the Creative Commons Attribution 4.0 License.
the Creative Commons Attribution 4.0 License.
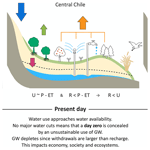
HESS Opinions: The unsustainable use of groundwater conceals a “Day Zero”
Camila Alvarez-Garreton
René Garreaud
Javier González
Roberto Rondanelli
Eugenia Gayó
Mauricio Zambrano-Bigiarini
Water scarcity is a pressing global issue driven by increasing water demands and changing climate conditions. Based on novel estimates of water availability and water use in Chile, we examine the challenges and risks associated with groundwater (GW) withdrawals in the country's central-north region (27–35° S), where extreme water stress conditions prevail. As total water use within a basin approaches the renewable freshwater resources, the dependence on GW reserves intensifies in unsustainable ways. This overuse has consequences that extend beyond mere resource depletion, manifesting into environmental degradation, societal conflict, and economic costs. We argue that the “Day Zero” scenario, often concealed by the uncertain attributes of GW resources, calls for a reconsideration of water allocation rules and a broader recognition of the long-term implications of unsustainable GW use. Our results offer insights for regions worldwide facing similar water scarcity challenges and emphasize the importance of proactive and sustainable water management strategies.
- Article
(3986 KB) - Full-text XML
- BibTeX
- EndNote
The risk of water scarcity in a basin increases as the water demand approaches the available renewable freshwater resources, hereafter referred to as water availability. Water availability at the basin scale is accounted for as the difference between total precipitation and natural evapotranspiration (ET), excluding land use-induced perturbations. When the ratio of total water uses to water availability – the water stress index (WSI) – exceeds 40 %, a basin is considered highly water stressed (Oki and Kanae, 2006). In extreme cases, when the time series of water availability and total water demands within a basin intersect or get too close to each other (i.e., WSI = 100 %), a “Day Zero” (D0) may occur. During a D0 event, water cuts are applied to prioritize water access for human consumption, and the ecological flows to maintain the well-being of ecosystems cannot be safeguarded. The concept of D0 has faced criticism from the scientific community (Warner and Meissner, 2021), which attributes to it a sensationalist use and lack of scientific robustness, among other concerns. Nevertheless, it generates a sense of urgency that puts pressure on decision-makers to take actions.
A well-known D0 event almost happened in 2018 in South Africa in the context of a severe multi-year drought, when Cape Town was at risk of being the first major city worldwide to run out of drinking water due to the low dam levels. The announcement of an imminent D0 on a specific date (12 April 2018, estimated based on the remaining water stored in the reservoir and the water use requirements from the city) triggered water saving strategies that, along with the arrival of winter precipitation interrupting the drought, allowed the city to avoid drastic water cuts (Maxmen, 2018; Burls et al., 2019). Another near-D0 situation occurred in July 2023 in Montevideo, capital of Uruguay. Due to low water reserves in the main reservoirs that provide drinking water to the city, the supply was replaced with brackish water from the La Plata river. As a consequence, the metropolitan area of Uruguay received non-drinkable water from the taps. Public opinion criticized this measure, claiming it masked a D0 situation by avoiding supply cuts at the expense of providing non-potable water (Gudynas, 2023).
Besides some well-known cases in metropolitan areas (Ahmadi et al., 2020), many basins around the globe are approaching or have already reached the point where water uses intersect with or surpass water availability, especially in water-limited regions with intensive irrigation (Oki and Kanae, 2006). In those cases, water needs are usually met by exploiting groundwater (GW) reservoirs at rates that exceed natural recharge, which is unsustainable in the long term (de Graaf et al., 2019). Indeed, many societies have supported agricultural and population growth by pumping GW storage (Bierkens and Wada, 2019).
An increasing number of studies are raising the alarm on the unsustainable use of GW worldwide, highlighting potential threats to water security and food security (e.g., Gleeson et al., 2016; Schwartz et al., 2020). These studies recognize that accessing GW savings is crucial for addressing water scarcity, especially during droughts. However, depending on GW saving becomes risky when over-extraction renders it a scarce or depleted resource. Although assessing the limits beyond which GW extraction becomes unsustainable remains an ongoing research and technical challenge, we know that pumping rates exceeding the natural GW recharge leads to long-term decline in reserves beyond those expected from climate variability (Bierkens and Wada, 2019). From that perspective, the evidence of a long-term decline in water table can be used to infer or anticipate an unsustainable GW use in a basin.
Here, we build upon the above evidence and emphasize that a D0 scenario, which typically triggers alarms and immediate management responses when it is associated with surface reservoir depletions, may be concealed by an unsustainable use of GW. To illustrate this, we focus on the central-north region of Chile, where GW overexploitation and its potential consequences have recently been reported by Taucare et al. (2024) and Jódar et al. (2023). By following different approaches, those studies assessed the effects of climate variability and GW withdrawals on GW level changes over the last 4 decades (1979–2020), and they drew similar conclusions regarding the dominant role of water withdrawals in GW declines. Water withdrawals in those studies were computed based on allocated water use rights – the legal entitlements that allow users to extract water –, which is a common approach adopted in water resources assessment studies in Chile due to the lack of actual water use estimates. A caveat of this approach is that it may introduce large uncertainties as water use rights do not necessarily represent actual water uses but rather the fixed monthly or annual flows that are legally permitted for use (see Sect. 5).
Our study complements these recent findings by making use of novel estimates of water use and availability developed by the Center for Climate and Resilience Research (Alvarez-Garreton et al., 2023b), covering an extended period (1960–2020). We reflect on how the unsustainable GW use carries risks usually unforeseen or neglected, even before a D0 is reached, and we discuss how this poses an intergenerational justice dilemma. Finally, we identify key water management limitations, from general perceptions of GW to specific management instruments, that should be addressed to mitigate the impacts of GW overexploitation. While the argument is framed using Chile as a case study, the approach and conclusions can be applied to any region experiencing significant water stress and unsustainable GW usage.
The data products used here were developed by the Center for Climate and Resilience Research (Alvarez-Garreton et al., 2023b) to evaluate the long-term evolution of water availability, land use and water use across continental Chile, and they are available for exploration at https://seguridadhidrica.cr2.cl, last access: 1 October 2023). Time series of water availability were computed for each basin in Chile as the difference between catchment-scale annual precipitation and near-natural ET. Precipitation comes from the CR2MET meteorological dataset, which provides daily time series in a regular ° grid (∼5 km) for the period 1960–2020 (Boisier, 2023). The near-natural ET, with the same temporal and spatial resolutions, is estimated from a simple “bucket” water balance model. This model is driven by CR2MET meteorological inputs and a static land cover representative of the year 1950, assuming no irrigation. The model includes parameters related to the soil water holding capacity, root depth, canopy rainfall interception, maximum ratio of actual to potential evapotranspiration (akin to crop coefficients), and phenological cycle (leaf area index) across 61 natural and anthropic land cover classes.
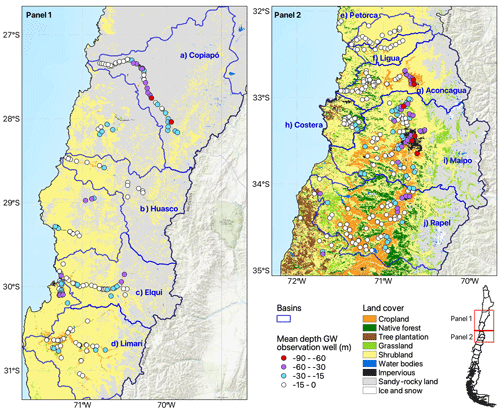
Figure 1Location of the 10 major basins in central-north Chile. The land cover corresponds to Zhao et al. (2016). The maps show the GW observation wells that have at least 10 years of observations since 1960. Each observation well is colored by their mean depth computed for their complete period of record.
The water use dataset provides annual time series for the period 1960–2020 of consumptive and non-consumptive sectorial water uses. For the LULUCF sector (Land Use, Land Use Change and Forestry), water uses were computed over the same grid and using the same ET model previously described but taking into account the changes in land cover and irrigation practices over the study period. Water consumption from industrial sectors and drinking water were estimated at the level of communal units, considering specific drivers (e.g., population, energy, or mining production) and water use coefficients, following a methodology similar to DGA (2017). Some of the key novelties of this product is that it is based on actual socioeconomic activities, including the evolution of LULUCF, industrial production and population, and that land use-related water consumption estimates are consistent with the climate data. It is important to note that these water use estimates are independent from the allocated water use rights, thereby addressing an important information gap in Chile and enabling a proper contrast of climate variability, water availability, and water uses. This, in turn, facilitates the assessment of the historical evolution of water stress and groundwater overexploitation, including an examination of its causes and consequences.
The variations in GW levels are obtained from the observation well network maintained by the water directorate, which is publicly accessible from https://snia.mop.gob.cl/BNAConsultas/reportes (last access: 1 October 2023).
To illustrate the relationship between water uses, water availability (with their corresponding WSI) and a concealed D0 situation, we focus on 10 major basins in the central-north region of Chile (Fig. 1), where a number of water conflicts have been experienced due to rising demand and reduced water availability related to climate variability (e.g., Barría et al., 2021b; Muñoz et al., 2020), and where GW overexploitation has been recently reported (Taucare et al., 2024; Duran-Llacer et al., 2020; Jódar et al., 2023). We first discuss the case of the Maipo basin, which encompasses the extensive metropolitan area of Santiago, and subsequently expand to the broader region of central-north Chile to illustrate the argument from a wider perspective.
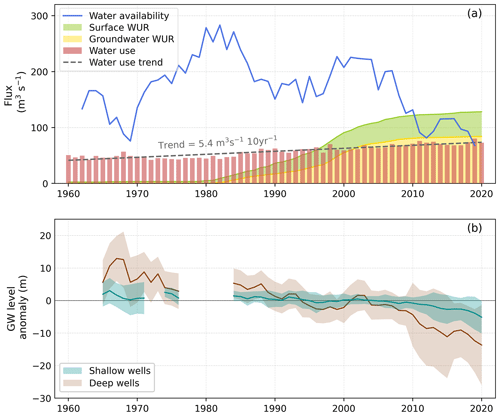
Figure 2Panel (a) shows the time series of water availability, water use rights (WURs), water uses and the linear trend of water uses for the Maipo basin. Panel (b) shows the GW level anomalies of 89 observation wells located in the Maipo basin, computed as the difference between GW levels and the mean level for 1980–2010 (baseline adopted to avoid including the megadrought period). The median (solid lines) and standard deviation (shaded area) of the GW level anomalies are plotted when at least five observations were available. Following well construction guidelines (INDAP, 2010), observation wells were classified as shallow or deep wells if their mean annual GW levels (shown in Fig. 1) were above or below 15 m, respectively.
The metropolitan area of Santiago, located in the Maipo River basin in central Chile (Fig. 1), houses nearly six million inhabitants (30 % of the Chilean population). According to the water use dataset (Sect. 2), the Maipo basin's current total water consumption is approximately 75 m3 s−1, representing 15 % of Chile's total consumptive water use. About 60 % of the basin's water consumption is attributed to the agricultural sector, while 35 % is allocated for sanitation and drinking water.
Since the mid-20th century, total water use has continuously increased and, in the last decade, has approached water availability in the Maipo basin (Fig. 2). These extreme water stress conditions (WSI close to 100 %) emerge in the context of a protracted drought spanning more than a decade since 2010, with precipitation deficits ranging between 20 % to 70 % (Garreaud et al., 2017, 2019). The so-called megadrought in central Chile is partially controlled by natural climate variability but also weighted by a long-term drying trend affecting the subtropical South Pacific region (Boisier et al., 2016, 2018). This trend, as an anthropogenic climate change signal, is expected to persist in the coming decades, in line with global climate projections (IPCC, 2022).
Yet, the causes of the extreme water stress in the Maipo basin are not solely attributable to climate variability and droughts. The water demand, driven by the expansion of irrigated agriculture, has been maintained or even increased in central Chile over recent decades, despite the diminishing water availability. To a large extent, water use in this region has been sustained at the expense of GW resources, as indicated by the increasing allocation of water use rights for GW withdrawals (Fig. 2a). Larger GW extractions and lower recharge rates are further evidenced by the ongoing depletion of GW levels (Fig. 2b).
Unlike estimating the time until a surface reservoir runs out, such as in the Cape Town and Montevideo cases, there is significant uncertainty in estimating when a GW reserve will be exhausted or reduced to a state that is unrecoverable within human time frames, leading to an absolute D0 scenario. Determining that time frame requires a precise quantification of the water volume remaining in aquifers, GW recharges, and GW extraction rates. However, a tentative estimate of the absolute D0 can be derived as
where W represents the water volume of the saturated aquifer, UGW the groundwater extraction rate, and RN the net GW recharge rate (water recharges minus springs). Previous studies have estimated a volume of water of about 30 km3 for the main aquifer in the Maipo basin (Araneda et al., 2010; DGA, 2021) with net GW recharge rates in the range of 10 to 20 m3 s−1 (Döll and Fiedler, 2008; Riffo, 2022). If we consider present water uses of 75 m3 s−1 and a ratio of underground to total water uses ranging between 35 % to 65 % (upper bound corresponds to the ratio of GW to total water use rights in the Maipo basin, Fig. 2), the absolute D0 time frame would range between 50 to 200 years, depending on the values considered for recharge and GW to total water use ratio.
These rough numbers computed from variables that are challenging to estimate and monitor provide an order of magnitude range that goes from several decades to a few centuries for the total depletion of GW sources in the Maipo basin, that is, for reaching an absolute D0 in Chile's largest city. In contrast to the short time frames associated with a D0 caused by depleted surface reservoirs in some major cities (e.g., Cape Town, Montevideo), several decades may seem like plenty of time to prepare for an absolute D0 in Chile. However, such an absolute D0 is far more critical than running out of surface reserves since GW reservoirs may take a much longer time to recover.
This situation poses an intergenerational justice dilemma as we are depleting the water savings of previous generations and spending them in short-term economic activities whose benefits may not be perceived by future generations (Hiskes, 2009). Conversely, limiting the current generation's use of GW to sustainable levels entails costs for today's society that cannot be easily offset by the benefits that this sustainable use would have in future – and potentially wealthier – generations (e.g., Andersen et al., 2020).
The use of fossil water (i.e., resources that entered the aquifers centuries or millennia ago) would be a paragon example of this intergenerational dilemma. The quantification of fossil water in arid and semi-arid Chile has not been fully addressed. Still, there is evidence indicating that a part of GW reserves in several basins derive from late glacial climate conditions (Gayo et al., 2012; Moran et al., 2019; Viguier et al., 2018). Available evidence from a headwater section of the Maipo basin (Mapocho basin) have reported deep wells (water table below 112 m depth) characterized by low tritium concentrations (<0.8) and 14C contents (70 pmc, percent modern carbon), indicating that such resources were likely recharged about 3000 years ago (Iriarte et al., 2009). These GW age tracers indicate that GW with modern and old fractions could also occur in the downstream area of the Maipo basin.
In Santiago, a recent adaptive measure taken by governmental and water supply agencies has been the construction of deep pumping wells (reaching up to 300 m depth) to supply drinking water for human consumption. While this measure is timely in the short term, helping to mitigate the risks of supply shortages in a highly populated region, it also promotes the use of GW sources that might be, at best, very difficult to recover within human timescales. This, in turn, will likely exacerbate GW depletion.
Like the Maipo basin, consumptive water uses in most basins located in central-north Chile (27–35° S, Fig. 1) are nearing or exceeding their water availability (Figs. 3 and A1). The definition of water availability entails the maximum potential of the integrated long-term freshwater flow incoming to a basin, which could potentially be much higher than the long-term GW recharge. Therefore, the intersection of water use and availability curves reveals a structural imbalance: permanent water uses rely more on depleting GW storage rather than on renewable sources. This situation will likely persist or worsen in the medium term due to the expected reduction in precipitation and GW recharge. Current climate models project a reduction of 10 % to 30 % in annual precipitation by the end of the 21st century under high (SSP1-RCP2.6) and low (SSP3-RCP7.0) greenhouse gas emission mitigation scenarios, respectively (Alvarez-Garreton et al., 2023b; IPCC, 2022). At the same time, water use will likely increase with population and economic growth (Meza et al., 2014).
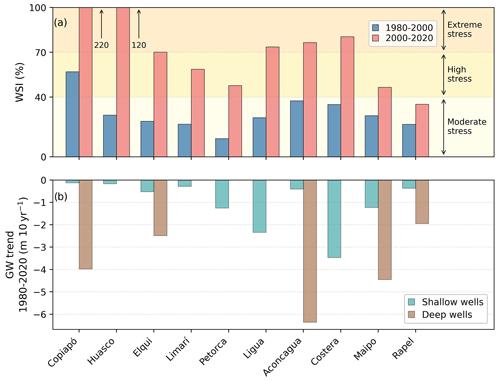
Figure 3Panel (a) shows the WSI of 10 major basins of central-north Chile, computed as water use to availability ratio for the periods 1980–2020 and 2000–2020. The basins are sorted from north to south. The two most arid ones (Copiapó and Huasco) have WSI values above the upper WSI limit, so their values are written beside the bars. The categories of water stress were obtained from Oki and Kanae (2006). Panel (b) shows the trend of GW levels of shallow and deep observation wells computed for the period 1980–2020. Note that the basins Huasco, Limarí, Petorca, Ligua, and Costera do not have enough deep well observations to compute these trends.
Except for the most arid basin (Copiapó), all basins in central-north Chile maintained a moderate level of water stress during the 1980–2000 period (Fig. 3a). However, during the 2000–2020 period, 9 out of the 10 basins moved towards a high or extreme water stress (Fig. 3a). The Rapel basin is the sole exception, maintaining a moderate stress level over the past 2 decades. This basin has the largest water availability compared to the other eight basins (Fig. A1), which allows for sustaining large water uses (similar to those of the Maipo basin) at lower water stress levels. Furthermore, the water supply in the Rapel basin relies less on GW sources, as indicated by the lower allocation of GW use rights in the basin, which would also explain its lower GW declines in Fig. 3b.
For the rest of the basins, the large WSI values displayed in Fig. 3a align with the GW declines observed over the past 4 decades (Fig. 3b) and the growing trend in the allocation of water use rights from GW sources (Fig. A1). The GW declines in this region have been reported in previous studies (Pizarro et al., 2022; Valois et al., 2020; Duran-Llacer et al., 2020; Jódar et al., 2023; Taucare et al., 2024). Within the study area, the most significant case is the mean reduction of up to 6 m per decade in the Aconcagua basin (Fig. 3b).
Despite the current water stress levels observed in Fig. 3a, the decreased water availability driven by the megadrought over the last decade has brought a relatively low direct cost to the Chilean economy (Fernández et al., 2023). This could be partly explained by the extensive use of GW in the region and the country's robust infrastructure. However, when shifting from a national to a local scale, the direct impacts of declining GW levels become more evident. For example, the need for deeper wells to reach the water table has intensified social inequalities, particularly in rural areas where reliance on shallow pumping wells is common. Here, the GW level decline has led to interruptions in the water supply for basic needs and small-scale agriculture activities, as reported by previous studies in the Petorca and Ligua basins (Duran-Llacer et al., 2020; Muñoz et al., 2020). Although this does not involve water cuts in a megacity, it represents a D0 condition for those communities.
Furthermore, environmental impacts may also emerge well before reaching an absolute D0 condition. Declining GW levels have the potential to directly impact the ecological integrity of groundwater-dependent ecosystems and may cause a disconnection between surface and GW sources (Jódar et al., 2023), potentially leading to the desiccation of rivers, wetlands, and lakes. This has been observed in the Ligua and Petorca basins (Duran-Llacer et al., 2020, 2022; Muñoz et al., 2020) as well as in the Maipo basin (Barría et al., 2021b).
Water management in Chile is primarily regulated by the Water Code, promulgated in 1981, with several amendments since then (Congreso Nacional de Chile, 1981, 2005, 2022). This code defines water as a national asset for public use and regulates its allocation by means of water use rights, which are legal entitlements specifying the quantity and timing of water that can be used by private and public users. While these titles are granted free of charge by the state, once allocated they represent property assets that can be traded by their owners, with no water market regulations to their buying and selling transactions. Further details regarding the water management system can be found in Alvarez-Garreton et al. (2023a), Taucare et al. (2024), Barría et al. (2021a) and Alvez et al. (2020).
The estimates of total water use at the present time shown in Figs. 2 and A1 indicate that for most of the study basins water uses are within the legal limits – i.e., they do not exceed the allocated water use rights. However, this evidence aggregated at the catchment scale does not imply the absence of illegal water uses within the basins. In fact, previous studies have reported unauthorized GW extraction in basins in central Chile, such as Maipo (Venegas-Quiñones, 2022), Petorca (Muñoz et al., 2020), and Ligua basins (Budds, 2008).
While not disregarding the potential occurrence of unauthorized water withdrawals at specific locations, our findings point out that the problem at the basin scale is not due to illegal overuse but rather to the current water allocation scheme being inadequate to prevent water stress conditions. To elaborate on this, we highlight some aspects outlined in the Water Code that help us to understand the causes of the current overallocation of water resources:
-
Since its amendment in 2005 (Congreso Nacional de Chile, 2005), the Water Code stipulates that the allocation of surface water use rights must consider the protection of ecological flows. Nonetheless, the specified safeguarded flow, set at a maximum of 20 % of the mean annual streamflow, is not sufficient. This implies total water usage exceeding 80 % of the water availability (i.e., WSI > 80 %), which is associated with an extreme water stress condition (Alvarez-Garreton et al., 2023a).
-
The allocation of GW use rights does not consider the interactions between the GW and surface systems, nor does it consider the pre-existing surface water use rights within a given basin.
-
Surface and GW use rights are allocated as fixed absolute flow values and do not account for long-term, climate-driven changes in water availability (Barría et al., 2021a).
-
Before the last Water Code modification in 2022, water use rights were allocated in perpetuity. After 2022, new allocations may expire after 30 years only if the central authorities demonstrate that they are not being used or are causing water scarcity problems (Congreso Nacional de Chile, 2022). This modification does not apply to water rights granted before 2022, which account for most of the water rights allocated in Chile.
The above dispositions of the Water Code entail an inadequate protection of ecological flows, a disconnected management of surface and GW resources, and the failure to account for long-term climate change. This allows for a tight balance between water uses and availability that can become a structural condition of overuse in a basin. In combination with the drier climatic conditions experienced in central-north Chile over the last decade, these water management caveats have led to unsustainable GW withdrawals (Jódar et al., 2023; Taucare et al., 2024).
These water management limitations promote water overuse, as the main signal that current users have (besides natural water scarcity by drought) is not the price of water extraction (owners of water use rights do not pay for water) but rather the amount of water provided by the water use rights they own. Despite the evident shortcomings, addressing these issues is far from straightforward. Segments of public opinion in Chile argue that revising already allocated water use rights, such as limiting their perpetuity condition or adjusting their allocated volumes considering current and future water availability, may introduce legal uncertainties that might harm the economy (Libertad y Desarrollo, 2019). The argument is that the owners of water use rights need to plan their investments and revenues based on certain water volumes. Indeed, like private property, the nature of water rights regulation allows owners to mortgage their water entitlements to obtain a loan from the bank (Muchnik et al., 1997). Regardless of the various perspectives on this matter, it is important to note that such legal certainty becomes physically unrealistic if the basin is not able to provide the amount of water stipulated by the allocated water use rights.
Climate projections for central Chile suggest that droughts such the one of the 2010 decade will become more frequent. With current extraction rates (a conservative scenario), GW levels will likely continue to decrease, causing socioeconomic and environmental impacts and bringing a major urban area such as Santiago closer to an absolute D0. The large uncertainty regarding D0 estimates as those shown here (50 to 200 years) highlights the urgent need to improve the estimations of GW volume and recharge rates and to account for its uncertainty in decision-making.
Furthermore, long-term water management plans should consider the risks of GW overexploitation in order to achieve sustainable development goals and water security. This involves revisiting the Water Code to address specific limitations, such as those highlighted here. In particular, we recommend the following:
-
Update the formulae used to compute ecological flows and remove the upper threshold of 20 % of mean annual streamflow.
-
Integrate the allocation of surface and GW use rights in such a way that the total flow granted within a basin does not exceed the water uses associated with an extreme water stress level (i.e., WSI < 70 %) while accounting for the decreased precipitation expected for Chile.
This paper argues that access to GW savings is crucial for coping with water scarcity, particularly during droughts. However, when water withdrawals are steadily greater than recharges, GW storage inevitably declines over time. The partial or complete depletion of GW has present-day detrimental impacts on society and environment, plus potential negative effects that extend beyond the time frame of this generation, concealing risks for water security that are often underestimated or disregarded. These risks are analogous to those that would exist if water uses relied on a melting glacier or a depleting surface reservoir. However, unlike surface resources, designing strategies to prevent an absolute D0 is a challenging task due to the hidden nature of GW resources. The road towards the absolute D0 poses an intergenerational justice dilemma while crossing several tipping points beyond which social, economic, and environmental impacts may become irreversible (Gleeson et al., 2020).
The capacity to access GW allows for tapping into large water volumes, often seen as an additional water source to the one available on the surface, but the maintenance of GW savings is reliant on recharge rates from the surface. Given this constraint, water consumption rates that approach or exceed freshwater availability will not be sustainable in the long term, whether the access is from GW, rivers, channels, or reservoirs.
To move towards a common perspective about the sustainable use of water resources, we recommend revisiting the definition of water availability to explicitly include the sustainable use of GW. It is crucial to stop considering GW savings, which may not be renewable within time frames of the generation that benefits from its use, as an additional and unlimited source of water. This likely implies a change in regulations and the adoption of a set of rewards and sanctions that maintain the system far from overuse tipping points (Castilla-Rho et al., 2017).
Natural water reserves in aquifers or in the form of snow and glaciers, along with artificial savings in reservoirs, primarily contribute to water availability through temporal regulation. From an infrastructure perspective, increasing water availability can also be achieved through water transfer between basins and desalination of seawater, both of which have socio-environmental benefits and costs that should be considered in their evaluation.
The sustained declines of GW reported here, as well as those reported in several regions worldwide (de Graaf et al., 2019), represent a critical situation that should trigger effective responses, just as they are triggered when the decline of a surface reservoir indicates the arrival of a D0.
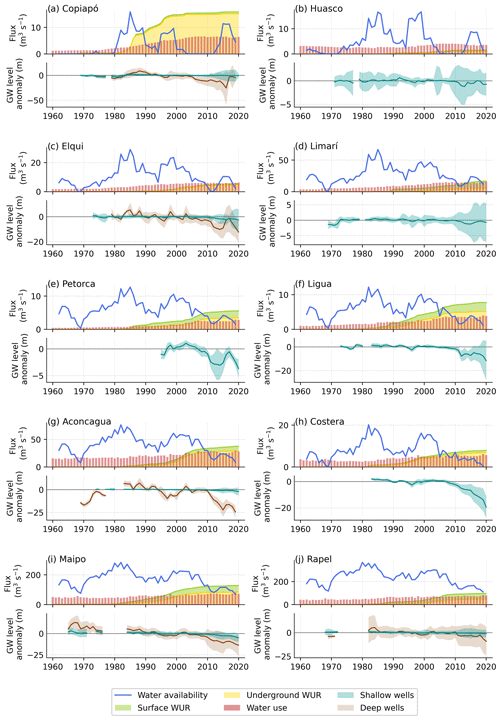
Figure A1The upper plots in each panel show the time series of water availability, water uses, and water use rights (WURs) for the 10 major basins in central-north Chile. The lower plots in each panel show the GW level anomalies of the observation wells located in each basin, computed as the difference between GW levels and the mean level for 1980–2010. The median (solid lines) and standard deviation (shaded area) of the GW level anomalies are plotted when at least five observations were available. Following well construction guidelines (INDAP, 2010), observation wells were classified as shallow and deep wells if their mean annual GW levels (shown in Fig. 1) were above or below 15 m, respectively.
The water use and availability data were obtained upon request from the Center for Climate and Resilience Research website at https://www.cr2.cl (Center for Climate and Resilience Research:, 2023). The GW levels data were obtained from the Dirección General de Aguas (DGA) website https://snia.mop.gob.cl/BNAConsultas/reportes (DGA, 2023). The water use rights were obtained from the CAMELS-CL dataset (Alvarez-Garreton et al., 2018), available at the Center for Climate and Resilience Research website at https://camels.cr2.cl (CAMELS-CL, 2023).
CAG and JPB conceived the idea, perform the analyses and wrote an early draft of the manuscript. All the authors revised early manuscript drafts and wrote the final paper.
The contact author has declared that none of the authors has any competing interests.
Publisher's note: Copernicus Publications remains neutral with regard to jurisdictional claims made in the text, published maps, institutional affiliations, or any other geographical representation in this paper. While Copernicus Publications makes every effort to include appropriate place names, the final responsibility lies with the authors.
This article is part of the special issue “Drought, society, and ecosystems (NHESS/BG/GC/HESS inter-journal SI)”. It is a result of the Panta Rhei Drought in the Anthropocene workshop 2022, Uppsala, Sweden, 29–30 August 2022.
This research has been developed within the framework of the Center for Climate and Resilience Research (CR2, ANID/FONDAP/1523A0002) and the research projects ANID/FSEQ210001 and ANID/FONDECYT/11240924.
This research has been supported by the Agencia Nacional de Investigación y Desarrollo (grant nos. ANID/FONDAP/1523A0002, ANID/FSEQ210001, and ANID/FONDECYT/11240924).
This paper was edited by Noemi Vergopolan and reviewed by two anonymous referees.
Ahmadi, M. S., Sušnik, J., Veerbeek, W., and Zevenbergen, C.: Towards a global day zero? Assessment of current and future water supply and demand in 12 rapidly developing megacities, Sustain. Cities Soc., 61, 102295, https://doi.org/10.1016/j.scs.2020.102295, 2020.
Alvarez-Garreton, C., Mendoza, P. A., Boisier, J. P., Addor, N., Galleguillos, M., Zambrano-Bigiarini, M., Lara, A., Puelma, C., Cortes, G., Garreaud, R., McPhee, J., and Ayala, A.: The CAMELS-CL dataset: catchment attributes and meteorology for large sample studies – Chile dataset, Hydrol. Earth Syst. Sci., 22, 5817–5846, https://doi.org/10.5194/hess-22-5817-2018, 2018.
Alvarez-Garreton, C., Boisier, J. P., Billi, M., Lefort, I., Marinao, R., and Barría, P.: Protecting environmental flows to achieve long-term water security, J. Environ. Manage., 328, 116914, https://doi.org/10.1016/j.jenvman.2022.116914, 2023a.
Alvarez-Garreton, C., Boisier, J. P., Blanco, G., Billi, M., Nicolas-Artero, C., Maillet, A., Aldunce, P., Urrutia-Jalabert, R., Zambrano-Bigiarini, M., Guevara, G., Galleguillos, M., Muñoz, A., Christie D., Marinao, R., and Garreaud, R.: Seguridad Hídrica en Chile: Caracterización y Perspectivas de Futuro, Santiago, https://www.cr2.cl/seguridadhidrica/ (last access: 1 December 2023), 2023b.
Alvez, A., Aitken, D., Rivera, D., Vergara, M., McIntyre, N., and Concha, F.: At the crossroads: can desalination be a suitable public policy solution to address water scarcity in Chile's mining zones?, J. Environ. Manage., 258, 110039, https://doi.org/10.1016/j.jenvman.2019.110039, 2020.
Andersen, T. M., Bhattacharya, J., and Liu, P.: Resolving intergenerational conflict over the environment under the pareto criterion, J. Environ. Econ. Manage., 100, 102290, https://doi.org/10.1016/j.jeem.2019.102290, 2020.
Araneda, M. C., Soledad Avendaño, M. R., and Díaz Del Río, G.: Modelo estructural de la cuenca de Santiago, Chile y su relación con la hidrogeología, Revista Geofísica, 62, 29–48, 2010.
Barría, P., Barria Sandoval, I., Guzman, C., Chadwick, C., Alvarez-Garreton, C., Diaz-Vasconcellos, R., Ocampo-Melgar, A., and Fuster, R.: Water allocation under climate change: A diagnosis of the Chilean system, Elementa, 9, 1–20, https://doi.org/10.1525/elementa.2020.00131, 2021a.
Barría, P., Chadwick, C., Ocampo-Melgar, A., Galleguillos, M., Garreaud, R., Díaz-Vasconcellos, R., Poblete, D., Rubio-Álvarez, E., and Poblete-Caballero, D.: Water management or megadrought: what caused the Chilean Aculeo Lake drying?, Reg. Environ. Change, 21, 19, https://doi.org/10.1007/s10113-021-01750-w, 2021b.
Bierkens, M. F. P. and Wada, Y.: Non-renewable groundwater use and groundwater depletion: A review, Environ. Res. Lett., 14, 063002, https://doi.org/10.1088/1748-9326/ab1a5f, 2019.
Boisier, J. P.: CR2MET: A high-resolution precipitation and temperature dataset for the period 1960–2021 in continental Chile (v2.5), Zenodo [data set], https://doi.org/10.5281/zenodo.7529682, 2023.
Boisier, J. P., Rondanelli, R., Garreaud, R., and Muñoz, F.: Anthropogenic and natural contributions to the Southeast Pacific precipitation decline and recent megadrought in central Chile, Geophys. Res. Lett., 43, 413–421, https://doi.org/10.1002/2015GL067265, 2016.
Boisier, J. P., Alvarez-Garretón, C., Cordero, R. R., Damiani, A., Gallardo, L., Garreaud, R. D., Lambert, F., Ramallo, C., Rojas, M., and Rondanelli, R.: Anthropogenic drying in central-southern Chile evidenced by long-term observations and climate model simulations, Elem. Sci. Anth., 6, 1–20, https://doi.org/10.1525/elementa.328, 2018.
Budds, J.: Whose Scarcity? The Hydrosocial Cycle and the Changing Waterscape of La Ligua River Basin, Chile, in: Contentious geographies: Environmental knowledge, meaning, scale, Ashgate Studies in Environmental Policy and Practice, edited by: Goodman, M. K., Boykoff, M. T., and Evered, K. T., Farnham, Surrey, 59–78; eBook ISBN 9781315573953, 2008.
Burls, N. J., Blamey, R. C., Cash, B. A., Swenson, E. T., Fahad, A., Bopape, M. J. M., Straus, D. M., and Reason, C. J. C.: The Cape Town “Day Zero” drought and Hadley cell expansion, NPJ Clim. Atmos. Sci., 2, 27, https://doi.org/10.1038/s41612-019-0084-6, 2019.
CAMELS-CL: https://camels.cr2.cl (last access: 1 October 2023), 2023.
Castilla-Rho, J. C., Rojas, R., Andersen, M. S., Holley, C., and Mariethoz, G.: Social tipping points in global groundwater management, Nat. Hum. Behav., 1, 640–649, https://doi.org/10.1038/s41562-017-0181-7, 2017.
Center for Climate and Resilience Research: https://www.cr2.cl (last access: 1 October 2023), 2023.
Congreso Nacional de Chile: Decreto Fuerza de Ley 1122, Fija Texto del Código de Aguas, Biblioteca del Congreso Nacional de Chile, 1–68, https://bcn.cl/2f8tw (last access: 1 October 2023), 1981.
Congreso Nacional de Chile: Ley 20017, Modifica el Código de Aguas, Ministerio de Obras Públicas, 1–7, https://bcn.cl/2n3tw (last access: 1 October 2023), 2005.
Congreso Nacional de Chile: Decreto con fuerza de ley 1122, Fija Texto del Código de Aguas, Ministerio de Justicia, 1–89, https://bcn.cl/2f8tw (last access: 1 October 2023), 2022.
de Graaf, I. E. M., Gleeson, T., (Rens) van Beek, L. P. H., Sutanudjaja, E. H., and Bierkens, M. F. P.: Environmental flow limits to global groundwater pumping, Nature, 574, 90–94, https://doi.org/10.1038/s41586-019-1594-4, 2019.
DGA: Estimación de la demanda actual, proyecciones futuras y caracterización de la calidad de los recursos hídricos en Chile, SIT 419, Realizado por: Unión temporal de proveedores Hídrica Consultores SPA y Aquaterra ingenieros LTDA, https://snia.mop.gob.cl/repositoriodga/handle/20.500.13000/6960 (last access: 1 October 2023), 2017.
DGA: Plan estratégico de gestión hídrica en la cuenca del Maipo, Santiago, 1–420, https://snia.mop.gob.cl/repositoriodga/handle/20.500.13000/125473 (last access: 1 October 2023), 2021.
DGA: https://snia.mop.gob.cl/BNAConsultas/reportes (last access: 1 October 2023), 2023.
Döll, P. and Fiedler, K.: Global-scale modeling of groundwater recharge, Hydrol. Earth Syst. Sci., 12, 863–885, https://doi.org/10.5194/hess-12-863-2008, 2008.
Duran-Llacer, I., Munizaga, J., Arumí, J. L., Ruybal, C., Aguayo, M., Sáez-Carrillo, K., Arriagada, L., and Rojas, O.: Lessons to be learned: Groundwater depletion in Chile's ligua and petorca watersheds through an interdisciplinary approach, Water, 12, 2446, https://doi.org/10.3390/w12092446, 2020.
Duran-Llacer, I., Arumí, J. L., Arriagada, L., Aguayo, M., Rojas, O., González-Rodríguez, L., Rodríguez-López, L., Martínez-Retureta, R., Oyarzún, R., and Singh, S. K.: A new method to map groundwater-dependent ecosystem zones in semi-arid environments: A case study in Chile, Sci. Total Environ., 816, 151528, https://doi.org/10.1016/j.scitotenv.2021.151528, 2022.
Fernández, F. J., Vásquez-Lavín, F., Ponce, R. D., Garreaud, R., Hernández, F., Link, O., Zambrano, F., and Hanemann, M.: The economics impacts of long-run droughts: Challenges, gaps, and way forward, J. Environ. Manage., 344, 118726, https://doi.org/10.1016/j.jenvman.2023.118726, 2023.
Garreaud, R., Alvarez-Garreton, C., Barichivich, J., Boisier, J. P., Christie, D., Galleguillos, M., LeQuesne, C., McPhee, J., and Zambrano-Bigiarini, M.: The 2010–2015 mega drought in Central Chile: Impacts on regional hydroclimate and vegetation, Hydrol. Earth Syst. Sci., 21, 6307–6327, https://doi.org/10.5194/hess-21-6307-2017, 2017.
Garreaud, R. D., Boisier, J. P., Rondanelli, R., Montecinos, A., Sepúlveda, H. H., and Veloso-Aguila, D.: The Central Chile Mega Drought (2010–2018): A climate dynamics perspective, Int. J. Climatol., 40, 421–439, https://doi.org/10.1002/joc.6219, 2019.
Gayo, E. M., Latorre, C., Jordan, T. E., Nester, P. L., Estay, S. A., Ojeda, K. F., and Santoro, C. M.: Late Quaternary hydrological and ecological changes in the hyperarid core of the northern Atacama Desert (∼21° S), Earth-Sci. Rev., 113, 120–140, https://doi.org/10.1016/j.earscirev.2012.04.003, 2012.
Gleeson, T., Befus, K. M., Jasechko, S., Luijendijk, E., and Cardenas, M. B.: The global volume and distribution of modern groundwater, Nat. Geosci., 9, 161–164, https://doi.org/10.1038/ngeo2590, 2016.
Gleeson, T., Cuthbert, M., Ferguson, G., and Perrone, D.: Annual Review of Earth and Planetary Sciences Global Groundwater Sustainability, Resources, and Systems in the Anthropocene, Annu. Rev. Earth Planet. Sci., 48, 431–463, https://doi.org/10.1146/annurev-earth-071719-055251, 2020.
Gudynas, E.: Montevideo is the world's first case of a capital without drinking water in the twenty-first century, Day zero, https://ripess.eu/en/montevideo-is-the-worlds-first-case-of-a-capital-without-drinking (last access: 1 October 2023), 2023.
Hiskes, R. P.: Toward a Global Consensus on Environmental Human Rights, in: The human right to a green future: Environmental rights and intergenerational justice, Cambridge University Press, 92–116, https://doi.org/10.1017/CBO9780511575396.006, 2009.
INDAP: Manual de pequeñas obras de riego en la agricultura campesina familiar, 1–231, https://bibliotecadigital.ciren.cl/handle/20.500.13082/28979 (last access: 1 October 2023), 2010.
IPCC: Climate Change 2022: Impacts, Adaptation, and Vulnerability, in: Contribution of Working Group II to the Sixth Assessment Report of the Intergovernmental Panel on Climate Change, edited by: Pörtner, H.-O. , Roberts, D. C., Tignor, M., Poloczanska, E. S., Mintenbeck, K., Alegría, A., Craig, M., Langsdorf, S., Löschke, S., Möller, V., Okem, A., and Rama, B., Cambridge University Press, 3056 pp., https://doi.org/10.1017/9781009325844, 2022.
Iriarte, S., Atenas, M., Aguirre, E., and Tore, C.: Aquifer recharge and contamination determination using environmental isotopes: Santiago basin, Chile. A study case, in: Estudios de hidrologia isotopica en America Latina 2006, Organismo Internacional de Energía Atómica, 97–112, http://www-pub.iaea.org/MTCD/publications/PDF/TE_1611s_web.pdf (last access: 1 October 2023), 2009.
Jódar, J., Urrutia, J., Herrera, C., Custodio, E., Martos-Rosillo, S., and Lambán, L. J.: The catastrophic effects of groundwater intensive exploitation and Megadrought on aquifers in Central Chile: Global change impact projections in water resources based on groundwater balance modeling, Sci. Total Environ., 914, 169651, https://doi.org/10.1016/j.scitotenv.2023.169651, 2023.
Libertad y Desarrollo: Reforma al Código de Aguas: Principales Cambios y Desafíos, Temas públicos LyD, 1533, 1–7, https://lyd.org/wp-content/uploads/2022/03/TP-1533-REFORMA-CODIGO-DE-AGUAS.pdf (last access: 1 October 2023), 2019.
Maxmen, A.: Cape Town scientists prepare for “Day Zero”: As water crisis brews, researchers plan to modify studies and prioritize public health, Nature, 554, 13–14, https://doi.org/10.1038/d41586-018-01134-x, 2018.
Meza, F. J., Vicuña, S., Jelinek, M., Bustos, E., and Bonelli, S.: Assessing water demands and coverage sensitivity to climate change in the urban and rural sectors in central Chile, J. Water Clim. Change, 5, 192–203, https://doi.org/10.2166/wcc.2014.019, 2014.
Moran, B. J., Boutt, D. F., and Munk, L. A.: Stable and Radioisotope Systematics Reveal Fossil Water as Fundamental Characteristic of Arid Orogenic-Scale Groundwater Systems, Water Resour. Res., 55, 11295–11315, https://doi.org/10.1029/2019WR026386, 2019.
Muchnik, E., LuraschI, M., and Maldini, F.: Commercialization of water rights in Chile, Naciones Unidas. División de Desarrollo Productivo y Empresarial de la CEPAL, Santiago, 27 pp., https://hdl.handle.net/11362/4697 (last access: 1 October 2023), 1997.
Muñoz, A. A., Klock-Barría, K., Alvarez-Garreton, C., Aguilera-Betti, I., González-Reyes, Á., Lastra, J. A., Chávez, R. O., Barría, P., Christie, D., Rojas-Badilla, M., and Lequesne, C.: Water crisis in petorca basin, Chile: The combined effects of a mega-drought and water management, Water, 12, 1–17, https://doi.org/10.3390/w12030648, 2020.
Oki, T. and Kanae, S.: Global Hydrological Cycles and Freshwater Resources, Science, 313, 1068–1073, 2006.
Pizarro, R., Garcia-Chevesich, P. A., McCray, J. E., Sharp, J. O., Valdés-Pineda, R., Sangüesa, C., Jaque-Becerra, D., Álvarez, P., Norambuena, S., Ibáñez, A., Vallejos, C., and Mendoza, R.: Climate Change and Overuse: Water Resource Challenges during Economic Growth in Coquimbo, Chile, Sustainability, 14, 3440, https://doi.org/10.3390/su14063440, 2022.
Riffo, D.: Actualización del modelo DGA 2000 del acuífero Maipo-Mapocho para el análisis de la influencia del cambio climático sobre la sustentabilidad futura del sistema, Universidad de Chile, https://repositorio.uchile.cl/handle/2250/186901 (last access: 1 October 2023), 2022.
Schwartz, F. W., Liu, G., and Yu, Z.: HESS Opinions: The myth of groundwater sustainability in Asia, Hydrol. Earth Syst. Sci., 24, 489–500, https://doi.org/10.5194/hess-24-489-2020, 2020.
Taucare, M., Viguier, B., Figueroa, R., and Daniele, L.: The alarming state of Central Chile's groundwater resources: A paradigmatic case of a lasting overexploitation, Sci. Total Environ., 906, 167723, https://doi.org/10.1016/j.scitotenv.2023.167723, 2024.
Valois, R., MacDonell, S., Núñez Cobo, J. H., and Maureira-Cortés, H.: Groundwater level trends and recharge event characterization using historical observed data in semi-arid Chile, Hydrolog. Sci. J., 65, 597–609, https://doi.org/10.1080/02626667.2020.1711912, 2020.
Venegas-Quiñones, A.: New method to identify illegal uses of water by using remote sensing and neural network in Laguna de Aculeo, Chile, MS thesis, University of Arizona, https://repository.arizona.edu/handle/10150/665579 (last access: 1 October 2023), 2022.
Viguier, B., Jourde, H., Yáñez, G., Lira, E. S., Leonardi, V., Moya, C. E., García-Pérez, T., Maringue, J., and Lictevout, E.: Multidisciplinary study for the assessment of the geometry, boundaries and preferential recharge zones of an overexploited aquifer in the Atacama Desert (Pampa del Tamarugal, Northern Chile), J. S. Am. Earth Sci., 86, 366–383, https://doi.org/10.1016/j.jsames.2018.05.018, 2018.
Warner, J. F. and Meissner, R.: Cape Town's “Day Zero” water crisis: A manufactured media event?, Int. J. Disast. Risk Reduct., 64, 102481, https://doi.org/10.1016/j.ijdrr.2021.102481, 2021.
Zhao, Y., Feng, D., Yu, L., Wang, X., Chen, Y., Bai, Y., Hernández, H. J., Galleguillos, M., Estades, C., Biging, G. S., Radke, J. D., and Gong, P.: Detailed dynamic land cover mapping of Chile: Accuracy improvement by integrating multi-temporal data, Remote Sens. Environ., 183, 170–185, 2016.
- Abstract
- Introduction
- A novel dataset to illustrate the case of Chile
- A concealed day zero scenario in Santiago
- The non-sustainable GW use in central-north Chile
- Caveats in water management
- Water management recommendations
- Conclusions
- Appendix A
- Data availability
- Author contributions
- Competing interests
- Disclaimer
- Special issue statement
- Acknowledgements
- Financial support
- Review statement
- References
This opinion paper reflects on the risks of overusing groundwater savings to supply permanent water use requirements. Using novel data recently developed for Chile, we reveal how groundwater is being overused, causing ecological and socioeconomic impacts and concealing a Day Zero
scenario. Our argument underscores the need for reformed water allocation rules and sustainable management, shifting from a perception of groundwater as an unlimited source to a finite and vital one.
This opinion paper reflects on the risks of overusing groundwater savings to supply permanent...
- Abstract
- Introduction
- A novel dataset to illustrate the case of Chile
- A concealed day zero scenario in Santiago
- The non-sustainable GW use in central-north Chile
- Caveats in water management
- Water management recommendations
- Conclusions
- Appendix A
- Data availability
- Author contributions
- Competing interests
- Disclaimer
- Special issue statement
- Acknowledgements
- Financial support
- Review statement
- References