the Creative Commons Attribution 4.0 License.
the Creative Commons Attribution 4.0 License.
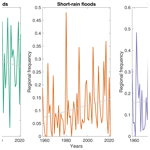
Changes in Mediterranean flood processes and seasonality
Patrick Arnaud
Guillaume Artigue
Michel Lang
Emmanuel Paquet
Luc Neppel
Eric Sauquet
Floods are a major natural hazard in the Mediterranean region, causing deaths and extensive damages. Recent studies have shown that intense rainfall events are becoming more extreme in this region but, paradoxically, without leading to an increase in the severity of floods. Consequently, it is important to understand how flood events are changing to explain this absence of trends in flood magnitude despite increased rainfall extremes. A database of 98 stations in southern France with an average record of 50 years of daily river discharge data between 1959 and 2021 was considered, together with a high-resolution reanalysis product providing precipitation and simulated soil moisture and a classification of weather patterns associated with rainfall events over France. Flood events, corresponding to an average occurrence of 1 event per year (5317 events in total), were extracted and classified into excess-rainfall, short-rainfall, and long-rainfall event types. Several flood event characteristics have been also analyzed: flood event durations, base flow contribution to floods, runoff coefficient, total and maximum event rainfall, and antecedent soil moisture. The evolution through time of these flood event characteristics and seasonality was analyzed. Results indicated that, in most basins, floods tend to occur earlier during the year, the mean flood date being, on average, advanced by 1 month between 1959–1990 and 1991–2021. This seasonal shift could be attributed to the increased frequency of southern-circulation weather types during spring and summer. An increase in total and extreme-event precipitation has been observed, associated with a decrease of antecedent soil moisture before rainfall events. The majority of flood events are associated with excess rainfall on saturated soils, but their relative proportion is decreasing over time, notably in spring, with a concurrent increased frequency of short rain floods. For most basins there is a positive correlation between antecedent soil moisture and flood event runoff coefficients that is remaining stable over time, with dryer soils producing less runoff and a lower contribution of base flow to floods. In a context of increasing aridity, this relationship is the likely cause of the absence of trends in flood magnitudes observed in this region and the change of event types. These changes in flood characteristics are quite homogeneous over the domain studied, suggesting that they are rather linked to the evolution of the regional climate than to catchment characteristics. Consequently, this study shows that even in the absence of trends, flood properties may change over time, and these changes need to be accounted for when analyzing the long-term evolution of flood hazards.
- Article
(6718 KB) - Full-text XML
-
Supplement
(827 KB) - BibTeX
- EndNote
There is a growing interest in understanding the evolution of floods occurring in different regions in the context of climate change. The recent sixth report of the Intergovernmental Panel on Climate Change (Ali et al., 2022), reported a mixture of observed trends in different Mediterranean countries, with both increasing and decreasing river floods and, overall, a low confidence in their attribution to climate change. Several large-scale studies on changes in flood risk (Slater et al., 2021a; Blöschl et al., 2017, 2019) have indicated for the Mediterranean region a possible decrease over the last decades. This difficulty in detecting changes in flood hazard is doubtlessly linked to the fact that there are different types of floods (Tarasova et al., 2019; Berghuijs et al., 2019; Stein et al., 2020; Tramblay et al., 2022). Indeed, a distinction can be made between floods associated with soil saturation excess, soil infiltration excess, or snowmelt-driven floods, and the relative frequency of these different types of floods may change over time (Zhang et al., 2022). Furthermore, these changes can occur at local to regional scales, given complex combinations of climatic and physiographic triggers, making global generalization of changes in flood risk hazardous if not irrelevant (Whitfield, 2012; Blöschl et al., 2015).
Only a few studies are focusing on changes in flood types over time; it is important to understand the long-term changes in flood processes to evaluate how flood risk can evolve, notably in order to better adapt the flood mitigation strategies (Merz et al., 2014). The main limitation to performing such studies is the need for long time series of river discharge and the need to have large samples of flood events to evaluate how their properties may change over time. For many regions of the world, the lack of observed river discharge data prevents this type of analysis. Some studies attempted to analyze the changes in different types of floods for different regions (Berghuijs et al., 2019; Mao et al., 2019; Kemter et al., 2020; Bertola et al., 2021; Liu et al., 2022; Tramblay et al., 2022; Tarasova et al., 2023). Most of these studies rely on flood classification schemes with varying degrees of complexity depending on the type of data available, allowing a data-based separation of floods into their distinct generation mechanisms (Tarasova et al., 2019; Berghuijs et al., 2019; Tarasova et al., 2020; Stein et al., 2020, 2021; Tramblay et al., 2022).
For basins under a Mediterranean climate, several studies reported complex interactions between precipitation increases and lower antecedent soil moisture, leading to threshold effects (Brunner et al., 2021a) in the catchment response to changing hydro-climatic conditions (Wasko and Nathan, 2019; Cao et al., 2020; Bertola et al., 2021). Recent large-scale studies (Jiang et al., 2022; Tarasova et al., 2023) suggested a reduction in the frequency of floods driven by soil saturation in Europe, including in basins in the Mediterranean area. Yet, these studies rely on coarse-resolution climate forcings provided by E-OBS, and there is a need to assess whether these findings are also valid in smaller basins with high-resolution datasets, notably with a better estimation of precipitation extremes. For instance, in Bertola et al. (2021), a decrease in extreme precipitation is found with E-OBS for basins in southern France, while Ribes et al. (2019) observed an opposite increasing trend using a network of about 700 rain gauges in that area. In addition, recent studies at the European scale focused on annual maxima only when, to attribute changes in flood event types, it is also relevant to consider other metrics such as the runoff coefficient and the flood event duration, which require data about flood events and not only their maxima.
In French Mediterranean basins, several studies reported an increase in precipitation extremes (Tramblay et al., 2013; Blanchet and Creutin, 2022; Ribes et al., 2019) that did not translate into increased floods (Tramblay et al., 2019). It is hypothesized that, as in many regions of the world, a decrease in soil moisture linked with a greater aridity can potentially offset the increase in precipitation extremes and thus not increase flood severity (Sharma et al., 2018; Tramblay et al., 2019; Wasko and Nathan, 2019; Wasko et al., 2021; Huang et al., 2022). Excess soil moisture was previously identified as an important flood driver, notably in the Mediterranean (Kemter et al., 2020; Bertola et al., 2021), indicating that it can play an important role. Yet, besides studies on trend detection or changes in flood types, no study has provided an in-depth analysis of the joint long-term evolution of flood processes in Mediterranean basins in relation to their drivers such as precipitation, soil moisture, and the evolution of synoptic weather patterns associated with floods. In particular, there is a need to assess how these changes may be modulated in different catchment sizes with different land use, notably in the Mediterranean context, where floods are generated by rainfall events characterized by a strong spatial and temporal variability. Therefore, the objective of the study is to evaluate how the characteristics of Mediterranean floods are changing. A recent study (Tramblay et al., 2019) indicated no significant trends in flood hazards for a large ensemble of basins located in southern France. This database is used herein and is expanded with a weather type classification linked to floods to further analyze the possible changes in flood event characteristics. This analysis provides a comprehensive view at the regional scale of the evolution in time of flood event characteristics (runoff coefficients, soil moisture, base flow contribution, etc.), flood event seasonality in relation to large-scale atmospheric patterns, flood event types (excess-rain floods, short-rain floods, etc.), and their respective magnitude.
In Sect. 2, we present the different datasets used for this analysis, including river discharge, precipitation, soil moisture data, and weather type classification. In Sect. 3, the methods are presented for event extraction, seasonality analysis, and the assessment of changes in flood drivers. Results are presented in Sect. 4.
We consider 98 catchments in southern France (Fig. 1) where the time series of daily discharge exceeds 30 years of complete data between 1959 and 2021 (Tramblay et al., 2019). Among them, 48 basins have more than 50 years of data, and the basins selected are not influenced by reservoir or dam regulation. The catchment sizes range from 14 to 3195 km2, with a mean size equal to 480 km2 (see Table S1 in the Supplement). Basins with a nival regime were removed following identification from the river discharge hydrographs and the removal of basins with more than 20 % of precipitation falling as snow. In addition to river discharge data, the precipitation and soil moisture for each basin were retrieved from the SAFRAN-ISBA-MODCOU (SIM) reanalysis covering the whole French territory at 8 km spatial resolution (Vidal et al., 2010). Precipitation and soil moisture data have been extracted and averaged at the catchment scale. The soil moisture data extracted from SIM are a soil wetness index obtained from the normalization of the volumetric soil moisture content with the wilting point and field capacity, ranging between 0 and 1. Land cover classes (forest, agricultural, urban) corresponding to 2018 have been extracted from the CORINE land cover inventory (Büttner, 2014). In addition, we used the weather type (WT) classification from Electricité de France (EDF), corresponding to a daily classification into eight synoptic situations associated with rainfall events over France (Garavaglia et al., 2010). This classification is built on geopotential heights at pressure levels of 700 and 1000 hPa, associated with rainy days over France.
3.1 Extraction of flood events
We extracted a sample of flood events with a mean occurrence of one event per year using a peaks-over-threshold approach. This type of sampling is chosen since low annual maximum runoff could be observed during dry years (Farquharson et al., 1992). A declustering algorithm is applied to identify single events to avoid introducing autocorrelation in the analysis and to ensure that flood events are independent; for this, we use the following two rules (Lang et al., 1999): first, there must be a minimum of n days between events, with n = 5 + log(catchment area); and second, between two consecutive peaks, runoff must drop below two-thirds of the smallest peak. The maximum daily runoff of each event is kept. This means that for an event lasting several days, only the maximum daily discharge and the corresponding date are kept. Then, for each flood event, we computed the total rainfall and maximum rainfall. The n-day previous precipitation is extracted. Total rainfall for each event is estimated by a cumulative sum of precipitation starting the day of the flood, and this aggregation stops if there are 2 consecutive days with precipitation close to zero (1 mm) to account for rainfall intermittency within events. The maximum daily precipitation is extracted from the same time interval used to compute total event precipitation. The soil moisture at the beginning of the events is extracted from the previous day from the start of the rainfall event. A base flow filter has been used to separate direct runoff and base flow for each time series, namely the Lyne–Hollick filter (Lyne and Hollick, 1979), with its default parameters. For each flood event, the base flow corresponding to the peak has been extracted to estimate the direct runoff, which corresponds to the event rainfall contribution, in addition to base flow. Different metrics characterizing each flood event have been computed: total rainfall (mm), event maximum rainfall (mm), duration of the rainfall event (d), duration of the flood event (d), antecedent soil moisture (0–1), and runoff coefficient (0–1). The runoff coefficient was computed for each event as the ratio of direct runoff depth and total event precipitation
3.2 Analysis of the mean date of occurrence
Circular statistics (Burn, 1997; Berens, 2009) are used to analyze flood timing. The dates are first converted into an angular value, then from this sample of angular values, the mean date of occurrence (θ) can be computed together with the concentration index (r), which measures the variability of the flood occurrences around the mean date. Using the dates of flood events, considering hydrological years starting from 1 September, θ and r are computed from the sample of dates. The first step in the analysis of seasonality is to test against circular uniformity. Circular uniformity refers to the case in which all angular values of flood dates around the circle are equally likely, indicative of the absence of flood seasonality. In that case, the computation of the mean date would have little relevance. The Rayleigh (Fisher, 1993) and Hermans–Rasson (Landler et al., 2019) tests are used to test against uniformity for unimodal distributions to verify the presence of flood seasonality (i.e., floods do not occur randomly throughout the year). To associate flood events and weather types, for each rainy day corresponding to flood events, the weather type has been extracted from the weather type's classification.
3.3 Classification of flood-generating processes
A classification is applied to the flood events, adapted from a previously implemented classification at the global scale (Stein et al., 2020), in the United States (Stein et al., 2021) and Africa (Tramblay et al., 2022). This approach relates the occurrence of rainfall amounts above various thresholds to the occurrence of floods. Flood events in each catchment are classified according to three hydrometeorological generation processes, namely, the excess rainfall, short rainfall, and long rainfall, using a decision tree. Excess rainfall is defined as a flood event triggered by rainfall that is higher than average and that occurs over wet soils (i.e., soil moisture above than 50 % saturation), short rainfall is defined as a single daily rainfall event above high thresholds (the 95th percentile computed over the whole time series of rainfall), and long rainfall is defined as several consecutive days (> 2 d) with rainfall above the 95th percentile of rainfall summed over 7 d. The classification first evaluates if a larger-than-average multi-day rainfall fell on wet soil to determine if the flood event was an excess-rainfall type of flood. If that was not the case, it evaluates whether the thresholds for long rainfall and then short rainfall are exceeded. If no process could be identified, the class of other is assigned.
3.4 Changes in flood characteristics
To assess the changes over time in flood dates and generating mechanisms, we split the records of each station into two periods of equal length. Given that most stations have records starting after the 1960s on average, the first period ranges from 1959 to 1990, and the second one ranges from 1991 to 2021, with a pivot year within ± 5 years around 1991, allowing the comparison of the two time slices across the different stations. To assess the relative changes in the flood drivers, the frequency of each driver for each time period was computed, and then we computed for each station the relative change (%) in each driver contribution (Berghuijs et al., 2019). In addition, to detect trends in the long-term frequency of event types per year, pooled at the regional scale, we used the Mann–Kendall test for trends, modified to account for autocorrelation in the time series (Hamed and Ramachandra Rao, 1998).
We use the same approach to estimate changes in the different flood event characteristics, and we applied the two-tailed Wilcoxon test to check the difference in medians. In addition, to assess the regional significance of the trends, we also employed the Mann–Kendall test for flood event characteristics pooled at the regional scale. For flood dates, we computed the mean dates of occurrence for the two time periods and assessed the significance of the difference using the Watson–Williams test, which is a circular analogue to the two-sample t test (Watson and Williams, 1956). Finally, to estimate potential relationships between different flood characteristics, the Spearman correlation coefficient (ρ) is computed.
4.1 Change in flood event characteristics
There are several changes in flood event characteristics, as seen in Fig. 2, between the two sub-periods of 1959–1990 and 1991–2021. On average, total event precipitation increases in 65 basins (66 % of basins); maximum event rainfall increases in 76 basins, which is consistent with previous studies in this area (Ribes et al., 2019; Tramblay et al., 2019; Blanchet and Creutin, 2022); on the other hand, antecedent soil moisture decreases in 71 basins; baseflow contribution decreases in 75 basins; and runoff coefficient decreases in 68 basins. These changes in soil moisture, base flow, and runoff coefficients are consistent with an overall increase of aridity in southern Europe that is mostly driven by higher evapotranspiration (Tramblay et al., 2020) and have been also observed in other regions with a similar climate (Ho et al., 2022). The number of local statistically significant changes for each flood event characteristic is given in Table 1. These numbers remain small, but it should be noted that time series are quite short for a robust statistical assessment in a context of high interannual variability. To overcome this issue, we also assessed the regional significance of these changes in flood event characteristics. We performed a regional pooling of the events and applied the Mann–Kendall test to detect trends in the regional series of event characteristics. As shown in Table 1, all the detected changes are regionally significant except for the decrease in base flow contribution to peak discharge during floods. Overall, an increase in total event rainfall can be observed, mostly caused by the increase of maximum rainfall during the events (the changes in the two variables are correlated, with ρ = 0.52), while the flood event durations decrease on average, which is consistent with studies at the global scale (Wasko et al., 2021).
These changes in precipitation are associated with a decrease of antecedent soil moisture before the beginning of the rainfall events. This decrease is also related to a smaller contribution of base flow during floods in some basins. There is indeed a significant correlation between the relative changes in the base flow contribution to peak runoff and soil moisture (ρ = 0.56), indicating that the soil moisture decrease is likely the main driver of these changes. There is also, for most basins, a significant nonlinear relationship (exponential type) between the flood event antecedent soil moisture and runoff coefficients, as reported in many studies (Penna et al., 2011; Rogger et al., 2013; Raynaud et al., 2015; Tarasova et al., 2020). Indeed, for the first time period, 1959–1990, the median Spearman correlation between antecedent soil moisture and flood runoff coefficients (see Fig. S1 in the Supplement) is equal to 0.43 and is significant at the 5 % level in 56 basins (67 basins at the 10 % significance level). For the second time period, 1991–2021, the median correlation increases to 0.45 and is significant in 64 basins at the 5 % significance level (68 at the 10 % significance level). These results show that, contrary to popular belief, at the catchment scale drier soils produce less runoff, and this characteristic is even slightly accentuated over time. Indeed, increased runoff coefficients induced either by hydrophobic soil conditions following droughts (Burch et al., 1989), soil crusting, and sealing (Bissonnais and Singer, 1993) or by compaction (Alaoui et al., 2018) are well-documented processes that mostly occur at the local plot scale and that do not produce discernible effects at the catchment scale. This observation is reinforced by the fact that no negative correlation between runoff coefficients and initial soil moisture was detected. Regarding the explanatory factors of the association between soil moisture and runoff coefficients, we found stronger correlations (significant at the 5 % level) between these two variables in catchments with a higher percentage of urban or agricultural areas, and on the other hand, we found weaker correlations with an increased percentage of forests or mean catchment altitude. There is only a very small increase in the correlations for larger basins (no significant correlation with basin sizes), indicating that this relationship between soil moisture and runoff coefficient remains valid for all basin scales considered in the present study. This relationship between runoff coefficients and antecedent soil moisture remained stable between 1959–1990 and 1991–2021 (Fig. 3).
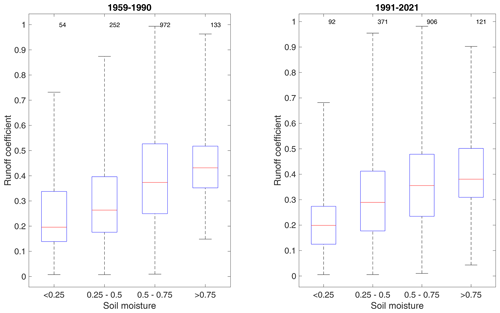
Figure 3Relationship between the flood event runoff coefficients and antecedent soil moisture for the two time periods considered: 1959–1990 and 1991–2021. For each box, the central line indicates the median, and the bottom and top edges of the box indicate the 25th and 75th percentiles, respectively. The whiskers extend to the most extreme data points. The numbers at the top of the figure indicate the number of events in each category.
4.2 Changes in flood dates
Floods in southern France tend to occur mainly during October to December for basins close to the Mediterranean and east of the Cévennes mountain range, while for basins located on the western part of the region, they tend to occur later during winter months, centered in January or February (Fig. 4). Both the Rayleigh and Hermans–Rasson tests reject the null hypothesis of uniformity at the 5 % level, indicating that floods do not occur randomly throughout the year. In most cases, the seasonal distribution is unimodal, except for a few cases; at about 15 stations, the maximum occurrence of floods is observed in late autumn or winter, and a secondary minor peak of occurrence is observed, usually centered around the month of March or April. These floods are associated with rainfall events rather than snowmelt since for only 3 basins did the snowfall contribution reach 19 % of total precipitation, whereas the snowfall contribution was much lower for the remaining 12 basins (less than 5 %).
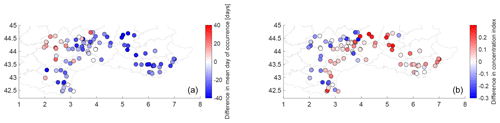
Figure 5Changes in mean flood date (a) and changes in the concentration index (b) between 1959–1990 and 1991–2021.
For 79 basins, floods tend to occur earlier during the year, on average by −22 d between 1959–1990 and 1991–2021 (Fig. 5a). On the other hand, for 19 basins, the mean flood date occurs later in the second period, with an average of +12 d. These changes in the mean date are significant in 26 basins at the 0.1 level according to the Watson–Williams test (18 basins at the 0.05 significance level). There are two differentiated spatial patterns: all basins where floods tend to occur earlier are widespread in the center of the Mediterranean region, and basins where floods tend to occur later are found only in the northwestern margin of the domain. In these basins, the mean floods occur in late winter until February and March. The same spatial patterns of changes in mean flood dates have been observed by Blöschl et al. (2017) but without a provision of the possible causes of these seasonal shifts. For the concentration index (i.e., the variability around the mean date), similarly, two different patterns are found: for basins where floods tend to occur earlier, the concentration index increases, meaning more floods are clustered around the mean date, while for western basins where floods tend to occur later, the concentration index decreases, meaning a larger variability in flood dates (Fig. 5b).
To assess the regional changes in flood dates, we first separated into two regional samples the stations where floods occur earlier (sample 1) or later (sample 2). Then we used the Watson–Williams test, previously used to assess changes in flood dates in each station, to compare these two regional samples. The test results indicate that, for the 19 stations where floods tend to occur later, the changes in flood dates are not significant at the 5 % level (p value = 0.0821); on the other hand, for the 79 stations where floods occur earlier, the change is significant (p value = 5.34 × 10−8).
4.3 Associations between flood occurrence and weather patterns
The seasonal patterns observed for the floods are closely related to the occurrence of different weather types in different sub-regions. As shown in Fig. 6, most basins located east of the Cévennes mountain range have floods associated with WT4 (southern circulation) and that western basins have floods associated with WT2 (steady oceanic). The most frequent pattern associated with 37 % of floods, WT4, is known to trigger intense rainfall events in this region (Ducrocq et al., 2008; Tramblay et al., 2013). Interestingly, the WT6 (eastern circulation) and WT7 (southwestern circulation) are both associated, to a lesser extent, with floods across the whole region but without notable spatial differences in the relative frequency of floods associated with these weather types. Changes in flood seasonality could be ascribed to changes in the seasonal occurrence of the weather types (Fig. 7): WT4 tends to occur more frequently from March to August during 1991–2021 compared to 1959–1990, and these changes are statistically significant (see Fig. S2). When looking at the actual count of WT4 days, this change represents an increase of 69 events during that 6-month period for 1991–2021 and thus an average of +2.2 d yr−1. Associated with a warmer Mediterranean Sea over the last decades, notably during summer (Pastor et al., 2020), the combination of these two factors could explain the earlier occurrence of floods east of the Cévennes mountain range. Similarly, there is an increased frequency of WT2 in January, February, and March between 1991–2021 and 1959–1990 that is also significant (Fig. S2) and that could be possibly related to the later occurrence of floods west of the Cévennes range. Although this change in weather type seasonality leading to heavy rainfalls is a plausible cause of the observed changes in the flood seasonality, more research is needed to better understand these relationships and to attribute changes in flood seasonality. Notably, this is also necessary to be able to analyze in more detail the moisture supply from the Mediterranean or Atlantic seas, the interaction with the atmospheric thermodynamics, and the duration, localization, and spatial structure of the rainfall episodes inducing floods.
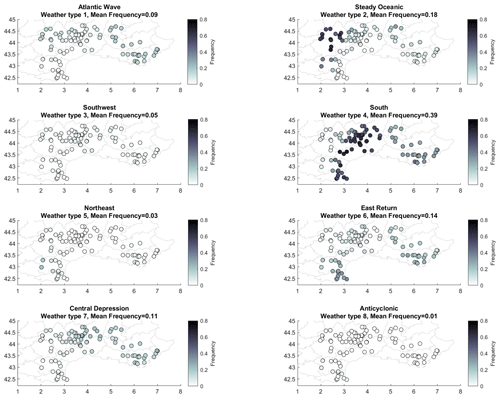
Figure 6Frequency of the different weather types associated with flood events; the names of the different weather types are from Garavaglia et al. (2010).
4.4 Changes in flood-generating processes
When first applying the classification of flood-generating processes to all floods, we find a predominance of excess-rainfall events (Fig. 8) followed by long rains and short rains, which is consistent with the known flood-generating processes in this region (Mediterranean episodes) and, in particular, the strong influence of saturated soils on runoff generation, with floods mostly occurring during the autumn (Tramblay et al., 2010, 2019). The category of other regroups less than 1 % of floods, and it represents mostly events with very low precipitation amounts, likely due to an underestimation of rainfall in the SAFRAN database for some events. It is worth noticing that, despite the large sample of basins considered, the patterns are remarkably consistent and homogeneous across different basin sizes and locations. There is a significant, yet low, correlation (ρ = 0.26, p value = 0.008) between the ratio of excess-rain floods and catchment size, with a larger proportion of excess rain in larger basins, while on the other hand, there is an even weaker and negative correlation (ρ = −0.16, p value = 0.09) between the ratio of short rain and basin size. It should be noted that floods driven by short-rain episodes potentially affect smaller regions than floods driven by excess floods (Brunner and Dougherty, 2022). For 30 basins (not necessarily the largest ones), the proportion of excess rain exceeds 80 % of the total number of flood events (see Fig. S3). For short rain and long rain, the maximum contributions observed were much lower at 36 % and 32 %, respectively, but these maximum values are only found in small basins. Indeed, instances where short rain or long rain exceed 30 % of episodes are only found in basins smaller than 100 km2 .
The mean date of occurrence is significantly different between the three flood types according to the Watson–Williams test. As shown in Fig. 9, the highest proportion of floods induced by short rain is observed during September to November, while the floods induced by long rain mostly occur during October to December, and excess-rain floods are observed in late autumn and winter, with a peak in February. This is consistent with the annual soil moisture cycle in this region: at the end of the summer, the soils are dry, and it takes several months to replenish the soil moisture level, which is at its highest during winter. If examining the long-term changes in this monthly repartition of flood types (see Fig. S4), the frequency of excess rain decreases from February to April and also in October, while it increases during winter months. This implies that the season during which excess-rain floods occur is reduced in terms of length and is concentrated during wet winter months. On the other hand, the frequency of short- or long-rain floods increases in June and September, months that are getting drier over time in this region.
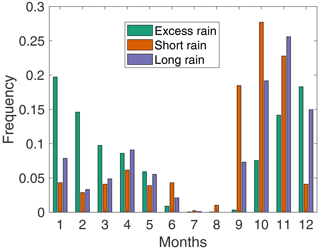
Figure 9Mean monthly frequency of occurrence for the three flood drivers: excess rain, short rain, and long rain.
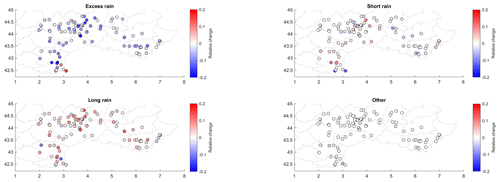
Figure 10Relative changes in the frequency of excess rain, short rain, and long rain between 1959–1990 and 1991–2021.
The noticeable changes in flood processes over time are a reduction of excess rainfall in 71 basins and an increased frequency of short rains in 53 basins and of long rains in 63 basins (Fig. 10), while short-rain and long-rain floods decrease for 19 and 22 basins, respectively. For excess rain, there are only 16 basins where its relative proportion is increasing; they are mostly located on the margins of the Alps and Pyrenees mountains. For more frequent events (i.e., if considering an average of three episodes per year instead of one), the number of basins with a change is larger, with a reduction of excess rainfall in 82 basins out of 98 (results not shown). This indicates that the soil moisture depletion has more impacts on small to moderate flood events, as previously observed by Bertola et al. (2021). There is no relationship between the rate of change in the different flood-generating processes and catchment sizes indicating a clear regional pattern. The average magnitude of these changes across all basins remains low, on average −4.1 % for excess rain, +1.2 % for short rain, and + 2.1 % long rain. Yet, the magnitude of these changes ranges from +15 % to −21 % for excess rain, +11 % to −20 % for short rain, and +12 % to −11 % for long rain, depending on the catchment, indicating that local catchment characteristics could strongly modulate the regional signal. In addition, the average values over the whole domain hide some local changes: for instance, short-rainfall floods are increasing in the southeastern part of the Cévennes while decreasing for the northwestern part, as seen in Fig. 10.
4.5 Regional changes
To assess whether the changes in the relative influence of the three different flood types are significant at the regional scale, we computed for each year the relative frequency of the different flood types for all basins together. It is indeed not possible to do this analysis for each station independently due to the small sample sizes over the two periods. These changes in the occurrence of flood types are significant at the regional scale according to the Mann–Kendall test (Fig. 11) for the frequency of excess-rain floods and short-rain floods (at the 5 % significance level) but not for the long-rain floods. With all events pooled regionally, the decrease in excess-rain floods is equal to −13 % between 1959–1990 and 1991–2021, and the increase in short-rain floods is equal to +36 %. In addition, to assess whether these results are robust in relation to the thresholds used in the classification of flood events, a Monte Carlo experiment was also conducted. Results show (see Fig. S5) that regional changes in excess-rain and short-rain floods are not dependent on classification thresholds, while this is not the case for long rain floods. In terms of flood severity for the different flood types, the median flood computed for each basin is strongly correlated to basin size (ρ = 0.78) for floods caused by excess rain, short rain (ρ = 0.80), and long rain (ρ = 0.75); and very similar results are found for the maximum flood. On the contrary, the specific discharge of flood peaks is nonlinearly related to basin sizes, with a clear threshold effect for basins smaller than 500 km2, which have a much larger specific discharge than larger basins.
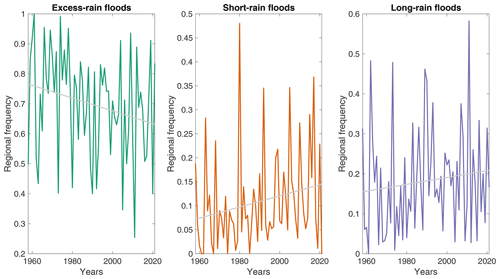
Figure 11Regional frequency of excess-rain, short-rain, and long-rain floods between 1959 and 2021. The gray lines denote a least-squares linear fit to represent the long-term tendency.
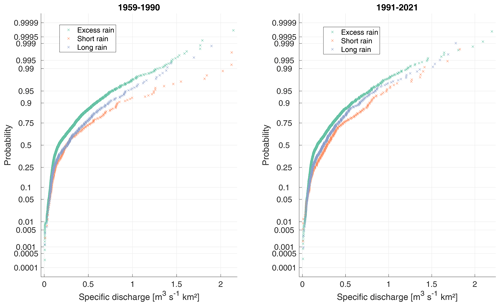
Figure 12Distribution of regionally sampled floods corresponding to excess-rain, short-rain, and long-rain types of floods for the two time periods 1959–1990 and 1991–2021.
Given that there are different flood sample sizes in the different basins corresponding to different flood-generating processes, we pooled regionally the flood events. To do so, we computed the specific discharge for each event (i.e., the flood magnitude divided by catchment area) to analyze the distributions of specific discharge for all the events associated with excess rain, long rain, or short rain. Specific discharge is used herein since it is a good indicator of flash-flood severity, notably in this Mediterranean region (Delrieu et al., 2005, Ruin et al., 2008). Figure 12 shows that the short-rain floods are more severe in terms of specific discharge than excess-rain or long-rain floods at the regional level (as also shown by Tarasova et al., 2023). The regional distributions for the different event types are different according to the Kolmogorov–Smirnov test. It must be noted that, for a given basin, the magnitude of the different types of floods may not be very different, showing the strong variability from one event to another that is not solely linked to the flood trigger. When comparing the different flood distributions between the time periods 1959–1990 and 1991–2021, the differences in flood magnitudes between excess rain, long rain, and short rain are reduced. This is mainly due to a slight decrease in the specific discharge of short-rain floods, notably for flood events with a return level higher than 10 years, while the excess-rain floods show very few changes in intensity over time.
The aim of this study was to document the evolution of the characteristics of Mediterranean floods using long river discharge records in southern France, a high-resolution climate and surface reanalysis that is used operationally to monitor water resources and extremes in France, and a classification of weather patterns. This large regional database with an average of 50 years of records allowed us to detect regional significant changes in several flood event characteristics. In most basins, floods tend to occur earlier during the year, with the mean flood date being, on average, advanced by 1 month. This seasonal shift can be attributed to the increased frequency during spring and summer of southern-circulation weather types, which are strongly associated with the occurrence of floods in this region. Over time, floods also tend to be more clustered in time over the different basins, as reflected by a decreasing variability in flood occurrence throughout the year. On the contrary, for the westernmost basins influenced by Atlantic circulation patterns, floods tend to occur later during the year, which is also due to a seasonal shift of the flood-generating circulation patterns that occur more frequently in late winter. These results indicate that changes in large-scale atmospheric circulation described by weather types are the likely driver of the seasonal shift of flood dates previously observed for French Mediterranean regions (Blöschl et al., 2017).
During floods, an increase in total and extreme-event precipitation has been observed, associated with a decrease of antecedent soil moisture before rainfall events, linked to a smaller contribution of base flow during floods. It can be concluded that it is the depletion of soil water content due to increased aridity in southern France, notably related to higher evapotranspiration rates (Tramblay et al., 2020), that is the likely cause of the absence of flood trends in this region despite the increase in extreme rainfall. It should also be noted that, over all basins, dryer soils are associated with lower runoff coefficients, and this relationship remains valid over time. This result contrasts sharply with the generally accepted idea that, in a drier climate, we observe stronger runoff. While this statement could be valid at the plot scale, we show herein that it is an opposite relationship compared to that found for the whole range of catchment sizes considered in the present work. The majority of flood events are associated with excess rainfall on saturated soils, but that proportion is decreasing over time with a concurrent increased frequency of short rain, potentially leading to more severe floods, as previously shown at the European scale by Tarasova et al. (2023). At the regional scale, we show that floods induced by short rains are indeed of higher magnitude, but due to a lower runoff coefficient induced by drier antecedent soil moisture, the specific discharge associated with short-rain floods is also decreasing over time. These results are consistent with those obtained in other regions of the world, showing that floods do not necessarily increase with the increase in extreme precipitation and that soil moisture seems to play a key role in explaining these changes and, ultimately, the lack of trends in terms of flood hazard (Wasko and Nathan, 2019; Bertola et al., 2021; Wasko et al., 2021). The results of the present study are rather homogeneous given the different catchment sizes and land use types, indicating that changes in flood type mainly result from regional climate change and not only from local changes, such as land cover or agricultural practice changes, or the increase in urban and peri-urban areas. Nonetheless, if the observed trend in increased short-rain floods persists in the upcoming decades, the severity of floods, particularly the most important ones, could increase along with the rise in rainfall extremes, particularly in areas where the soil infiltration potential is low, such as in mountainous or urbanized areas, which have expanded a lot in recent years in Mediterranean regions. For other types of basins, notably in lowland areas with agricultural or natural landscapes, caution should be exerted before extrapolating such hazards in the future since we show herein a potential reduction over time of the specific runoff, even for short-rain floods. This aspect could be further investigated using climate scenarios.
One of the main perspectives of this work would be to perform a similar analysis at sub-daily time steps, which would be more adapted to analyzing changes in flash-flood characteristics, notably in terms of the flashiness response of the catchments (Baker et al., 2004; Li et al., 2022). Indeed, the daily time step prevents a thorough analysis of changes in rainfall patterns, notably at shorter time steps. Yet, there is no gridded dataset of hourly precipitation before the 2000s in southern France that allows us to repeat such a similar study over 62 years. However, given the availability of radar rainfall over France, it would be possible to analyze, at least for the recent years after 2000, the evolution of several characteristics, such as the evolution of storm hydrographs, concentration time, and the flashiness response of the basins. Another relevant prospective work would be to analyze the spatial extent of floods. Given the future evolution of weather types associated with floods in combination with more local to regional characteristics, such as soil moisture state, these types of events may impact simultaneously wider or smaller parts of the region considered, and this could have serious implications for risk management (Brunner et al., 2021b; Brunner and Dougherty, 2022). Therefore, the joint analysis of flood occurrence in nearby basins would be highly relevant. Finally, there is also a need for new approaches to incorporate these changes in flood-generating processes into engineering practice (Slater et al., 2021b), notably to estimate the return levels for different types of infrastructure design.
The computed catchment-based indicators for each flood event in all basins are accessible from the following online repository: https://doi.org/10.5281/zenodo.8075640 (Tramblay, 2023). The complete time series of river runoff can be accesses freely here: https://hydro.eaufrance.fr/ (Ministère de la Transition Ecologique, 2023). The SAFRAN/ISBA outputs can be ordered freely for scientific purposes here: https://donneespubliques.meteofrance.fr (Meteo France, 2023).
The supplement related to this article is available online at: https://doi.org/10.5194/hess-27-2973-2023-supplement.
YT was responsible for the conceptualization, investigation, data curation, formal analysis, and preparation and writing of the original draft. YT designed the experiments, performed the analyses, and wrote the paper. PA, GA, ML, EP, LN, and ES were responsible for conceptualization, data curation, and reviewing and editing the paper.
The contact author has declared that none of the authors has any competing interests.
Publisher's note: Copernicus Publications remains neutral with regard to jurisdictional claims in published maps and institutional affiliations.
This study is a contribution to the EXPLORE2 project funded by the French Ministry of Ecological Transition. The access to the Météo France and Banque Hydro databases is acknowledged. The authors wish to thanks three anonymous reviewers and the associated editor, Manuela Brunner, for their comments.
This paper was edited by Manuela Irene Brunner and reviewed by three anonymous referees.
Alaoui, A., Rogger, M., Peth, S., and Blöschl, G.: Does soil compaction increase floods? A review, J. Hydrol., 557, 631–642, https://doi.org/10.1016/j.jhydrol.2017.12.052, 2018.
Ali, E., Cramer, W., Carnicer, J., Georgopoulou, E., Hilmi, N. J. M., Cozannet, G. L., and Lionello, P.: Mediterranean Region, Cross-Chapter Paper 4 in: Climate Change 2022: Impacts, Adaptation and Vulnerability. Contribution of Working Group II to the Sixth Assessment Report of the Intergovernmental Panel on Climate Change, edited by: Pörtner, H. O., Roberts, D. C., Tignor, M., Poloczanska, E. S., Mintenbeck, K., Alegría, A., Craig, M., Langsdorf, S., Löschke, S., Möller, V., Okem, A., and Rama, B., 2233–2272, https://doi.org/10.1017/9781009325844.021, 2022.
Baker, D. B., Richards, R. P., Loftus, T. T., and Kramer, J. W.: A new flashiness index: characteristics and applications to midwestern rivers and streams, J. Am. Water Resour. As., 40, 503–522, https://doi.org/10.1111/j.1752-1688.2004.tb01046.x, 2004.
Berens, P.: CircStat: A MATLAB Toolbox for Circular Statistics, J. Stat. Softw., 31, ), 1–21, https://doi.org/10.18637/jss.v031.i10, 2009.
Berghuijs, W. R., Harrigan, S., Molnar, P., Slater, L. J., and Kirchner, J. W.: The Relative Importance of Different Flood-Generating Mechanisms Across Europe, Water Resour. Res., 55, 4582–4593, https://doi.org/10.1029/2019WR024841, 2019.
Bertola, M., Viglione, A., Vorogushyn, S., Lun, D., Merz, B., and Blöschl, G.: Do small and large floods have the same drivers of change? A regional attribution analysis in Europe, Hydrol. Earth Syst. Sci., 25, 1347–1364, https://doi.org/10.5194/hess-25-1347-2021, 2021.
Bissonnais, Y. L. and Singer, M. J.: Seal Formation, Runoff, and Interrill Erosion from Seventeen California Soils, Soil Sci. Soc. Am. J., 57, 224–229, https://doi.org/10.2136/sssaj1993.03615995005700010039x, 1993.
Blanchet, J. and Creutin, J.-D.: Instrumental agreement and retrospective analysis of trends in precipitation extremes in the French Mediterranean Region, Environ. Res. Lett., 17, 074011, https://doi.org/10.1088/1748-9326/ac7734, 2022.
Blöschl, G., Gaál, L., Hall, J., Kiss, A., Komma, J., Nester, T., Parajka, J., Perdigão, R. A. P., Plavcová, L., Rogger, M., Salinas, J. L., and Viglione, A.: Increasing river floods: fiction or reality?, WIREs Water, 2, 329–344, https://doi.org/10.1002/wat2.1079, 2015.
Blöschl, G., Hall, J., Parajka, J., Perdigão, R. A. P., Merz, B., Arheimer, B., Aronica, G. T., Bilibashi, A., Bonacci, O., Borga, M., Čanjevac, I., Castellarin, A., Chirico, G. B., Claps, P., Fiala, K., Frolova, N., Gorbachova, L., Gül, A., Hannaford, J., Harrigan, S., Kireeva, M., Kiss, A., Kjeldsen, T. R., Kohnová, S., Koskela, J. J., Ledvinka, O., Macdonald, N., Mavrova-Guirguinova, M., Mediero, L., Merz, R., Molnar, P., Montanari, A., Murphy, C., Osuch, M., Ovcharuk, V., Radevski, I., Rogger, M., Salinas, J. L., Sauquet, E., Šraj, M., Szolgay, J., Viglione, A., Volpi, E., Wilson, D., Zaimi, K., and Živković, N.: Changing climate shifts timing of European floods, Science, 357, 588–590, https://doi.org/10.1126/science.aan2506, 2017.
Blöschl, G., Hall, J., Viglione, A., Perdigão, R. A. P., Parajka, J., Merz, B., Lun, D., Arheimer, B., Aronica, G. T., Bilibashi, A., Boháč, M., Bonacci, O., Borga, M., Čanjevac, I., Castellarin, A., Chirico, G. B., Claps, P., Frolova, N., Ganora, D., Gorbachova, L., Gül, A., Hannaford, J., Harrigan, S., Kireeva, M., Kiss, A., Kjeldsen, T. R., Kohnová, S., Koskela, J. J., Ledvinka, O., Macdonald, N., Mavrova-Guirguinova, M., Mediero, L., Merz, R., Molnar, P., Montanari, A., Murphy, C., Osuch, M., Ovcharuk, V., Radevski, I., Salinas, J. L., Sauquet, E., Šraj, M., Szolgay, J., Volpi, E., Wilson, D., Zaimi, K., and Živković, N.: Changing climate both increases and decreases European river floods, Nature, 573, 108–111, https://doi.org/10.1038/s41586-019-1495-6, 2019.
Brunner, M. I. and Dougherty, E. M.: Varying Importance of Storm Types and Antecedent Conditions for Local and Regional Floods, Water Resour. Res., 58, e2022WR033249, https://doi.org/10.1029/2022WR033249, 2022.
Brunner, M. I., Swain, D. L., Wood, R. R., Willkofer, F., Done, J. M., Gilleland, E., and Ludwig, R.: An extremeness threshold determines the regional response of floods to changes in rainfall extremes, Communications Earth & Environment, 2, 173, https://doi.org/10.1038/s43247-021-00248-x, 2021a.
Brunner, M. I., Slater, L., Tallaksen, L. M., and Clark, M.: Challenges in modeling and predicting floods and droughts: A review, WIREs Water, 8, https://doi.org/10.1002/wat2.1520, 2021b.
Burch, G. J., Moore, I. D., and Burns, J.: Soil hydrophobic effects on infiltration and catchment runoff, Hydrol. Process., 3, 211–222, https://doi.org/10.1002/hyp.3360030302, 1989.
Burn, D. H.: Catchment similarity for regional flood frequency analysis using seasonality measures, J. Hydrol., 202, 212–230, https://doi.org/10.1016/S0022-1694(97)00068-1, 1997.
Büttner, G.: CORINE Land Cover and Land Cover Change Products, in: Land Use and Land Cover Mapping in Europe, vol.+18, edited by: Manakos, I. and Braun, M., Springer Netherlands, Dordrecht, 55–74, https://doi.org/10.1007/978-94-007-7969-3_5, 2014.
Cao, Q., Gershunov, A., Shulgina, T., Ralph, F. M., Sun, N., and Lettenmaier, D. P.: Floods due to Atmospheric Rivers along the U. S. West Coast: The Role of Antecedent Soil Moisture in a Warming Climate, J. Hydrometeorol., 21, 1827–1845, https://doi.org/10.1175/JHM-D-19-0242.1, 2020.
Delrieu, G., Nicol, J., Yates, E., Kirstetter, P.-E., Creutin, J.-D., Anquetin, S., Obled, C., Saulnier, G.-M., Ducrocq, V., Gaume, E., Payrastre, O., Andrieu, H., Ayral, P.-A., Bouvier, C., Neppel, L., Livet, M., Lang, M., du-Châtelet, J. P., Walpersdorf, A., and Wobrock, W.: The Catastrophic Flash-Flood Event of 8–9 September 2002 in the Gard Region, France: A First Case Study for the Cévennes–Vivarais Mediterranean Hydrometeorological Observatory, J. Hydrometeorol., 6, 34–52, https://doi.org/10.1175/jhm-400.1, 2005.
Ducrocq, V., Nuissier, O., Ricard, D., Lebeaupin, C., and Thouvenin, T.: A numerical study of three catastrophic precipitating events over southern France. II: Mesoscale triggering and stationarity factors, Q. J. Roy. Meteor. Soc., 134, 131–145, https://doi.org/10.1002/qj.199, 2008.
Farquharson, F. A. K., Meigh, J. R., and Sutcliffe, J. V.: Regional flood frequency analysis in arid and semi-arid areas, J. Hydrol., 138, 487–501, https://doi.org/10.1016/0022-1694(92)90132-F, 1992.
Fisher, N. I.: Statistical analysis of circular data, Cambridge University Press, Cambridge, England, New York, NY, USA, 277 pp., https://doi.org/10.1017/CBO9780511564345, 1993.
Garavaglia, F., Gailhard, J., Paquet, E., Lang, M., Garçon, R., and Bernardara, P.: Introducing a rainfall compound distribution model based on weather patterns sub-sampling, Hydrol. Earth Syst. Sci., 14, 951–964, https://doi.org/10.5194/hess-14-951-2010, 2010.
Hamed, K. H. and Ramachandra Rao, A.: A modified Mann–Kendall trend test for autocorrelated data, J. Hydrol., 204, 182–196, https://doi.org/10.1016/S0022-1694(97)00125-X, 1998.
Ho, M., Nathan, R., Wasko, C., Vogel, E., and Sharma, A.: Projecting changes in flood event runoff coefficients under climate change, J. Hydrol., 615, 128689, https://doi.org/10.1016/j.jhydrol.2022.128689, 2022.
Huang, H., Fischella, M. R., Liu, Y., Ban, Z., Fayne, J. V., Li, D., Cavanaugh, K. C., and Lettenmaier, D. P.: Changes in Mechanisms and Characteristics of Western U. S. Floods Over the Last Sixty Years, Geophys. Res. Lett., 49, e2021GL097022, https://doi.org/10.1029/2021GL097022, 2022.
Jiang, S., Bevacqua, E., and Zscheischler, J.: River flooding mechanisms and their changes in Europe revealed by explainable machine learning, Hydrol. Earth Syst. Sci., 26, 6339–6359, https://doi.org/10.5194/hess-26-6339-2022, 2022.
Kemter, M., Merz, B., Marwan, N., Vorogushyn, S., and Blöschl, G.: Joint Trends in Flood Magnitudes and Spatial Extents Across Europe, Geophys. Res. Lett., 47, e2020GL087464, https://doi.org/10.1029/2020GL087464, 2020.
Landler, L., Ruxton, G. D., and Malkemper, E. P.: The Hermans–Rasson test as a powerful alternative to the Rayleigh test for circular statistics in biology, BMC Ecol., 19, 30, https://doi.org/10.1186/s12898-019-0246-8, 2019.
Lang, M., Ouarda, T. B. M. J., and Bobée, B.: Towards operational guidelines for over-threshold modeling, J. Hydrol., 225, 103–117, https://doi.org/10.1016/S0022-1694(99)00167-5, 1999.
Li, Z., Gao, S., Chen, M., Gourley, J. J., Liu, C., Prein, A. F., and Hong, Y.: The conterminous United States are projected to become more prone to flash floods in a high-end emissions scenario, Communications Earth & Environment, 3, 86, https://doi.org/10.1038/s43247-022-00409-6, 2022.
Liu, J., Feng, S., Gu, X., Zhang, Y., Beck, H. E., Zhang, J., and Yan, S.: Global changes in floods and their drivers, J. Hydrol., 614, 128553, https://doi.org/10.1016/j.jhydrol.2022.128553, 2022.
Lyne, V. D. and Hollick, M.: Stochastic time-variable rainfall runoff modelling, Hydrology and Water Resources Symposium, Institution of Engineers, Australia, Perth, 10–12 September 1979, 89–92, 1979.
Mao, Y., Zhou, T., Leung, L. R., Tesfa, T. K., Li, H., Wang, K., Tan, Z., and Getirana, A.: Flood Inundation Generation Mechanisms and Their Changes in 1953–2004 in Global Major River Basins, J. Geophys. Res.-Atmos., 124, 11672–11692, https://doi.org/10.1029/2019JD031381, 2019.
Merz, B., Aerts, J., Arnbjerg-Nielsen, K., Baldi, M., Becker, A., Bichet, A., Blöschl, G., Bouwer, L. M., Brauer, A., Cioffi, F., Delgado, J. M., Gocht, M., Guzzetti, F., Harrigan, S., Hirschboeck, K., Kilsby, C., Kron, W., Kwon, H.-H., Lall, U., Merz, R., Nissen, K., Salvatti, P., Swierczynski, T., Ulbrich, U., Viglione, A., Ward, P. J., Weiler, M., Wilhelm, B., and Nied, M.: Floods and climate: emerging perspectives for flood risk assessment and management, Nat. Hazards Earth Syst. Sci., 14, 1921–1942, https://doi.org/10.5194/nhess-14-1921-2014, 2014.
Meteo France: Données publiques, https://donneespubliques.meteofrance.fr, last access: 8 August 2023.
Ministère de la Transition Ecologique: Hydroportail, https://hydro.eaufrance.fr/, last access: 8 August 2023.
Pastor, F., Valiente, J. A., and Khodayar, S.: A Warming Mediterranean: 38 Years of Increasing Sea Surface Temperature, Remote Sens.-Basel, 12, 2687, https://doi.org/10.3390/rs12172687, 2020.
Penna, D., Tromp-van Meerveld, H. J., Gobbi, A., Borga, M., and Dalla Fontana, G.: The influence of soil moisture on threshold runoff generation processes in an alpine headwater catchment, Hydrol. Earth Syst. Sci., 15, 689–702, https://doi.org/10.5194/hess-15-689-2011, 2011.
Raynaud, D., Thielen, J., Salamon, P., Burek, P., Anquetin, S., and Alfieri, L.: A dynamic runoff co-efficient to improve flash flood early warning in Europe: evaluation on the 2013 central European floods in Germany: Dynamic runoff co-efficient for flash flood early warning in Europe, Met. Apps, 22, 410–418, https://doi.org/10.1002/met.1469, 2015.
Ribes, A., Thao, S., Vautard, R., Dubuisson, B., Somot, S., Colin, J., Planton, S., and Soubeyroux, J.-M.: Observed increase in extreme daily rainfall in the French Mediterranean, Clim. Dynam., 52, 1095–1114, https://doi.org/10.1007/s00382-018-4179-2, 2019.
Rogger, M., Viglione, A., Derx, J., and Blöschl, G.: Quantifying effects of catchments storage thresholds on step changes in the flood frequency curve: Step Changes in the Flood Frequency Curve, Water Resour. Res., 49, 6946–6958, https://doi.org/10.1002/wrcr.20553, 2013.
Ruin, I., Creutin, J.-D., Anquetin, S., and Lutoff, C.: Human exposure to flash floods – Relation between flood parameters and human vulnerability during a storm of September 2002 in Southern France, J. Hydrol., 361, 199–213, https://doi.org/10.1016/j.jhydrol.2008.07.044, 2008.
Sharma, A., Wasko, C., and Lettenmaier, D. P.: If Precipitation Extremes Are Increasing, Why Aren't Floods?, Water Resour. Res., 54, 8545–8551, https://doi.org/10.1029/2018WR023749, 2018.
Slater, L., Villarini, G., Archfield, S., Faulkner, D., Lamb, R., Khouakhi, A., and Yin, J.: Global Changes in 20-Year, 50-Year, and 100-Year River Floods, Geophys. Res. Lett., 48, e2020GL091824, https://doi.org/10.1029/2020GL091824, 2021a.
Slater, L. J., Anderson, B., Buechel, M., Dadson, S., Han, S., Harrigan, S., Kelder, T., Kowal, K., Lees, T., Matthews, T., Murphy, C., and Wilby, R. L.: Nonstationary weather and water extremes: a review of methods for their detection, attribution, and management, Hydrol. Earth Syst. Sci., 25, 3897–3935, https://doi.org/10.5194/hess-25-3897-2021, 2021b.
Stein, L., Pianosi, F., and Woods, R.: Event-based classification for global study of river flood generating processes, Hydrol. Process., 34, 1514–1529, https://doi.org/10.1002/hyp.13678, 2020.
Stein, L., Clark, M. P., Knoben, W. J. M., Pianosi, F., and Woods, R. A.: How Do Climate and Catchment Attributes Influence Flood Generating Processes? A Large-Sample Study for 671 Catchments Across the Contiguous USA, Water Res., 57, e2020WR028300, https://doi.org/10.1029/2020WR028300, 2021.
Tarasova, L., Merz, R., Kiss, A., Basso, S., Blöschl, G., Merz, B., Viglione, A., Plötner, S., Guse, B., Schumann, A., Fischer, S., Ahrens, B., Anwar, F., Bárdossy, A., Bühler, P., Haberlandt, U., Kreibich, H., Krug, A., Lun, D., Müller-Thomy, H., Pidoto, R., Primo, C., Seidel, J., Vorogushyn, S., and Wietzke, L.: Causative classification of river flood events, WIREs Water, 6, e1353, https://doi.org/10.1002/wat2.1353, 2019.
Tarasova, L., Basso, S., Wendi, D., Viglione, A., Kumar, R., and Merz, R.: A Process-Based Framework to Characterize and Classify Runoff Events: The Event Typology of Germany, Water Resour. Res., 56, e2019WR026951, https://doi.org/10.1029/2019WR026951, 2020.
Tarasova, L., Lun, D., Merz, R. Blösch, G., Basso, S., Bertola, M., Miniussi, A., Radovec, O., Samaniego, L., Thober, S., Kumar, R.: Shifts in flood generation processes exacerbate regional flood anomalies in Europe, Communications Earth & Environment, 4, 49, https://doi.org/10.1038/s43247-023-00714-8, 2023.
Tramblay, Y.: Flood event data in French Mediterranean basins, Zenodo [data set], https://doi.org/10.5281/zenodo.8075640, 2023.
Tramblay, Y., Bouvier, C., Martin, C., Didon-Lescot, J.-F., Todorovik, D., and Domergue, J.-M.: Assessment of initial soil moisture conditions for event-based rainfall–runoff modelling, J. Hydrol., 387, 176–187, https://doi.org/10.1016/j.jhydrol.2010.04.006, 2010.
Tramblay, Y., Neppel, L., Carreau, J., and Najib, K.: Non-stationary frequency analysis of heavy rainfall events in southern France, Hydrolog. Sci. J., 58, 280–294, https://doi.org/10.1080/02626667.2012.754988, 2013.
Tramblay, Y., Mimeau, L., Neppel, L., Vinet, F., and Sauquet, E.: Detection and attribution of flood trends in Mediterranean basins, Hydrol. Earth Syst. Sci., 23, 4419–4431, https://doi.org/10.5194/hess-23-4419-2019, 2019.
Tramblay, Y., Koutroulis, A., Samaniego, L., Vicente-Serrano, S. M., Volaire, F., Boone, A., Le Page, M., Llasat, M. C., Albergel, C., Burak, S., Cailleret, M., Kalin, K. C., Davi, H., Dupuy, J.-L., Greve, P., Grillakis, M., Hanich, L., Jarlan, L., Martin-StPaul, N., Martínez-Vilalta, J., Mouillot, F., Pulido-Velazquez, D., Quintana-Seguí, P., Renard, D., Turco, M., Türkeş, M., Trigo, R., Vidal, J.-P., Vilagrosa, A., Zribi, M., and Polcher, J.: Challenges for drought assessment in the Mediterranean region under future climate scenarios, Earth-Sci. Rev., 210, 103348, https://doi.org/10.1016/j.earscirev.2020.103348, 2020.
Tramblay, Y., Villarini, G., Saidi, M. E., Massari, C., and Stein, L.: Classification of flood-generating processes in Africa, Sci. Rep.-UK, 12, 18920, https://doi.org/10.1038/s41598-022-23725-5, 2022.
Vidal, J.-P., Martin, E., Franchistéguy, L., Baillon, M., Soubeyroux, J.-M.: A 50-year high-resolution atmospheric reanalysis over France with the Safran system, Int. J. Climatol., 30, 1627–1644, https://doi.org/10.1002/joc.2003, 2010.
Wasko, C. and Nathan, R.: Influence of changes in rainfall and soil moisture on trends in flooding, J. Hydrol., 575, 432–441, https://doi.org/10.1016/j.jhydrol.2019.05.054, 2019.
Wasko, C., Nathan, R., Stein, L., and O'Shea, D.: Evidence of shorter more extreme rainfalls and increased flood variability under climate change, J. Hydrol., 603, 126994, https://doi.org/10.1016/j.jhydrol.2021.126994, 2021.
Watson, G. S. and Williams, E. J.: On the Construction of Significance Tests on the Circle and the Sphere, Biometrika, 43, 344, https://doi.org/10.2307/2332913, 1956.
Whitfield, P. H.: Floods in future climates: a review: Changing floods in future climates, J.+Flood Risk Manag., 5, 336–365, https://doi.org/10.1111/j.1753-318X.2012.01150.x, 2012.
Zhang, S., Zhou, L., Zhang, L., Yang, Y., Wei, Z., Zhou, S., Yang, D., Yang, X., Wu, X., Zhang, Y., Li, X., and Dai, Y.: Reconciling disagreement on global river flood changes in a warming climate, Nat. Clim. Change, 12, 1160–1167, https://doi.org/10.1038/s41558-022-01539-7, 2022.