credibility of decisions made. It adopts the basic elements of gaming, such as challenges, rewards, experiences, strategies and emotions, to allow stakeholders to safely explore management options (Fleming et al., 2014, 2016).
Although one can see all models as games and all games as models, these conceptually related tools have developed as separate communities of practice (van Noordwijk et al., 2020). Games are models as they are succinct and often stylised representations of a more complex reality, and models are games as they allow the exploration of alternative strategies. In addition, both approaches require one to break down a complex system into several pieces, which is challenging as not all elements of the real conditions can or should be included in the models and/or games. Several considerations can serve as a guide to the simplification process from reality to model and game simulation (Medema et al., 2019), such as what knowledge we want to share with participants, what we want them to learn and what changes or responses we expect from them.
Socially interactive games and models that explore larger spatial and temporal horizons have complementary strengths. As reviewed in Villamor et al. (2023), games and models can (1) seek a conceptual triangulation representing the processes behind complex realties, (2) strive for numerical consistency between games and empirical models, (3) use games in the development of scenario models or (4) use models in the design of games that encourage players to learn by experiencing manageable complexity. As an example of the last option, Lohmann et al. (2014) designed and tested model-based roleplay with Namibian land reform beneficiaries, simulating 10 years of rangeland management. In this paper, we explore the feasibility of transforming a hydrological model into a serious game to provide socio-hydrological dynamics to stakeholders with diverse backgrounds to develop restoration plans.
Simplifying the complexity of the system and highlighting the socio-hydrological issues from a hydrological model to a socio-hydrological game will facilitate knowledge transfer between stakeholders and provide a better decision-making tool (Savic et al., 2016). However, such a simplification process can lead to serious games that are very specific to a given local context, making it difficult for the game to be applied to other places. For that reason, the elements and rules of the game should be easily adapted to other locations, or at least there should be guidelines on how the game can be applied elsewhere.
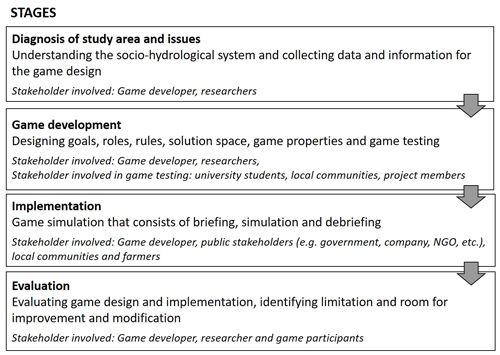
Figure 1Stages undertaken from the preparation to the evaluation of the H2Ours game, including stakeholder involvement across the different stages of this study.
Therefore, the objectives of this study are to develop a serious game that is adaptable to different socio-hydrological contexts and issues and to evaluate the quality of the game in terms of credibility, salience and legitimacy. To achieve our objectives, we developed a generic game with two adaptations to two locations in Indonesia that largely differ in their hydrological characteristics. First, we developed the H2Ours game based on the socio-hydrological characteristics of the Rejoso watershed in East Java. Then, we modified the H2Ours game according to the conditions of the Pawan–Kepulu peatland in West Kalimantan. The qualities of the game were assessed based on the criteria of credibility, salience and legitimacy, which were included in the game development process and the post-game assessment.
We organised the paper by presenting as a method the stages where we prepared, designed, tested, implemented and evaluated the two variants of the H2Ours game. The game itself is the primary “result”, illustrated by the game dynamics during test settings and early applications with local stakeholders. Feedback from game participants is presented as an evaluation of the current games. We end by discussing the simplification process from reality to game, effectiveness of the game in achieving the goals set and the lessons learned.
This study consists of four stages from the diagnosis of the study area to the evaluation of the game (Fig. 1). The different stakeholders involved in each stage are also provided.
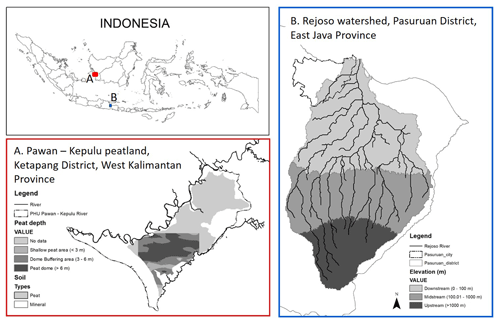
Figure 2The two study areas: (a) the Rejoso watershed that consists of upstream (elevation > 1000 m above sea level; ), midstream (elevation 100–1000 ) and downstream (elevation < 100 ) parts and (b) the Pawan–Kepulu peatland that consists of a peat dome (peat depth > 6 m), a peat-buffering dome (peat depth 3–6 m) and shallow peat (peat depth < 3 m).
2.1 Study areas
The two study areas used in this research, i.e. the Rejoso watershed and the Pawan–Kepulu peatland (Fig. 2), differ in their physical characteristics (hydrological system, land cover, soil type), but they experience similar socio-hydrological problems (lack of coordination and collective action). In the Rejoso watershed, the hydrological restoration was conducted under the Rejoso Kita project in which World Agroforestry (ICRAF) was responsible for research and development of conservation and restoration strategies, while in the Pawan–Kepulu peatland the hydrological restoration was conducted by Tropenbos Indonesia through the Working Landscape project and the Fires project. Both areas have environmental problems because of the disruption of the buffering peak flow that contributes to floods due to a lack of infiltration, which in turn is key to the supply of groundwater. To restore these hydrological functions, understanding of the relationship between land use, surface water or groundwater management and water balance at the landscape level is crucial before developing a joint strategy (IPBES, 2018).
The Rejoso watershed (1600 km2) is in the Pasuruan district, East Java, Indonesia. Based on the elevation and the hydrological system, we can divide the Rejoso watershed into three areas: downstream (< 100 ; metres above sea level), midstream (100–1000 ) and upstream (> 1000 ). This watershed is a national priority because the Umbulan spring is used, through a new pipeline, to supply water to 1.1 million people in the surrounding metropolitan area. Land conversion from agroforestry to intensive agriculture in the recharge areas (> 700 upstream and midstream) and massive groundwater extraction for rice fields using artesian wells in the downstream area were understood to cause the reduced average discharge of the Umbulan spring from 5 m3 s−1 (1980s) to 3.5 m3 s−1 (2020) (Leimona et al., 2018; Amaruzaman et al., 2018; Toulier, 2019; Khasanah et al., 2021). As the declining spring discharge is disrupting the water supply for drinking water, agriculture and industry, stakeholders in the Rejoso watershed need to develop strategies to restore the hydrological function of their watershed through land use management in the recharge area and groundwater utilisation in the downstream area to maintain the continuity of the water supply in the Umbulan spring (Khasanah et al., 2021).
The Pawan–Kepulu peatland is located in the Ketapang district in West Kalimantan. This area is between the Pawan and Kepulu rivers, functioning as a unified hydrological system (Fig. 2a). Based on the mapped peat depth, we divided the Pawan–Kepulu peatland into a relatively shallow peat area (peat depth < 3 m), a buffering dome area (peat depth 3–6 m) and a dome (peat depth > 6 m). In the 2000s, local communities and oil palm companies started to build canals for artificial drainage to facilitate timber extraction and management of oil palm and other forms of agriculture (Carlson et al., 2012). However, during the dry season, the canals cause a decrease in the groundwater level so that the peatland becomes drier and more vulnerable to fire. Land fires are detrimental to both the local area and the global level with haze and carbon emissions (Widayati et al., 2021). Therefore, there is interest in restoring the hydrological function of peatlands to prevent or reduce land fires (Murdiyarso et al., 2021).
2.2 Diagnosis of the study areas and issues
In developing the H2Ours game, the system diagnosis relied on hydrological information (e.g. hydrological boundaries, hydrological problems and efforts that may control the causes and overcome impacts), climate conditions (e.g. rainfall, potential evapotranspiration), land cover information (e.g. typology, the main locally relevant types, recent land cover change, lifecycle profitability estimates) and socio-economic information (e.g. village conditions, socio-economic issues, alternative livelihood options, institutional conditions). These information types were collected using the rapid hydrological appraisal (RHA) approach, which has been used and tested in a number of South-East Asian countries (van Noordwijk et al., 2013; Jeanes et al., 2006). In this approach, the information was grouped based on local ecological knowledge (LEK), public ecological knowledge (PEK) and modeller–scientist ecological knowledge (MEK). Mapping these different knowledge systems showed overlap, gaps and contrasts that provided starting points for further exploration.
To make it easier to describe the interactions between the components of a socio-hydrological system, we structured the socio-hydrological condition of the study area based on the Dynamics, Pressure, State, Impact and Responses (DPSIR) and Actors, Resources, Dynamics and Interaction (ARDI) frameworks. The DPSIR framework is widely used to carry out hydrological assessments because of its comprehensive connections between various components in a socio-hydrological system (Sun et al., 2016; Lu et al., 2022). We used the DPSIR framework to trace the causes of problems (including interactions and relationships between social and hydrological components) and further explore various responses to socio-hydrological problems (Sun et al., 2016). The ARDI framework is widely used in companion modelling approaches to guide system diagnosis as a first step towards designing serious games (Etienne et al., 2011). We used the ARDI framework to identify the main stakeholders involved in water management, the main resources, the main processes that affect changes in resources and the interaction between stakeholders and resources (Villamor et al., 2019).
2.3 Game development
In this step, we transformed the information from the DPSIR and ARDI analyses into components needed in the game design: goals, roles, rules and solution space (Fig. 1).
2.4 Scope and objective
The first stage in designing a serious game is to determine the scope and objective of the game (Silva, 2020; Mitgutsch and Alvarado, 2012). The scope of the game refers to the problem or issues to be addressed. The objective of the game refers to the kinds of knowledge, new insights or impacts expected to be obtained by players after participating in the game. We determined the scope and objective of the game based on the socio-hydrological problem defined in the previous stage (Sect. 2.2).
2.4.1 Roles
According to the ARDI framework (Sect. 2.2), we defined roles based on the main stakeholders involved in water management in each study area. Most of the players were asked to be a villager representing the largest stakeholder group, but others had specific roles as agents trying to influence villager decisions. Related to these roles, we designed goals that players must achieve during each simulation based on discussions and interviews with the relevant stakeholders according to their actual goals. Before the game started, we asked each group to choose a leader to facilitate discussion within the internal team and to represent the group when communicating with other groups.
2.4.2 Rules
According to the ARDI and DPSIR frameworks (Sect. 2.2), we transformed the interaction between actors and resources as the rules of the H2Ours game. To show the dynamics of changes in resources and the impact of human decisions, the game's rules consist of a set of values attached to each decision's type of land use and water infrastructure that describe both the economic and water balance components. The economic component consists of the production costs or capital required to manage a certain land use type and the income derived from that land use. The water balance component consists of surface flow and infiltration of each land use type and water infrastructure. The values used as rules for the economic component referred to research findings by ICRAF and Tropenbos Indonesia (Sect. 2.1). For the water balance component, the Rejoso watershed data were obtained from the hydrological modelling and field measurement (Leimona et al., 2018; Suprayogo et al., 2020), while the Pawan–Kepulu peatland data were based on field measurement (Tanika et al., 2024, in preparation). Several local communities then validated the values through a process of discussion and game testing (Sect. 2.4.5). We simplified the values for each land use type as a ratio between land uses to make the quantification process easier during the simulation process. A simple guideline for developing or modifying rules can be seen in Appendix A.
There are two conditions that are used to mark the positions of the participants regarding their goals in the game, i.e. economic and environmental conditions. We derived the economic conditions based on a simple profit calculation equation where profit is revenue minus all financial expenses (taxes, costs, incidental costs, etc.). The underlying economic analysis applied a lifecycle perspective to the various land use systems, annualising discounted future cost–benefit flows. At the sub-landscape level (e.g. upstream, dome), total profit is the difference between total revenue and total production costs, while the environmental indicators were derived based on a simple water balance model implemented in the Generic River Flow (GenRiver) model (https://www.cifor-icraf.org/knowledge/publication/__34283/, last access: 21 August 2024) (van Noordwijk et al., 2017). Consequently, the relationship between the two conditions allowed us to describe the socio-hydrological system of each study area.
2.4.3 Game solution space analysis
The purpose of the game solution space is to define the envelope of possible outcomes within the rules of the game, considering all possible choices made by players in the game (Speelman et al., 2014). In a random walk, any sequence of steps has equal probability and is blind to where this may lead. The solution space of the H2Ours game was explored based on the average of economic and environmental outcomes obtained with a computer-simulated random generator deciding choices for every step. We mapped the estimated solution space after 3, 10, 30, 100, 300 and 1000 random-walk iterations to obtain a reference for the trajectories observed in a limited number of actual, real-player games. The random-walk conditions were generated in R and then simulated using an Excel spreadsheet representation of the H2Ours game and its economic and environmental performance indicators. The 1000 random-walk data set was used to assess the probability density function of outcomes within the solution space. The economic and environmental performance indicators of actual game implementation refer to a player's land use decisions from four different game sessions in the Rejoso watershed which are calculated using the same Excel spreadsheet.
2.4.4 Game properties
The purpose of game development is to bring a game design into a real form that players can play or touch, such as a game board, various required tokens or other attributes that support the simulation of the game. We developed the game to be close to the perceived reality, so that players can relate their decisions to the consequences of the game session and the impacts that they have experienced or will experience with similar decisions. The game board, the game's land use options and the water simulation miniature are the key elements of recognition for players. Therefore, we adapted these elements to the conditions of each study area.
2.4.5 Game testing
The purpose of game testing is to assess the game's playability and dynamics. We tested the game in two ways: checking all the quantification systems using an Excel spreadsheet and checking the complexity through role-playing testing. In the role-playing testing, we tested the game several times with different participants: members of the project, undergraduate students and non-targeted farmer groups. During the role-playing testing with project members, we checked the suitability and game elements against reality: with the students, we calibrated and validated the rules and feedback system in the game; and then, with the farmer groups, we checked whether the rules of the game were sufficiently clear.
2.5 Game implementation
In this study, we conducted 10 game sessions with a total of 93 people participating, with 5 sessions in each of the study areas. All game sessions for the Rejoso watershed were held in October 2021, while those for the Pawan–Kepulu peatland were held in August 2022. In each study area, the first game session was organised with members of a multi-stakeholder forum consisting of representatives of governments, non-governmental organizations (NGOs), private sectors and universities to get ideas about regulations and programmes that would be offered to farmer communities. Four game sessions were organised with the farmer groups to explore the implementation of the regulations and programmes resulting from the game session with the multi-stakeholder forum.
For each game session, we invited a total of 9–12 representatives of farmer groups from the upstream, midstream and downstream villages of the Rejoso watershed and 12–16 representatives of four villages in the Pawan–Kepulu peatland. In the invitation, we let the group determine who would attend the simulation, provided that the group representatives were willing to hold discussions and exchange information with participants from other villages. For the four sessions with farmer groups, we grouped participants according to different criteria to get a variety of decisions. For the Rejoso watershed, we conducted two sessions with participants who had experience with the recent Payment for Ecosystem Services (PES) programme (Leimona et al., 2018) and two sessions with participants from neighbouring villages where the PES programme was not active. For the Pawan–Kepulu peatland, we conducted a game session with members of the village forest management unit, a session with members of an active farmer field school and two sessions with people who are not members of the village forest management unit or the farmer field school. Game sessions took place in a central location in each of the landscapes to allow easy access for all the participants. During the game session, the participants were asked to play the game in the role of a farmer in their location in the landscape.
Each game session required half a day of implementation (briefing, simulation and debriefing), excluding game preparation and participant surveys for further research. We started the session with a briefing of around 10–15 min to help participants connect with the game by introducing the environment, setting goals and clarifying the roles and rules of the game (Rudolph et al., 2014). After completing the game, we did a debriefing of around 30–40 min to allow participants to reflect on what they experienced and learned during the game (Crookall, 2023; Kim and Yoo, 2020). To maintain consistency of H2Ours for different game sessions, we used the game session guideline provided in Appendix B.
The game explores the trade-off space between economic and environmental outcomes, with the responses from players during the debriefing adding further insights. The economic and environmental outcomes were calculated based on the average economic and environmental conditions as a result of decision-making regarding land use combinations during a game simulation over 10 rounds. We present these results together with the results of the solution space analysis to show the positions of player decisions compared to random decision-making. During the debriefing, we asked the participants several questions, e.g. whether they enjoyed the game, what knowledge they gained from the game, how they responded to government regulations of the type included in the game or how they felt seeing other group decisions and their strategies as a member of a multi-stakeholder forum (for a study case, see the Pawan–Kepulu peatland).
2.6 Game evaluation
The aim of the evaluation stage is to assess the game session process and the quality of the game as the bases for the game's performance to fulfil its objectives. The game session process was evaluated based on game performance criteria in the forms of rules that can be understood, fun and playability over time. The quality of the game is assessed based on the scientific logic and reliable knowledge used to build the game (credibility), its relevance to societal issues (salience) and its acceptance by its participants (legitimacy) (Cash et al., 2002; van Voorn et al., 2016). For the effectiveness of the assessment, we followed an input–output assessment process, which evaluated the input used in the game during the development process and the output after the game session (Bedwell et al., 2012). We followed the latter approach and carried out the evaluation based on several criteria that refer to credibility, salience and legitimacy (Table C1 in Appendix C) using some criteria developed by Belcher et al. (2016).
Because Belcher's long list of criteria (Belcher et al., 2016) was originally used to assess the quality of the research, for this study we chose several criteria that were relevant to the game quality. Each of these criteria was measured during the game design process and after the game implementation. We measured these criteria according to how they were associated with the condition and diagnosis of the study area (Sect. 2.1 and 2.2) and the game development process (Sect. 2.3). Please see Table C1 for the parameters and sections associated with each criterion. Rapid evaluations were conducted after the game session to assess the process and quality of the game session. We converted these game performance criteria and the credibility, salience and legitimacy criteria to Likert questions and asked all the game participants to fill in the survey. In the Likert survey, we used five-point scales (strongly disagree, disagree, neutral, agree and strongly agree) in six statements to ask the participants about their feelings during the game, their understanding of the rules of the game, the length of the game simulation, new knowledge that they got from the game and adaptation of the game to their reality.
We organised this section by presenting the Rejoso watershed version and the Pawan–Kepulu peatland version site by site to make it easier to see the similarities and differences between the two applications, even though the Pawan–Kepulu peatland version of the H2Ours game was developed after the Rejoso watershed version.
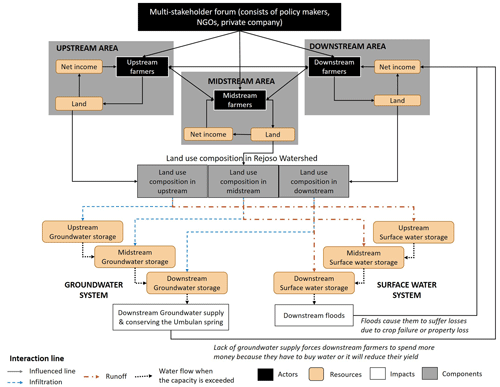
Figure 3Socio-hydrological model of the Rejoso watershed, defined using the ARDI framework. Interactions between actors themselves and between actors and the landscape influence the land use composition. The land composition affects the hydrological and economic situations, which influence the interactions reflexively. A similar socio-hydrological model with some adjustments for the Pawan–Kepulu peatland was also developed (Appendix D).
Table 1Framing problem definition for the Rejoso watershed and the Pawan–Kepulu peatland, Indonesia. Problem definition was done using the Drivers, Pressure, State, Impact and Responses (DPSIR) and Actors, Resources, Dynamics and Interaction (ARDI) frameworks, based on ICRAF and Tropenbos Indonesia research findings.
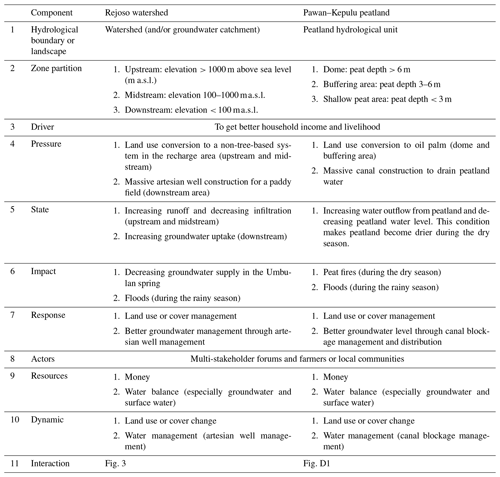
3.1 Diagnosis of the study areas and issues
Based on the results from the DPSIR and ARDI analyses, we found that the Rejoso watershed and the Pawan–Kepulu peatland showed similarities in their socio-hydrological contexts (Table 1). Stakeholder expectations for improved economic conditions led local communities to change land cover and extract excessive amounts of water resources (groundwater), causing disruption of the water balance. This disruption resulted in local communities and multi-stakeholder forums experiencing various hydrological problems, such as water shortages (or decreased groundwater levels) and flooding. However, the hydrological contexts of these two sites also differ, such as hydrological boundaries, topography, water management and interactions between stakeholders and the landscape (Figs. 3 and D1). Two proposed solutions (responses) were identified by ICRAF and Tropenbos Indonesia based on their research findings to restore hydrological functions in watersheds and peatlands, i.e. better land use management and (ground)water management (Table 1, component 7 – “Response”).
The interaction between the stakeholders and the landscape is represented by the types of decisions made by the multi-stakeholder forums and local communities regarding their landscape. Local communities (farmers from the upstream, midstream and downstream villages in the Rejoso watershed and farmers from neighbouring villages: Villages 1–4 in the Pawan–Kepulu peatland) have the authority to make decisions regarding their land, including land use and water management decisions (artesian wells in the Rejoso watershed and canal blockage in the Pawan–Kepulu peatland). Multi-stakeholder forums have authority over regulations and programmes applied to local communities to achieve their goals. They can refer to their existing or potential regulations and programmes.
3.2 Game development: the H2Ours game
3.2.1 Scope and objective of the game
The H2Ours game has the objective of helping to share knowledge and build collaboration between stakeholders in order to restore hydrological functions in a landscape. We determined the goals for the H2Ours game simulation in the two study areas to be sharing of knowledge and facilitation of collaboration, specifically for groundwater restoration and flood prevention (Table 1). However, the H2Ours game in the Rejoso watershed addressed the supply and utilisation of deep groundwater, while in the Pawan–Kepulu peatland it addressed peatland groundwater as an indicator of the wettability of peatlands and their vulnerability to land fires.
3.2.2 Roles
Based on the stakeholder identification survey in the Rejoso watershed and the Pawan–Kepulu peatland, we defined two key roles in this game, i.e. a multi-stakeholder forum and local (or farmer) communities. The goal of the multi-stakeholder forum is to prevent natural disasters, specifically water scarcity and floods in the Rejoso watershed and fires and floods in the Pawan–Kepulu peatland. In the Rejoso watershed, local communities can be grouped into people who live in the upstream village, midstream village and downstream village based on the village elevation. Meanwhile, in the Pawan–Kepulu peatland, local communities can be grouped into four groups of people living in four neighbouring villages (Village 1 to Village 4). Local communities represent landowners. Their goal is to meet their household needs (to produce sufficient food and to raise sufficient funds to pay taxes). The H2Ours game brings the various interests of these actors together and shows how they make their decisions regarding the management of land and water resources to meet their economic and environmental expectations.
Table 2Economic and environmental impacts as the rules of the H2Ours game in the Rejoso watershed. The variation in environmental components resulting from different land use options in the upstream and midstream depends on the ability of the land use options to infiltrate water, while the variation in environmental components downstream depends on the use of water based on farmers' perceptions. The rules of the H2Ours game in the Pawan–Kepulu peatland are in Appendix D (AF: agroforestry).
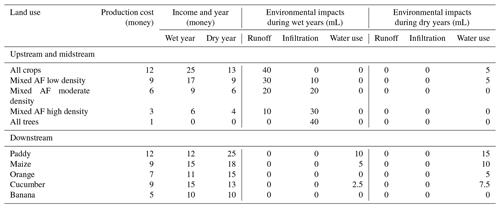
3.2.3 Rules
At the start of the game, players (i.e. multi-stakeholder forums or local communities) received a limited amount of play money. Community members were asked to manage their land to meet their household needs by arranging the land use type combination and water management in their area with the play money provided, while multi-stakeholder forums were asked to run programmes or to help reduce a local community's financial problems. Once players decided how they would manage their land or community programmes, the economic and environmental rules linked to those land use decisions were applied (Table 2). These rules then defined the dynamics of the economic and environmental conditions (see Tables 2, D1 and D2 for the Pawan–Kepulu peatland).
When during the rainy season the total surface water in the downstream area of the Rejoso watershed and in the shallow peat of the Pawan–Kepulu peatland exceeds its capacity (> 800 mL), flooding occurs. When the groundwater exceeds its capacity (> 700 mL), excess water flows to the Umbulan spring in the Rejoso watershed and to the sea in the Pawan–Kepulu peatland. However, when the groundwater was less than 200 mL, it caused water shortages for agriculture in the Rejoso watershed and made peat soil dry, which in turn triggered fires in the Pawan–Kepulu peatland. These environmental impacts decreased the overall community income. As a consequence of this, the players might not have enough money to manage their land, buy food or pay taxes in the next round of the game. The multi-stakeholder forums with their limited budget can then choose to help them by providing financial help or creating regulations or programmes to prevent these environmental problems. Through this gameplay, we aimed to encourage players to collaborate to achieve their goals.
In addition, the economic and environmental conditions during the game are influenced by the yearly weather that could be either wet or dry. In each round, the participants decided on land use, not knowing whether the next round would be a dry or wet year.
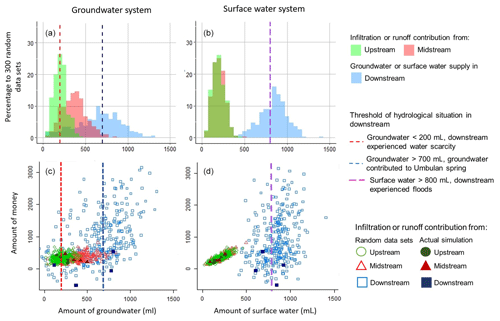
Figure 4Simulation of the hydrological and economic situations in the H2Ours game using a random value (N = 300) and actual game simulation (obs.) results (N = 4) for the Rejoso watershed. (a) Distribution of the infiltration contribution from the upstream and midstream areas and the groundwater supply downstream based on simulation with the random value. (b) Distribution of the runoff contribution from the upstream and midstream areas and surface water accumulation downstream based on simulation with the random value. (c) Groundwater situation and economic situation based on random-value simulation and actual simulation. (d) Runoff situation and economic situation based on random-value simulation and actual simulation. Appendix E provides a further analysis of the solution space.
3.2.4 Game solution space analysis
From the comparison results between 3, 10, 30, 100, 300 and 1000 computer-simulated random-walk iterations, we found that the shape and distribution of economic and environmental outcomes began to stabilise at 300 iterations. Therefore, we used 300 computer-simulated game sessions with randomly selected land and water use options as the basis for creating the solution space of this research and as a reference for the player-based game sessions. In 300 computer-simulated game runs with a random decision-making process, the groundwater distribution varied depending on the location, while the distribution of surface water in the upstream and midstream areas remained almost the same, and in the downstream area it was wider (Fig. 4a and b). Upstream and midstream had almost the same frequency distribution of surface water flows, while runoff from the upstream and midstream areas was dominated by wet years, which then may potentially cause flooding downstream in the same year. Contributions to groundwater from the upstream and midstream areas also responded to wet years, while groundwater utilisation by downstream stakeholders mostly occurred during dry years. Therefore, the frequency distributions of groundwater contributions were wider than those for surface water.
Related to the economic outcomes (Fig. 4c and d), efforts to increase infiltration in the upstream and midstream areas did not contribute much to increasing the income of the community. However, the efforts of farmers in the upstream and midstream areas to improve their economic conditions resulted in increased runoff, which caused flooding in the downstream areas. Therefore, for the downstream area, the relationship between environmental and economic conditions varies because of the influence of upstream and midstream conditions.
The presence of relationship values between humans and nature and between humans and other humans (relational values) influences decision-making regarding natural resource management (van Noordwijk et al., 2023, 2020). Therefore, the decisions made by the players during the game are influenced by various factors (e.g. interactions between players, game settings, level of player ecological knowledge) (Rodela and Speelman, 2023), while computer-simulated random decision-making is used to build the game's solution space. For example, when the upstream and midstream groups decided to maintain and improve their economic conditions, they caused a reduction in groundwater supply and increased flooding in the downstream area, which caused the downstream group to pay for the losses they experienced. Apart from that, during the game session the facilitator also provided PES scenarios (Appendix B, gameplay no. 9: repeat step 6 for the rest of the rounds with additional scenarios such as providing payment for ecosystem services). This scenario offered downstream groups to contribute a certain amount of money to maintain more trees in the upstream and midstream areas. Therefore, the downstream player groups always spend more money than the midstream and upstream player groups, either as a loss due to the environmental consequences (floods or water scarcity) or due to their efforts to prevent negative impacts by joining the PES programme.
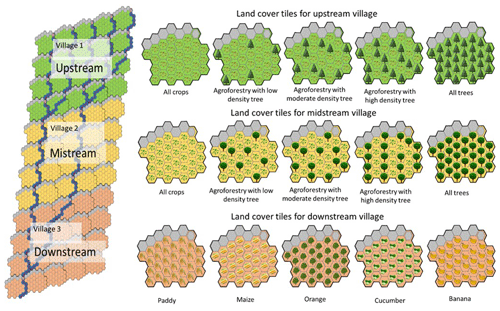
Figure 5Game board and land use and cover tiles of the H2Ours game in the Rejoso watershed. The land cover options in the upstream and midstream areas vary based on their ability to infiltrate water, while in the downstream area they vary based on farmers' perceptions of water utilisation. See Appendix D for the game materials for the Pawan–Kepulu peatland.
3.2.5 Game properties
To make the game engaging, we prepared game materials such as a game board to represent the landscape, land use tiles according to the existing and future land use types, a play money token and a water infrastructure token (Fig. 5). We also created a stylised miniature water balance (Fig. 6) to demonstrate how surface water flows can cause floods and how water infiltration increases groundwater supply. After each round calculating the economic and environmental conditions based on Table 2, we asked the players to pay production costs and taxes and get income and incentives using play money. The water balance was shown using a miniature with real water according to the produced surface water and groundwater.
3.2.6 Game testing
From the results of checking the game calculation in Excel, we adjusted the values used in the rules to ensure that these values are sensitive enough to changes in strategy by players, i.e. the initial money given to players, as well as the initial water for groundwater and surface water. The role-playing testing with project members allowed us to validate the game scenarios that would be applied in the game implementation. With the university students, we adjusted the flow of the game and set the number of rounds to 8–10 and the length of the simulation time to 2 h. With the local communities (non-targeted participants), we checked the terminology used during the game session.
3.3 Game implementation
The game session with the H2Ours game takes approximately 2 h (excluding briefing and debriefing). For the Rejoso watershed version, the 2 h game session consisted of 10 rounds with 6–12 players divided into three groups (or 2–4 players per group) acting as local communities: upstream, midstream and downstream. In the Pawan–Kepulu peatland version, the 2 h game session consisted of eight rounds with 8–16 players divided into four groups, and the players were asked to select their village name as the first step towards creating ownership. In both versions, an additional group of players consisting of two to four people can act as public stakeholders (government, companies, NGOs) and interact with villages.
During the game session, players in their roles as farmers or local communities tried to improve their household income and livelihood to at least manage until the next year. The results of the game implementation showed that there was a trade-off between economic and environmental conditions and between the upstream, midstream and downstream groups (Fig. 4 below). In the Rejoso watershed, the efforts of the upstream and midstream communities to improve their economic situation by increasing their crop area brought negative environmental impacts for downstream communities such as flooding and water scarcity. The efforts of upstream and midstream communities to reduce these problems resulted in a reduction in their economic outcomes. This situation led to negotiation between communities. In contrast, the negotiation process in the Pawan–Kepulu peatland was related to the canal blockage construction between villages with the multi-stakeholder forum. To achieve a closed hydrological system to maintain the wetness of the peatland, the construction of the canal blockage must be carried out collectively by all the villages according to the location as suggested by the multi-stakeholder forum. The construction of the canal blockage reduced the income of farmers or local communities due to decreased yield or increased harvesting costs. Furthermore, the multi-stakeholder forum persuaded the community by providing compensation for maintaining more trees to protect the peat dome area.
During the debriefing sessions, the participants in the Rejoso watershed and the Pawan–Kepulu peatland mentioned that the game showed that any decision at the plot level impacted the hydrological function at the landscape level. They also mentioned that, when they did not meet their economic needs, the economic conditions became their priority. In addition, they indicated that they would accept any regulation or programme from other stakeholders as long as their income was not significantly reduced. However, if that did happen, they expected some financial compensation. From the perspective of the multi-stakeholder forum, they said that it would be easier if the village knew what they wanted in advance so that the programmes and assistance are able to meet their needs. In addition, regulations should be complemented by supporting schemes such as compensation or incentive schemes, not just regulations issued by the government. Further analysis of these different perspectives will be presented in follow-up papers (Tanika et al., 2024, in preparation).
3.4 Game evaluation
After playing the game, the participants of both study areas were asked to fill out a survey to assess the credibility, salience and legitimacy of the game (Appendix C, Table C1). For the credibility of the game, the survey showed that, on average, 87 % of the participants from both study areas indicated that they understood the rules of the game well or even very well, while 78 % of the participants indicated that they knew the purpose of the game. For the salience and legitimacy of the game, the survey showed that 92 % of the participants gained new understanding and that 87 % said that they were able to apply the knowledge that they took away from the game to real life. Besides the credibility, salience and legitimacy criteria, we also asked the participants for their opinion of the game session process. From the survey, 87 % of the participants enjoyed the simulation and 79 % of them felt that the length of the game session was fair.
To meet the first objective of this paper to develop an adaptable serious game that can represent the socio-hydrological system, we presented a generic version of the H2Ours game as a result of the development and modification process in two different landscapes in Indonesia (Sect. 4.1). Then, to assess whether the H2Ours game can facilitate knowledge transfer and knowledge sharing regarding water use and management and whether it can support negotiation and coordination between various stakeholders as the second objective, we evaluated the H2Ours game based on input–output assessment according to evaluation criteria (Sect. 4.2).
4.1 The adaptability of the H2Ours game allows simplification of complex socio-hydrological systems
The complexity of a system is closely related to the interdependent information and interactions between elements in the system (Vidal and Marle, 2008; Rumeser and Emsley, 2019). A serious game and its associated conceptual models help to simplify this complexity by reducing the amount of information and interactions and by only showing the most relevant information from a holistic perspective (Strait and Dawson, 2006; Rumeser and Emsley, 2019). For the development of the H2Ours game, we used the DPSIR and ARDI frameworks to identify the components and interconnections of the complex socio-hydrological system of the Rejoso watershed (Table 1, column 3). The Rejoso watershed version of the H2Ours game was then adapted to the Pawan–Kepulu peatland version by modifying the socio-hydrological condition (Table 1, column 4). Therefore, these well-established frameworks act as a generic version of the H2Ours game, which can easily be modified according to other socio-hydrological realities.
The two study sites experience more complex socio-hydrological problems than represented in the H2Ours game. In our game, the water quantity issues were represented in line with national priority issues in that location, which resulted in groundwater scarcity and floods for the Rejoso watershed (Fig. 3) and fire and floods for the Pawan–Kepulu peatland (Fig. D1). In reality, the Rejoso watershed also experiences other hydrological problems, e.g. erosion and landslides in the upstream areas and water quality degradation due to the high amount of chemical fertiliser (Amaruzaman et al., 2018; Leimona et al., 2018), while the Pawan–Kepulu peatland also experiences land degradation and water contamination because of mining in the upper area of the peatland (Widayati et al., 2021). The complexity of a socio-hydrological system is formed due to many relationships and interconnections of the various components (aggregate complexity), and therefore self-organisation through gradual learning is the key to a better transformation (Manson, 2001). If all the real-life problems are included at once in the game, the risk of confusing people increases, especially those without a technical educational background (Gomes et al., 2018), which would preclude their understanding of the causes and effects of the problem. Therefore, by unravelling each individual problem and showing its causes and associated impact, players were able to expand their understanding gradually. We believe that the generic H2Ours game creates the opportunity to explore different problems, allowing the players to gain a deeper understanding and start building connections between various problems. In this way, it is possible to create opportunities to build overall socio-hydrological understanding in the future.
By comparing the H2Ours game in the two study areas, we found that there were game elements that could remain the same, while others had to be adjusted to the local situation. Game elements related to the interactions between humans or between humans and the environment (relational value) are similar in the two study areas (e.g. land use management to maximise profits, effort scenarios to restore hydrological functions, the need for coordination and negotiation among stakeholders). As such, these elements remained the same between the locations (“Driver” and “Pressure” in Table 1). However, the environmental response to the drivers and pressures generally requires technical adjustments to local conditions (e.g. hydrological boundaries, land use types and composition, water infrastructures, hydrological systems) (Table 1, “State” and “Impact”). Therefore, our generic H2Ours game (defined using the components of Table 1) proved to be easy to adapt to other problems and/or locations. In addition, it is expected to overcome the complexity of a system as we can choose the most important and most influential socio-hydrological problems that need to be addressed.
4.2 Game evaluation and lessons learned
During the game design, we evaluated the H2Ours game using the input–output assessment process (Bedwell et al., 2012). Here, credibility, salience and legitimacy were assessed throughout the different stages of the H2Ours game development (Fig. 1). During the game development of the Rejoso watershed, we assessed the credibility of the H2Ours game by relying on the biophysical and hydrological research, including hydrological modelling through the GenRiver model (Leimona et al., 2018; Suprayogo et al., 2020), while the Pawan–Kepulu peatland is based on the biophysical measurement and hydrological modelling (Tanika et al., 2024, in preparation). For the salience and legitimacy, we relied on the results of participatory research involving various stakeholders in the Rejoso watershed and the Pawan–Kepulu peatland (Amaruzaman et al., 2018; Leimona et al., 2018; Widayati et al., 2021; Leimona and Khasanah, 2022). By considering the criteria of credibility, salience and legitimacy since the data and information collection, it was easier for the H2Ours game to fulfil these criteria during the evaluation after the simulation.
We limit the evaluation in this study to the quality of the game as a product. As a serious game, H2Ours has certain goals that it needs to meet (Rodela et al., 2019), i.e. as a tool that can facilitate the transfer and sharing of knowledge from its players to support the coordination and negotiation process (Sect. 3.2.1). Evaluating the game in fulfilling its objectives is more complicated than evaluating the game session process. Ideally, the evaluation of the game in achieving its objectives can be evaluated after several simulations at various levels of simulation and should be conducted before, during and after the game sessions (Oprins et al., 2015). The evaluation of the game in meeting the objective will be carried out in the next paper by providing evidence of changes in participant perceptions.
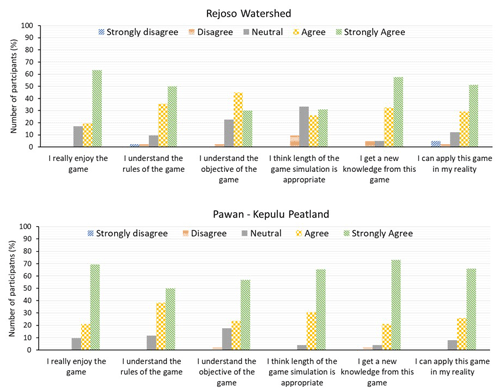
Figure 7Game evaluation from the participants in the Rejoso watershed (N = 41 people) and the Pawan–Kepulu peatland (N = 52 people).
As hydrological problems are usually complex and fundamental, any potential solution requires ample time for integrated planning and requires all relevant stakeholders to understand the dynamics of the system on a large scale (Medema et al., 2019). The H2Ours game tries to provide a simple representation of the landscape so that it makes it easier for players to be aware of the conditions of neighbouring players and to gain a system-level perspective of socio-hydrological issues. Improving player knowledge by looking at socio-hydrological problems in a broader context encourages responsible behaviour towards the environment, which is directly proportional to commitment (Keles et al., 2023). The evaluation of the game after the simulation (Fig. 7) indicated that most of the participants gained new knowledge from the game which they could apply to real life. Transparency of the rules of the H2Ours game allowed the players to see the interdependent connections between elements in the complex socio-hydrological system more clearly and made it easier for the players to explore various possibilities and to gain lessons from the reflection results (Kolbe et al., 2015; Kolb and Kolb, 2005; Fanning and Gaba, 2007). During the game session, after the players began to understand how the H2Ours game worked, the players started to initiate communication in the form of negotiations or coordination between groups or with external parties such as multi-stakeholder forums. This is in accordance with the four interacting knowledge-to-action steps in restoration strategies, where commitment begins after mutual understanding has been gained (van Noordwijk, 2018). One of the advantages of a serious game is that participants interact directly with the environment and get feedback as quickly as possible so that they can immediately analyse and correct inappropriate strategies (Bartolome et al., 2011; Feng et al., 2018). Moreover, during the H2Ours game session, players were faced with the game simulation that resembles an actual simulation, so they are indirectly encouraged to find possible solutions together as the two last parts of restoration strategies related to operationalisation and innovation.
There are several lessons learned from the H2Ours game development and simulation process in this study. First, setting up the game material with attributes of the local context helped participants to build emotions during the simulation. Second, to maintain participant commitment to restoration efforts after the game session, it is important to show that their collaborative and collective actions really worked in achieving their goals at the end of the game simulation. Third, based on the evaluation and debriefing results, even if they stated that they could apply the ideal collaborative actions that were explored in the game session, in real life, the enabling conditions needed to support this still need to be built (e.g. regulation, integrated planning strategies). As the game is a simplification of the real-life system, forms of collaborative action can be discussed directly by the players. In real life, the parties that are needed for successful collaboration may not easily meet each other to discuss issues openly. Therefore, it is necessary to create a condition where stakeholders can meet and explore collaboration options to jointly address issues and achieve goals. Without such encounters, the commitment referred to in the four knowledge-to-action chains cannot be attained.
The H2Ours game clearly showed the trade-off between the economy and the environment by calculating economic and environmental performance indicators in each round after the players changed the land use combination and water management. As a result, the relational values between humans and between humans and nature (e.g. trees and water being descended from their predecessors and being a legacy for their descendants, the use of certain woods in religious rituals) sometimes become blurred. A very clear trade-off between the economic and environmental conditions has led players to make decisions based solely on economic value. Therefore, the cost–benefit calculation of conservation activities needs to be done carefully in this game or include social values as part of the scenario in the game.
In this research, we invited participants from the upstream, midstream and downstream areas to play from the perspective of their location in the landscape. We expect that this impacted how the game was played. We intend exploring the impacts of role-switching by asking farmers to play the role of a farmer in another location in the landscape.
The generic version of the H2Ours game allows for exploration of the complexity of a socio-hydrological system. The game can easily be modified according to different needs and conditions. The complexity of the socio-hydrological system can be applied separately and/or simultaneously, depending on the knowledge level of the intended participants. With an adaptable game such as the one developed, the game designer can adjust the level of complexity included in the game and even include an advanced simulation that combines all possible problems and interactions found in a socio-hydrological system.
The H2Ours game was able to facilitate knowledge transfer and knowledge sharing and triggered collaborative actions by simplification in time and space. The H2Ours game saves time because the transparency of the rules allows the players to see that the restoration target is something that can be achieved in the future with a clearer perspective by exploring various strategies and scenarios during the game sessions. Space simplification allowed the players to see the entire landscape and the relationships between components that influence each other. In addition, they can easily see the various enabling conditions needed to implement the strategies in the game in real terms (e.g. the need for multi-stakeholder collaboration or a restoration master plan).
One of the challenges in developing or modifying the H2Ours game is to provide values for the economic and environmental impact components for each type of land use. Here is a simple guide to modifying the H2Ours game rules.
-
Determine the types of land use in the landscape. If the land use types are varied enough, take the four to six most dominant land covers, including the new land use types that might be intervened in.
-
For each type of land use, determine the economic value (production costs and income) and the environmental value (runoff, infiltration, water use). The value used as a rule does not have to be the actual value. You may only use the ratio between the land use types after setting up the maximum and minimum values. A simple method to collect this information is to conduct a survey with several farmers and ask them to rank or score the land use type based on their economic and environmental impacts (Fig. A1).
-
Determine infrastructures to be used in games that might affect economic and environmental conditions (e.g. artesian wells for irrigation, canal blockage, water storage).
Determine how each of these infrastructures affects economic and environmental conditions (e.g. artesian wells, construction costs, threats, amount of groundwater extraction). You can conduct a survey to collect that information and then normalise the value following the economic and environmental values.
-
During the game testing, assess those values with the participant as to whether they are reasonable and represent their actual condition.
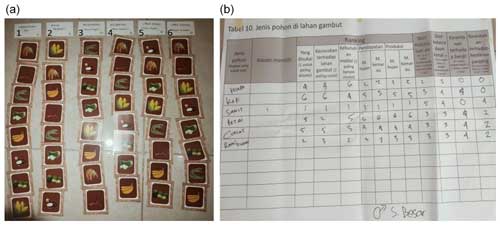
Figure A1(a) An example of the results from sorting the types of land use in the Rejoso watershed by one of the local farmers: (1) water use, (2) production costs, (3) income during the wet season, (4) income during the dry season, (5) preferences during the wet season and (6) preferences during the dry season. For the water balance component, we derived the hydrological model parameterisation. (b) Example results from scoring land use types during the focus group discussion with some farmers in the Pawan–Kepulu peatland to collect information about preferences, peat soil suitability, production costs, income during the wet and dry seasons, yields during the wet and dry seasons, water use, dependence on the presence of a canal, vulnerability rate regarding floods and vulnerability rate regarding drought.
Overview | Simulation of the impact of land use or cover change and water management on the hydrological situation (water balance) |
Objective | Knowledge sharing and decision-making to support collaborative and collective actions among stakeholders |
Benefits |
|
Duration | 2 h (or around 8–10 rounds) |
Number of players | 6–16 players |
Material |
|
Game play
-
Welcome all the players and give a general introduction to the workshop and game or simulation.
-
Select two to three people from the players to act as public stakeholders whose role is the management of the whole watershed or peatland by providing regulations or programmes to prevent various environmental problems (optional).
-
Group the remaining players into three groups (for the watershed version) or four groups (for the peatland version) to represent the farmers from different villages. During the game simulation, their goals are to live happily by fulfilling their needs.
-
Brief players by giving explanations and definitions of the terminology that is often used in the game, and build connections between the game properties and their actual situation so that the decisions made by the players are very close to their reality.
-
Introduce a co-facilitator for each group to help calculation of economic resources (optional).
-
Give initial money to the players (300–450 per group) and initial groundwater and surface water in the water balance simulation model.
-
Start the round by asking the players to decide on their land use system, and then calculate the economic and environmental impacts based on the (random) weather situation in that round.
-
Repeat step 6 for rounds 2 and 3 as the warm-up.
-
Repeat step 6 for the rest of the rounds with additional scenarios, such as announcing regulation by the government or providing payment for ecosystem programmes. Through discussions or interviews you can develop the scenarios based on the stakeholder perceptions of what they should do to restore the hydrological function.
-
Hold a debriefing session by asking the players about their strategies for achieving their goals and about their feelings during the game simulation.
In this study, we refer to the criteria of credibility, salience and legitimacy of Belcher et al. (2016) in the development and evaluation processes of the H2Ours game. Table C1 shows the criteria that we consider most relevant for representing the objective of the H2Ours game to facilitate knowledge transfer and sharing to support negotiation and collaboration among stakeholders. To use these criteria, we adjusted the definition of each criterion from the original definition (column 3) to a definition that meets the objectives of the H2Ours game (column 4). Then, how we include each criterion in the development and evaluation process of the H2Ours game is shown in columns 5 and 6.
Based on some references, focus ground discussions and interviews with various stakeholders in the Pawan–Kepulu peatland, we found that this area experiences land and forest fires during the dry year (season) and flood during the wet year (season). Land cover conversion from forest to oil palm plantation and crop season has led to massive canal construction to get better production. This situation makes the landscape drier during the dry year and vulnerable to fires.
The hydrological boundary of peatland is a peatland hydrological unit (PHU) as an area between two rivers. Usually in this landscape there is a peat dome (the deepest peat area), an area surrounding the peat dome (i.e. the buffering dome area) and an area with shallow peat. Villages are spread over the peat dome and the buffer zone, with them having different proportions of peat dome and buffer zone areas. However, for simplification, peat depth (including that of the peat domes) was distributed evenly between the villages (Fig. D1). However, for future game adaptations, the peat depth distributions in each village can be adjusted on the game board.
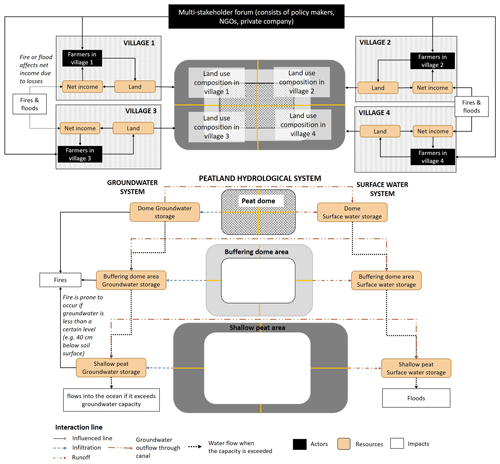
Figure D1Socio-hydrological model defined using the ARDI framework that was used to design the H2Ours game for the Pawan–Kepulu peatland. Interactions between actors themselves and between actors and the landscape influence land use composition, which affects the hydrological and economic situation, and then it influences interaction back.
D1 Rules of the game
Based on measurement data, focus group discussions with local farmers and some references, we designed the rules of the H2Ours game for the peatland version by combining six land use options (i.e. all trees, all oil palm, oil palm + trees, oil palm + seasonal crops, all crops, shrub or burnt area) and three canal density options (without a canal, with low- and high-density canals) (Tables D1 and D2).
Table D1Economic impacts in the Pawan–Kepulu peatland version together with the production costs in the dome area +2/plot and in the buffering dome area +1/plot.
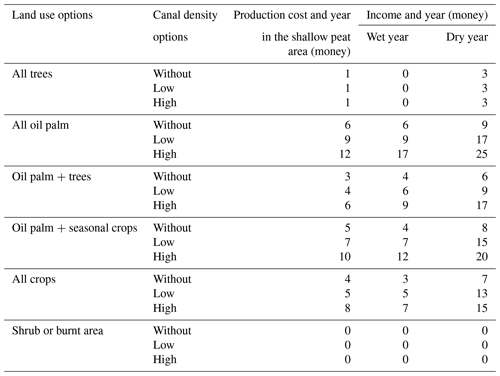
D2 Game properties
The component of the H2Ours game for the peatland version is similar to the watershed version, with modification on the board as the landscape and land use options (Fig. D2). The board is designed in such a way that it resembles a PHU with a dome in the middle, a buffering area around the dome and shallow peat on the outside. In the real simulation, we can add rivers and roads to help the players have a connection with their real situation.
Similar to the Rejoso watershed, the H2Ours game for peatlands also has the same water balance miniature (Fig. D3). This water balance model follows the hydrological system in Fig. D1. In the groundwater system, each tank has a fire vulnerability threshold. This threshold represents 40 cm below the soil surface in its actual condition as stipulated by government regulations. If the groundwater in each zone is below this limit, then the area has the potential for fires, which causes harm to the local community.
In addition to the H2Ours game for the peatland version, there is a peat infrastructure token in the form of canal blockage and firefighters (Fig. D4). In reality, the canal blockage blocks the canal to reduce or stop the groundwater outflow. In this game simulation the canal blockage changes the land use from high- to low-density canals or from low density to without a canal. Firefighters helps to prevent fires in plots during the dry year or season. However, providing canal blockage and firefighters costs some money.
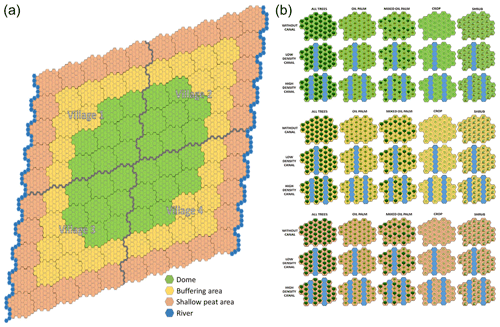
Figure D2Board of the H2Ours game for the peatland version that consists of the dome area, buffering dome area and shallow peat area (a). Land use options (all trees, all oil palm, oil palm + trees, all crops and shrubs) with two canal densities without, low canal density, and high canal density) for the Pawan–Kepulu peatland area (b).
The rules of the game determine the possible outcomes or solution space, within which the specific choices made by the game participants are located. If all the choices are random (equal probability of all choices available) without responses to the outcomes so far, substantial variation in outcomes is possible. The primary outcomes of interest are the surface water flows (rainfall not used as canopy interception evaporation or infiltration into the soil) and the groundwater flows (water infiltrating and not used for subsequent evapotranspiration), all depending on both land cover and rainfall.
The first question in defining this solution space is the number of random series that need to be evaluated to accurately estimate the frequency distributions of outcomes in various response parameters. We present data for 3, 10, 30, 100, 300 and 1000 iterations (Figs. E1–E4) (each including 10 rounds and 3 zones and thus 30 land use choices and 10 weather conditions, dry or wet). The actual game simulation was only done four times; therefore, the closest solution space has 3 or 10 random values, which is not sufficiently representative of the distribution. Based on Figs. E1 and E2, the solution space distribution pattern starts to appear in 30 random data sets. Therefore, to see the actual distribution of the farmers' decision-making, we need at least 30 game simulations. Figures E3 and E4 show that the relationship between economic conditions (money) and the environment (groundwater and surface water) in the downstream area is more scattered compared to the upstream and midstream areas. However, related to groundwater supply downstream (Fig. E3), the groundwater supply and the economic benefits obtained are higher. By contrast, the higher runoff obtained from the upstream and midstream areas (Fig. E4) will decrease their economic benefits.
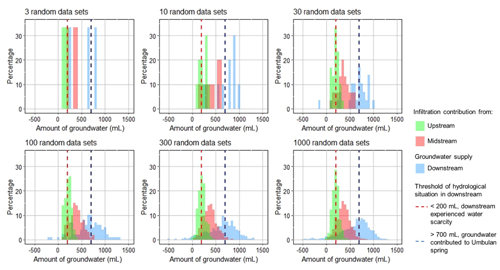
Figure E1Distribution of the infiltration contribution from the upstream and midstream areas and groundwater supply in the downstream area based on simulation with the random value.
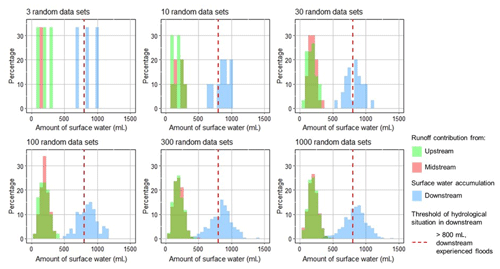
Figure E2Distribution of the runoff contribution from the upstream and midstream areas and surface water accumulation in the downstream area based on simulation with the random value.
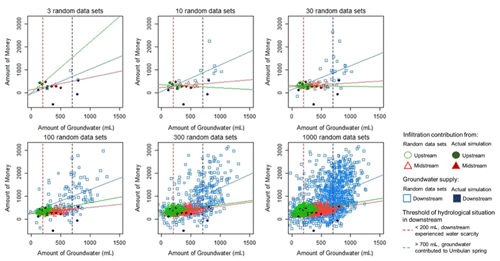
Figure E3Groundwater and economic conditions based on random-value simulation and actual simulation.
All raw data can be provided by the corresponding authors upon request.
LT, RRS and ALH designed the research project. LT, RRS and ENS designed the game. LT performed the game simulation and game analysis. LT, MvN, MPC and ENS wrote the manuscript. EP and BL gave input on the performance of the game simulation in each case study area.
The contact author has declared that none of the authors has any competing interests.
Publisher's note: Copernicus Publications remains neutral with regard to jurisdictional claims made in the text, published maps, institutional affiliations, or any other geographical representation in this paper. While Copernicus Publications makes every effort to include appropriate place names, the final responsibility lies with the authors.
We appreciate the valuable input from the colleagues of ICRAF and Tropenbos Indonesia during the H2Ours game development and game implementation. We would like to express our deep gratitude to the students of Merdeka University (Pasuruan, East Java), Tanjung Pura University (Pontianak, West Kalimantan) and Brawijaya University (Malang, East Java), who participated in the H2Ours game testing. This study was funded by Tropenbos Indonesia as part of the Working Landscape and Fires project, ICRAF as part of the Rejoso Kita project and INREF as part of the SESAM project.
This research has been supported by Tropenbos Indonesia, ICRAF and INREF.
This paper was edited by Roger Moussa and reviewed by Thomas Falk and two anonymous referees.
Amaruzaman, S., Khasanah, N., Tanika, L., Dwiyanti, E., Lusiana, B., Leimona, B., and Janudianto: Landscape characteristics of Rejoso Watershed: land cover dynamics, farming systems and community strategies, , Bogor, Indonesia, 106 pp., https://www.cifor-icraf.org/knowledge/ (last access: 21 August 2024), 2018.
Bartolome, N. A., Zorrilla, A. M., and Zapirain, B. G.: Can game-based therapies be trusted? Is game-based education effective? A systematic review of the Serious Games for health and education, in: 16th International Conference on Computer Games (CGAMES), 27–30 July 2011, Louisville, KY, USA, 275–282, https://doi.org/10.1109/CGAMES.2011.6000353, 2011.
Bedwell, W. L., Pavlas, D., Heyne, K., Lazzara, E. H., and Salas, E.: Toward a taxonomy linking game attributes to learning: An empirical study, Simulat. Gaming, 43, 729–760, https://doi.org/10.1177/1046878112439444, 2012.
Belcher, B. M., Rasmussen, K. E., Kemshaw, M. R., and Zornes, D. A.: Defining and assessing research quality in a transdisciplinary context, Res. Evaluat., 25, 1–17, https://doi.org/10.1093/reseval/rvv025, 2016.
Carlson, K. M., Curran, L. M., Ratnasari, D., Pittman, A. M., Soares-Filho, B. S., Asner, G. P., Trigg, S. N., Gaveau, D. A., Lawrence, D., and Rodrigues, H. O.: Committed carbon emissions, deforestation, and community land conversion from oil palm plantation expansion in West Kalimantan, Indonesia, P. Natl. Acad. Sci. USA, 109, 7559–7564, https://doi.org/10.1073/pnas.1200452109, 2012.
Cash, D., Clark, W. C., Alcock, F., Dickson, N., Eckley, N., and Jager, J.: Salience, Credibility, Legitimacy and Boundaries: Linking Research, Assessment and Decision Making, SSRN Electron. J., https://doi.org/10.2139/ssrn.372280, 2002.
Creed, I., van Noordwijk, M., Archer, E., Claassen, M., Ellison, D., Jones, J. A., McNulty, S. G., Vira, B., and Wei, X.: Forest, trees and water on a changing planet: How contemporary science can inform policy and practice, in: For. Water a Chang. Planet Vulnerability, Adapt. Gov. Oppor. A Glob. Assess. Rep., 38, edited by: Creed, I. F. and van Noordwijk, M., IUFRO World Ser., https://forestthreats.org/products/publications/Forest_Trees_and_Water_on_a_Changing_Planet.pdf (last access: 21 August 2024), 171–175, 2018.
Crookall, D.: Debriefing: A practical guide To cite this version: HAL Id: hal-03904518 Debriefing: A practical guide, in: Simulation for Participatory Education: Virtual Exchange and Worldwide Collaboration, Springer, https://doi.org/10.1007/978-3-031-21011-2_6, 2023.
Djuwansyah, M. R.: Environmental sustainability control by water resources carrying capacity concept: Application significance in Indonesia, IOP Conf. Ser. Earth Environ. Sci., 118, 1–6, https://doi.org/10.1088/1755-1315/118/1/012027, 2018.
D'Odorico, P., Carr, J., Dalin, C., Dell'Angelo, J., Konar, M., Laio, F., Ridolfi, L., Rosa, L., Suweis, S., Tamea, S., and Tuninetti, M.: Global virtual water trade and the hydrological cycle: Patterns, drivers, and socio-environmental impacts, Environ. Res. Lett., 14, 5, https://doi.org/10.1088/1748-9326/ab05f4, 2019.
Etienne, M., du Toit, D. R., and Pollard, S.: ARDI: A co-construction method for participatory modeling in natural resources management, Ecol. Soc., 16, 1, https://doi.org/10.5751/ES-03748-160144, 2011.
Fanning, R. M. and Gaba, D. M.: The role of debriefing in simulation-based learning, Simul. Healthc., 2, 115–125, https://doi.org/10.1097/SIH.0b013e3180315539, 2007.
Feng, Z., González, V. A., Amor, R., Lovreglio, R., and Cabrera-Guerrero, G.: Immersive virtual reality serious games for evacuation training and research: A systematic literature review, Comput. Educ., 127, 252–266, https://doi.org/10.1016/j.compedu.2018.09.002, 2018.
Ferguson, C., van den Broek, E. L., and van Oostendorp, H.: On the role of interaction mode and story structure in virtual reality serious games, Comput. Educ., 143, 1–13, https://doi.org/10.1016/j.compedu.2019.103671, 2020.
Fleming, T. M., Cheek, C., Merry, S. N., Thabrew, H., Bridgman, H., Stasiak, K., Shepherd, M., Perry, Y., and Hetrick, S.: Serious games for the treatment or prevention of depression: A systematic review, Revista de Psicopatología y Psicología Clínica, 19, 3, https://doi.org/10.5944/rppc.vol.19.num.3.2014.13904, 2014.
Fleming, T. M., de Beurs, D., Khazaal, Y., Gaggioli, A., Riva, G., Botella, C., Baños, R. M., Aschieri, F., Bavin, L. M., Kleiboer, A., Merry, S., Lau, H. M., and Riper, H.: Maximizing the impact of E-Therapy and Serious Gaming: Time for a paradigm shift, Front. Psychiatry, 7, 65, https://doi.org/10.3389/fpsyt.2016.00065, 2016.
Gomes, S. L., Hermans, L. M., Islam, K. F., Huda, S. N., Hossain, A. Z., and Thissen, W. A. H.: Capacity building for water management in peri-urban communities, Bangladesh: A simulation-gaming approach, Water (Switzerland), 10, 11, https://doi.org/10.3390/w10111704, 2018.
Guo, Y., Zhang, Y., Zhang, L., and Wang, Z.: Regionalization of hydrological modeling for predicting streamflow in ungauged catchments: A comprehensive review, WIREs. Water, 8, e1487, https://doi.org/10.1002/wat2.1487, 2021.
IPBES: The IPBES assessment report on land degradation and restoration, edited by: Montanarella, L., Scholes, R., and Brainich, A., Secretariat of the Intergovernmental Science-Policy Platform on Biodiversity and Ecosystem Services, Bonn, Germany, https://doi.org/10.4324/9781315640051-105, 744 pp., 2018.
Jeanes, K., van Noordwijk, M., Joshi, L., Widayati, A., Leimona, F., and Leimona, B.: Rapid Hydrological Appraisal in the context of environmental service rewards, World Agroforestry, Bogor, Indonesia, 56 pp., 2006.
Keles, H., Yayla, O., Tarinc, A., and Keles, A.: The Effect of Environmental Management Practices and Knowledge in Strengthening Responsible Behavior: The Moderator Role of Environmental Commitment, Sustainability, 15, 2, https://doi.org/10.3390/su15021398, 2023.
Khasanah, N., Tanika, L., Pratama, L. D. Y., Leimona, B., Prasetiyo, E., Marulani, F., Hendriatna, A., Zulkarnain, M. T., Toulier, A., and van Noordwijk, M.: Groundwater-Extracting Rice Production in the Rejoso, Land, 10, 586, https://doi.org/10.3390/land10060586, 2021.
Kim, Y. J. and Yoo, J. H.: The utilization of debriefing for simulation in healthcare: A literature review, Nurse Educ. Pract., 43, 102698, https://doi.org/10.1016/j.nepr.2020.102698, 2020.
Kolb, A. Y. and Kolb, D. A.: Learning styles and learning spaces: Enhancing experiential learning in higher education, Acad. Manag. Learn. Edu., 4, 193–212, https://doi.org/10.5465/AMLE.2005.17268566, 2005.
Kolbe, M., Grande, B., and Spahn, D. R.: Briefing and debriefing during simulation-based training and beyond: Content, structure, attitude and setting, Best Pract. Res. Cl. Anaesthesiol., 29, 87–96, https://doi.org/10.1016/j.bpa.2015.01.002, 2015.
Leimona, B. and Khasanah, N.: Co-Investment for sustainable production landscape in the downstream Rejoso Watershed, Indonesia Country Program, World Agroforestry (ICRAF), Bogor, Indonesia, 164 pp., 2022.
Leimona, B., Khasanah, N., Lusiana, B., Amaruzaman, S., Tanika, L., Hairiah, K., Suprayogo, D., Pambudi, S., and Negoro, F. S.: A business case: co-investing for ecosystem service provisions and local livelihoods in Rejoso watershed, World Agroforestry Centre (ICRAF) Southeast Asia Regional Program, Bogor, Indonesia, 39 pp., ISBN: 978-979-3198-99-6, https://publikasi.agroforestri.id/sea/detail?pubID=4306 (last access: 21 August 2024), 2018.
Lohmann, D., Falk, T., Geissler, K., Blaum, N., and Jeltsch, F.: Determinants of semi-arid rangeland management in a land reform setting in Namibia, J. Arid Environ., 100–101, 23–30, https://doi.org/10.1016/j.jaridenv.2013.10.005, 2014.
Lu, M., Wang, S., Wang, X., Liao, W., Wang, C., Lei, X., and Wang, H.: An Assessment of Temporal and Spatial Dynamics of Regional Water Resources Security in the DPSIR Framework in Jiangxi Province, China, Int. J. Env. Res. Pub. He., 19, 3650, https://doi.org/10.3390/ijerph19063650, 2022.
Manson, S. M.: Simplifying complexity: a review of complexity theory, Geoforum, 32, 405–414, https://doi.org/10.1016/S0016-7185(00)00035-X, 2001.
Marini, D., Medema, W., Adamowski, J., Veissière, S. P. L., Mayer, I., and Wals, A. E. J.: Socio-psychological perspectives on the potential for serious games to promote transcendental values in IWRM decision-making, Water (Switzerland), 10, 1097, https://doi.org/10.3390/w10081097, 2018.
Mazzucato, M., Okonjo-Iweala, N., Rockstrom, J., and Shanmugaratnam, T.: Turning the Tide: A Call to Collective Action, Global Commission on the Economics of Water, Paris, 2023.
Medema, W., Mayer, I., Adamowski, J., Wals, A. E. J., and Chew, C.: The potential of serious games to solve water problems: Editorial to the special issue on game-based approaches to sustainable water governance, Water (Switzerland), 11, 2562, https://doi.org/10.3390/w11122562, 2019.
Mitgutsch, K. and Alvarado, N.: Purposeful by design?: A serious game design assessment framework, in: Foundations of Digital Games 2012, FDG 2012 – Conference Program, Raleigh North Carolina, 29 May–1 June 2012, 121–128, https://doi.org/10.1145/2282338.2282364, 2012.
Murdiyarso, D., Lestari, I., Hanggara, B. B., Saragi-Sasmito, M., Basuki, I., and Taufik, M.: Managing Water Regimes Controlling Greenhouse Gas Emissions and Fires in Indonesian Tropical Peat Swamp Forests, in: Wetland Carbon and Environmental Management, American Geophysical Union, 355–369, https://doi.org/10.1002/9781119639305.ch19, 2021.
Oprins, E., Visschedijk, G., Roozeboom, M. B., Dankbaar, M., Trooster, W., and Schuit, S. C. E.: The game-based learning evaluation model (GEM): Measuring the effectiveness of serious games using a standardised method, Int. J. Technol. Enhanc. Learn., 7, 326–345, https://doi.org/10.1504/IJTEL.2015.074189, 2015.
Rodela, R. and Speelman, E. N.: Serious games in natural resource management: steps towards assessment of their contextualized impacts, Curr. Opin. Env. Sust., 65, 101375, https://doi.org/10.1016/j.cosust.2023.101375, 2023.
Rodela, R., Ligtenberg, A., and Bosma, R.: Conceptualizing serious games as a learning-based intervention in the context of natural resources and environmental gove, Water (Switzerland), 11, 245, https://doi.org/10.3390/w11020245, 2019.
Rosa, L., Rulli, M. C., Davis, K. F., Chiarelli, D. D., Passera, C., and D'Odorico, P.: Closing the yield gap while ensuring water sustainability, Environ. Res. Lett., 13, 104002, https://doi.org/10.1088/1748-9326/aadeef, 2018.
Rossano, V., Roselli, T., and Calvano, G.: A serious game to promote environmental attitude, Smart Innov. Syst. Tech., 75, 48–55, https://doi.org/10.1007/978-3-319-59451-4_5, 2017.
Rudolph, J. W., Raemer, D. B., and Simon, R.: Establishing a safe container for learning in simulation the role of the presimulation briefing, Simul. Healthc., 9, 339–349, https://doi.org/10.1097/SIH.0000000000000047, 2014.
Rumeser, D. and Emsley, M.: Can Serious Games Improve Project Management Decision Making Under Complexity?, Proj. Manag. J., 50, 23–39, https://doi.org/10.1177/8756972818808982, 2019.
Savic, D. A., Morley, M. S., and Khoury, M.: Serious gaming for water systems planning and management, Water (Switzerland), 8, 456, https://doi.org/10.3390/w8100456, 2016.
Silva, F. G. M.: Practical methodology for the design of educational serious games, Information, 11, 1–13, https://doi.org/10.3390/info11010014, 2020.
Singh, G. and Kumar, E.: Input data scale impacts on modeling output results: A review, J. Spat. Hydrol., 13, https://www.researchgate.net/ (last access: 21 August 2024), 2017.
Speelman, E. N., García-Barrios, L. E., Groot, J. C. J., and Tittonell, P.: Gaming for smallholder participation in the design of more sustainable agricultural landscapes, Agr. Syst., 126, 62–75, https://doi.org/10.1016/j.agsy.2013.09.002, 2014.
Strait, C. and Dawson, R.: Too much information, https://www.pmi.org/learning/library/point-information-overload-management-process-2769# (last access: 21 August 2024), 2006.
Sun, S., Wang, Y., Liu, J., Cai, H., Wu, P., Geng, Q., and Xu, L.: Sustainability assessment of regional water resources under the DPSIR framework, J. Hydrol., 532, 140–148, https://doi.org/10.1016/j.jhydrol.2015.11.028, 2016.
Suprayogo, D., van Noordwijk, M., Hairiah, K., Meilasari, N., Rabbani, A. L., Ishaq, R. M., and Widianto, W.: Infiltration-Friendly Agroforestry Land Uses on, Land, 9, 240, https://doi.org/10.3390/land9080240, 2020.
Toulier, A.: Multidisciplinary study for the characterization of volcanic aquifers hydrogeological functioning: case of Bromo-Tengger volcano (East Java, Indonesia), Thèse, 272 pp. https://theses.hal.science/tel-02488883v1/file/2019_TOULIER_archivage.pdf (last access: 21 August 2024), 2019.
Tsai, W. P., Feng, D., Pan, M., Beck, H., Lawson, K., Yang, Y., Liu, J., and Shen, C.: From calibration to parameter learning: Harnessing the scaling effects of big data in geoscientific modeling, Nat. Commun., 12, 1, https://doi.org/10.1038/s41467-021-26107-z, 2021.
van Noordwijk, M.: Agroforestry as part of climate change response, IOP Conf. Ser. Earth Environ. Sci., 200, 1, https://doi.org/10.1088/1755-1315/200/1/012002, 2018.
van Noordwijk, M., Lusiana, B., Leimona, B., Dewi, S., and Wulandari, D. (Eds.): Negotiation-support toolkit for learning landscapes, edited World Agroforestry Southeast Asia Regional Program, World Agroforestry Centre (ICRAF) Southeast Asia Regional Program, ISBN: 978-979-3198-74-3, 2013.
van Noordwijk, M., Tanika, L., and Lusiana, B.: Flood risk reduction and flow buffering as ecosystem services – Part 2: Land use and rainfall intensity effects in Southeast Asia, Hydrol. Earth Syst. Sci., 21, 2341–2360, https://doi.org/10.5194/hess-21-2341-2017, 2017.
van Noordwijk, M., Speelman, E., Hofstede, G. J., Farida, A., Kimbowa, G., Geraud, G., Assogba, C., Best, L., and Tanika, L.: Sustainable Agroforestry Landscape Management: Changing the Game, Land, 9, 1–38, 2020.
van Noordwijk, M., van Oel, P., Muthuri, C., Satnarain, U., Sari, R. R., Rosero, P., Githinji, M., Tanika, L., Best, L., Comlan Assogba, G. G., Kimbowa, G., Andreotti, F., Lagneaux, E., Wamucii, C. N., Hakim, A. L., Miccolis, A., Abdurrahim, A. Y., Farida, A., Speelman, E., and Hofstede, G. J.: Mimicking nature to reduce agricultural impact on water cycles: A set of mimetrics, Outlook Agr., 51, 114–128, https://doi.org/10.1177/00307270211073813, 2022.
van Noordwijk, M., Villamor, G. B., Hofstede, G. J., and Speelman, E. N.: Relational versus instrumental perspectives on values of nature and resource management decisions, Curr. Opin. Env. Sust., 65, 101374, https://doi.org/10.1016/j.cosust.2023.101374, 2023.
van Voorn, G. A. K., Verburg, R. W., Kunseler, E. M., Vader, J., and Janssen, P. H. M.: A checklist for model credibility, salience, and legitimacy to improve information transfer in environmental policy assessments, Environ. Modell. Softw., 83, 224–236, https://doi.org/10.1016/j.envsoft.2016.06.003, 2016.
Vidal, L. A. and Marle, F.: Understanding project complexity: Implications on project management, Kybernetes, 37, 1094–1110, https://doi.org/10.1108/03684920810884928, 2008.
Villamor, G. B., Griffith, D. L., Kliskey, A., and Alessa, L.: Contrasting stakeholder and scientist conceptual models of food-energy-water systems: a case study in Magic Valley, Southern Idaho, Socio-Environmental Syst. Model., 2, 16312, https://doi.org/10.18174/sesmo.2020a16312, 2019.
Villamor, G. B., van Noordwijk, M., and Troitzsch, K. G.: Triangulating agent-based models, role-playing games, and a stakeholder-centric approach to change scenarios, Curr. Opin. Env. Sust., 64, 101323, https://doi.org/10.1016/j.cosust.2023.101323, 2023.
Widayati, A., Louman, B., Mulyoutami, E., Purwanto, E., Kusters, K., and Zagt, R.: Communities' adaptation and vulnerability to climate change: Implications for achieving a climate-smart landscape, Land, 10, 1–20, https://doi.org/10.3390/land10080816, 2021.
Zhao, Q., Bai, J., Huang, L., Gu, B., Lu, Q., and Gao, Z.: A review of methodologies and success indicators for coastal wetland restoration, Ecol. Indic., 60, 442–452, https://doi.org/10.1016/j.ecolind.2015.07.003, 2016.
- Abstract
- Introduction
- Methodology
- Results
- Discussion
- Conclusion
- Appendix A: H2Ours rule development
- Appendix B: Guideline for facilitating the H2Ours game
- Appendix C: Criteria of credibility, salience and legitimacy
- Appendix D: H2Ours game for the peatland version (case study for the Pawan–Kepulu peatland)
- Appendix E: Solution space of the H2Ours game in the Rejoso watershed
- Data availability
- Author contributions
- Competing interests
- Disclaimer
- Acknowledgements
- Financial support
- Review statement
- References
- Abstract
- Introduction
- Methodology
- Results
- Discussion
- Conclusion
- Appendix A: H2Ours rule development
- Appendix B: Guideline for facilitating the H2Ours game
- Appendix C: Criteria of credibility, salience and legitimacy
- Appendix D: H2Ours game for the peatland version (case study for the Pawan–Kepulu peatland)
- Appendix E: Solution space of the H2Ours game in the Rejoso watershed
- Data availability
- Author contributions
- Competing interests
- Disclaimer
- Acknowledgements
- Financial support
- Review statement
- References