the Creative Commons Attribution 4.0 License.
the Creative Commons Attribution 4.0 License.
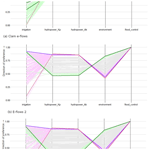
Quantifying the trade-offs in re-operating dams for the environment in the Lower Volta River
Jazmin Zatarain Salazar
Marloes Mul
Pieter van der Zaag
Jill Slinger
The construction of the Akosombo and Kpong dams in the Lower Volta River basin in Ghana changed the downstream riverine ecosystem and affected the lives of downstream communities, particularly those who lost their traditional livelihoods. In contrast to the costs borne by those in the vicinity of the river, Ghana has enjoyed vast economic benefits from the affordable hydropower, irrigation schemes and lake tourism that developed after construction of the dams. Herein lies the challenge; there exists a trade-off between water for river ecosystems and related services on the one hand and anthropogenic water demands such as hydropower or irrigation on the other. In this study, an Evolutionary Multi-Objective Direct Policy Search (EMODPS) is used to explore the multi-sectoral trade-offs that exist in the Lower Volta River basin. Three environmental flows, previously determined for the Lower Volta, are incorporated separately as environmental objectives. The results highlight the dominance of hydropower production in the Lower Volta but show that there is room for providing environmental flows under current climatic and water use conditions if the firm energy requirement from Akosombo Dam reduces by 12 % to 38 % depending on the environmental flow regime that is implemented. There is uncertainty in climate change effects on runoff in this region; however multiple scenarios are investigated. It is found that climate change leading to increased annual inflows to the Akosombo Dam reduces the trade-off between hydropower and the environment as this scenario makes more water available for users. Furthermore, climate change resulting in decreased annual inflows provides the opportunity to strategically provide dry-season environmental flows, that is, reduce flows sufficiently to meet low flow requirements for key ecosystem services such as the clam fishery. This study not only highlights the challenges in balancing anthropogenic water demands and environmental considerations in managing existing dams but also identifies opportunities for compromise in the Lower Volta River.
- Article
(3055 KB) -
Supplement
(752 KB) - BibTeX
- EndNote
Freshwater resources are under increasing pressure worldwide (WWF, 2018; He et al., 2019). As global population and standards of living have gone up, the capacity of many river basins to meet social, economic and environmental water demands has declined (Best, 2019; Fitzhugh and Richter, 2004; Postel and Richter, 2003). In the mid-20th century, many dams were built with ambitious goals for hydropower generation, flood control and irrigation among others, and dam construction has been seeing a resurgence in recent years (Grill et al., 2015; Best, 2019). This phenomenon is occurring, despite the fact that even the economic justification for many existing dams is being called into question (Ansar et al., 2014; Flyvbjerg and Bester, 2021), the life cycle emissions of some dams is above the median emissions for fossil fuel plants (Schlömer et al., 2014; Almeida et al., 2019), and the negative social and environmental impacts of dams on riparian ecosystems and communities have been established for some time (WCD, 2000; Stone, 2011; Duflo and Pande, 2007; Richter et al., 2010). Proponents of dam construction argue that in developing regions, particularly in Africa, the large energy deficit (Hafner et al., 2018), coupled with high inter-annual rainfall variability and the fact that 75 % of the population live in semi-arid or arid regions (Vörösmarty et al., 2005; Smith, 2004), makes multipurpose dams important infrastructures for energy and food security. Evidently, tools are required for investigating operation policies for managing and maximizing the benefits of dams and the water resources they control.
Multi-objective evolutionary algorithms (MOEAs) are one such tool for assessing the trade-offs between water users in a river basin. MOEAs use stochastic search tools to simultaneously find the Pareto approximate set across multiple objectives (Reed et al., 2013; Matrosov et al., 2015; Hurford et al., 2020; Zatarain Salazar et al., 2016; Kiptala et al., 2018). The Pareto approximate or non-dominated set of solutions are the suite of solutions for which increasing the water allocation to one user leads to a reduction in the benefit to others. The advantage of MOEAs is that they do not require pre-specifying preferences across objectives, thereby supporting unbiased a posteriori decision making (Reed et al., 2013; Hurford et al., 2014). Furthermore, MOEAs allow for heterogeneous and non-linear problem formulations with incommensurable objectives and different risk attitudes across objectives. Accordingly, non-market objectives can be evaluated alongside conventional economic objectives. This is particularly useful for including environmental flows (e-flows) and ecosystem services for which monetary valuation is often difficult and contested (Bingham et al., 1995; Costanza et al., 1997, 2014; Luisetti et al., 2011). The capability of MOEAs to find Pareto approximate strategies for a suite of water systems applications has been thoroughly assessed by Reed et al. (2013) and for multi-purpose reservoir operations by Zatarain Salazar et al. (2016). In this paper, an Evolutionary Multi-Objective Direct Policy Search (EMODPS) framework is applied to map the states of a system, in this case, reservoir levels and time of the year, to actions, the release of water for different water uses (Giuliani et al., 2016; Zatarain Salazar et al., 2017). This approach has been applied to find Pareto approximate operating policies for multi-objective, multi-reservoir systems (Quinn et al., 2017; Wild et al., 2019). The motivation to use EMODPS was informed by the fact that for the selected case study, multi-objective reservoir operating policies had to be found under uncertainty. Traditional approaches for optimal control, such as stochastic dynamic programming, do not permit the Pareto approximate policies across multiple objectives to be found in a single run, requiring instead that the Pareto front is constructed by testing different weights for each of the system's objectives. Such a method increases the computational burden and yields a sparse Pareto front, thereby potentially missing regions of suitable policies. The use of EMODPS overcomes this challenge by generating the trade-offs across all the system's objectives simultaneously in a single algorithmic run, creating a diverse and more accurate Pareto front (Giuliani et al., 2016). This motivates the use of direct policy search, in which radial basis functions are used to find a flexible shape to map storage levels and time to release decisions for multiple objectives.
In many of the studies where MOEAs have been applied, the e-flow objective in the simulation component of the model either meets a minimum flow release (Zatarain Salazar et al., 2017; Gonzalez et al., 2021; Kiptala et al., 2018; Hurford et al., 2020) or minimizes the deviation of flow from the natural, unregulated flow regime (Hurford and Harou, 2014). The former objective, minimum flow releases, fails to thoroughly capture the essence of e-flows which are the “quantity, timing, and quality of freshwater flows and levels required to sustain aquatic ecosystems” (The Brisbane Declaration and Global Action Agenda on Environmental Flows, 2018, cited in Arthington et al., 2018). The latter, the objective of returning fully to the natural flow regime, is an unlikely objective in many highly modified and utilized river basins (Acreman et al., 2014; Horne et al., 2017b). In this study, a multi-objective analysis of the trade-offs between key water users and the environment in the heavily modified Lower Volta River basin in Ghana is carried out. The environmental objectives include designer e-flows (Acreman et al., 2014) developed for different ecosystem services in the basin. In contrast to the aim of restoring a river to a near natural state, designer e-flows define and construct parts of the flow hydrograph of a river to meet certain desired ecological and social outcomes (Acreman et al., 2014; Horne et al., 2017a). Three e-flows, defined for the Lower Volta River in previous studies, are investigated and compared: one to support the Volta clam (Galatea paradoxa) (Owusu et al., 2022b) and the other two to support multiple ecosystem services including fisheries, aquatic weed control, flood recession agriculture and sediment transport (Mul et al., 2017). In addition, future climatic scenarios are investigated. This study highlights the challenges faced by dam operators in balancing environmental and anthropogenic water demands for existing dams in heavily modified and utilized river basins and simultaneously investigates the room for compromise in the case of the Lower Volta River under uncertainty. The main contribution of the paper is twofold: first, it explores the room for compromise in the Lower Volta by quantifying the Pareto approximate trade-offs when e-flows previously prescribed for the basin are implemented. Secondly this paper is a new application of the EMODPS under high uncertainty, where only the system goals and direction of preference are specified in the multi-objective decision problem.
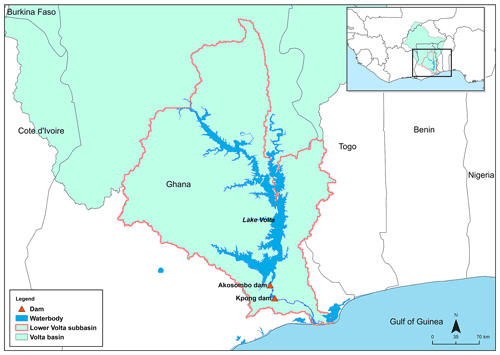
Figure 1The Akosombo and Kpong dams located in the Lower Volta River basin, which discharges into the Gulf of Guinea.
In the following section, a description of the Lower Volta River basin is given, followed by the Methods section in which (i) the simulation model for the lower Volta is described, (ii) the multi-objective evolutionary optimization setup is explained, (iii) the objective functions are formulated and (iv) relevant climate-induced effects on discharge are specified. Next is the Results section, where we present the trade-off analysis between e-flows and other water uses in the Lower Volta for the current baseline scenario and possible future scenarios. We conclude with a discussion on the implications of implementing e-flows in the Lower Volta and draw lessons for the application of EMODPS in other heavily modified basins under uncertain future conditions.
The Lower Volta River, located in Ghana, is one of four sub-basins in the Volta River basin in West Africa (Fig. 1). It is located furthest downstream, flowing into the Gulf of Guinea and covering an area of 66 700 km2, approximately 16 % of the Volta Basin. The most important hydraulic infrastructure in the Lower Volta is the Akosombo Dam, which was built in 1965 for hydropower production with an installed capacity of 1038 MW (VRA, 2021). In 1981, a smaller 160 MW run-of-the-river dam, the Kpong Dam, also began operation downstream. The lake created by the Akosombo Dam is the largest man-made lake by surface area at about 8500 km2. It has an average depth of 18.8 m and holds approximately 148 km3 of water at maximum capacity (VRA, 2021).
Construction of the Akosombo Dam led to the resettlement of over 80 000 people (Darko et al., 2019; Alhassan, 2009) and also changed the dynamic flow regime downstream from one with average low and high flows of approximately 36 m3 s−1 in March and 5100 m3 s−1 in September–October respectively, to a steady flow of about 1000 m3 s−1 per month all year round with no account taken of seasonality (Ntiamoa-Baidu et al., 2017). Consequently, the riverine ecosystem changed and so did the lives of downstream communities. Creek fishing, floodplain agriculture and the clam fishing industries, which together made up three-quarters of total real income of the Lower Volta riparian population in 1954, collapsed (Moxon, 1969; De-Graft Johnson, 1999; Tsikata, 2008; Lawson, 1972). In addition, invasive aquatic weeds proliferated, providing habitat for disease vectors including mosquitoes and snails and thereby increasing the prevalence of waterborne and water-related diseases such as malaria and schistosomiasis (Akpabey et al., 2017; Gyau-Boakye, 2001). Other environmental costs include changes to the sediment load leading to erosion along the coastline of Ghana, as well as Togo and Benin (Bollen et al., 2011; Roest, 2018; Appeaning Addo et al., 2020), as well as a reduction in salt water intrusion (Beadle, 1974; People and Rogoyska, 1969; Nyekodzi et al., 2018). Among the population in the Lower Volta, perceptions of the Akosombo Dam and the run-of-the-river Kpong Dam downstream are still overwhelmingly negative: in a survey of over 400 citizens older than 50 years in 2016, approximately 92 % considered their socio-economic conditions to be better under pre-dam natural flows (Baah-Boateng et al., 2017).
The costs borne by the river ecosystem and the communities in the vicinity of the dams in the Lower Volta are in strong contrast to the vast economic benefits that Ghana as a whole has enjoyed from the relatively affordable hydropower, irrigation schemes and tourism that developed after construction of the dams (Eshun and Amoako-Tuffour, 2016; Alhassan, 2009). After construction, the Akosombo Dam provided over 70 % of Ghana's electricity and is credited with powering Ghana's industrialization and making it one of the most developed countries in West Africa (Alhassan, 2009). The dam currently makes up about 20 % of the installed electricity generating capacity in Ghana (Dye, 2020) and is operated by the Volta River Authority (VRA).
The local–national mismatch in benefits deriving from the operation of the Akosombo Dam has been investigated in previous studies, notably in 2016 by Ntiamoa-Baidu et al. (2017). The 2016 study adopted a simulation-based approach using the Water Evaluation and Planning (WEAP) tool to compare the current flow regime with the natural flow regime and two other scenarios for re-operating the Akosombo Dam (Annor et al., 2017; Mul et al., 2017). The alternative dam operation scenarios were found to reduce power generation by 45 % to 74 % and were deemed undesirable (Annor et al., 2017). This was at a time when Akosombo and Kpong dams made up about 40 % of installed capacity and Ghana was experiencing power rationing due to low water levels in the Volta River and a shortfall in gas supply to other power plants (Dye, 2020). The present energy context of Ghana is very different and is characterized by an “overabundance” of electricity generation potential – almost twice the peak load demand and therefore a reduced dependence on power generation at Akosombo and Kpong dams (Dye, 2020; Kumi, 2017). While installed capacity does not directly translate into power delivery, it is worth re-examining the trade-offs between water users in the Lower Volta under this changed situation given that it is as a result of “take-or-pay” power purchase agreements with private power companies, whereby 90 % of the power made available by these companies has to be paid for irrespective of whether it is used or not (Dye, 2020). In 2018, the cost of this extra capacity was approximately 5 % of the country's gross domestic product (GDP) (The World Bank, 2018; Dye, 2020).
The simulation model for this study was developed using the mass balance of inflows, net evaporation rates and releases from the Akosombo Dam from 1981 to 2012 (data obtained from VRA). In addition to net evaporation and inflows, additional input data to the model consisted of downstream water levels and physical characteristics of the dam such as the storage–area–level relationships. The model was initially set up using the current baseline dam operation policy for hydropower, flood control and irrigation over a wet (2010), dry (2006) and normal (1985) year. The choice to calibrate for years with specific conditions as against the full historical time series was a practical one to expedite the calibration phase of the study. The Nash–Sutcliffe model efficiencies of 0.89, 0,91 and 0.90 respectively were obtained when the modelled and observed reservoir volumes were compared for each of the wet, dry and normal years, thus indicating a good model fit (Figs. S1–S3 in the Supplement).
Radial basis functions (RBFs) were used to parameterize the control policies for mapping reservoir levels and the time into daily release decisions (Zatarain Salazar et al., 2016, 2017). RBFs are non-linear approximating networks that allow the time-dependent operating decisions to depend on more than a single variable and most importantly can accommodate multiple objectives simultaneously (e.g. hydropower, irrigation, flood prevention and e-flows). By providing alternative Pareto approximate solutions, it is possible to visualize trade-offs between the objectives and thereby inform policy decisions. In a comparative analysis, Giuliani et al. (2016) found that RBF solutions performed better in terms of convergence, consistency and diversity of solutions as compared to another widely used universal approximator, artificial neural networks (ANNs). Indeed, using such a non-linear approximating network avoids “restricting the search for the optimal policy to a subspace of the decision space that does not include the optimal solution” (Giuliani et al., 2016).
3.1 Multi-objective problem formulation for the Lower Volta system
When the storage volume in the reservoir is known at time t, and the decision time step is set to 1, the downstream releases can be determined for the time interval [t, t+1]. The release from Akosombo Dam () is determined by the irrigation, hydropower, environmental and flood control demands ( (Eq. 1) (Fig. 2). Kpong Dam is operated as a run-of-the-river hydropower system by VRA after water is diverted for irrigation (Eq. 2).
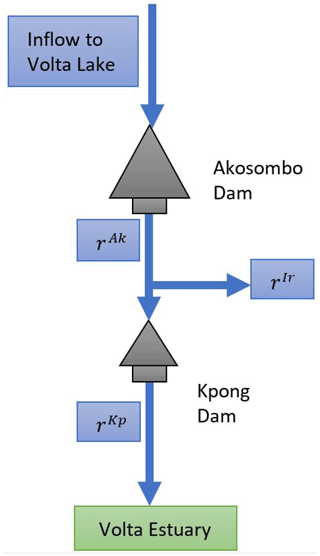
Figure 2Topology of reservoir system in the Lower Volta. rAk, rKp and rIr are the flow releases from Akosombo, from Kpong and for irrigation respectively.
The release from Kpong Dam () is therefore calculated as the difference between the release from Akosombo Dam and irrigation () and represents the downstream releases for hydropower, e-flows and floods ().
The operating policy is commonly parameterized as a function of the reservoir storage volume at a particular time. The parameterized operating policy f is then defined as a mapping between the decisions ut and the policy inputs zt comprising the time t and system state, or storage volume, xt, Eq. (3), namely
The kth decision variable in the vector ut (with k=1, …, n) is therefore defined as a weighted sum of radial basis functions, as specified in Eq. (4):
where N is the number of radial basis functions φi(⋅), and wi,k is the non-negative weight of the ith radial basis function, and the N weights sum to unity. The ith Gaussian radial basis function is then given by Eq. (5):
where j=1, …, M is the number of input variables, zt is the policy input (e.g. time t, reservoir level xt), and cj,i and bj,i are the centres and radii respectively of the ith Gaussian radial basis function for the jth input variable. The parameter vector θ is defined as with i=1, …, N; j=1, …, M; k=1, …, n, where the centre and radius are normalized with and . The policy parameters θ are determined by simulating the system over the time horizon H under the policy , …, H−1}. In this way the inputs to the RBF policy (time index and reservoir storage volume) are mapped to the outputs (release decisions). The policy parameters are evaluated by solving the multi-objective problem function, f, specified in Eq. (6) in the objective space using an informed search algorithm θ*. The objective functions J are the operating objectives of the reservoir as defined in Eqs. (7) to (12) with any maximization objectives multiplied by −1 to reformulate all the objectives as minimizations.
The number of RBFs used in this study was four (i.e. n=4). Thus, the total number of parameters (θ) for the control policy in this study is 24. A daily decision time step, representative of “real operations” of the two dams by VRA, was used (Annor et al., 2017). The simulation time horizon, H, of 29 years using historical data starting from January 1984 was constrained by the availability of data. This period however encompasses key dry and wet periods. In 1997–2000 and 2006–2007, there was power rationing in Ghana as water levels in the Akosombo reservoir fell to 73.01 m (July 1999) and 72.16 m (August 2006) respectively, both lower than the minimum operating level of 73.15 m (VRA, 2021). On the other hand, in 1991 and 2010, extremely high inflows caused the reservoir water level to rise to 83.90 and 84.42 m, respectively, close to the maximum operating level of 84.73 m, and necessitated the opening of the spillways. The 2010 reservoir level remains the highest point ever recorded at Akosombo. The four objectives considered in this study are described in more detail below.
-
Annual hydropower. The annual hydropower generated at Akosombo and Kpong dams is maximized as defined in Eq. (7). While the annual firm energy requirement from Akosombo Dam is 4415 GWh yr−1, the amount of electricity generated has typically exceeded this target in the past due to high national dependence on power generation from this dam. As such, operations at Akosombo have generally been to maximize power considering the reservoir volume and inflows to the dam (Annor et al., 2017). There is no firm energy target at Kpong, which is a run-of the river dam and generates power with releases from Akosombo after the diversion for irrigation.
where energy production (HPt) in GW is given by Eq. (8):
where t∈I denotes the days in a year, η is the turbine efficiency (dimensionless), g is acceleration due to gravity (9.81 m s−2), ρw is water density (1000 kg m−3), ht is net hydraulic head (m) and is flow through the turbines (m3 s−1). The hydropower objective at Akosombo is subject to constraints on the minimum daily firm energy requirement of 6 GWh d−1 for system stability and the maximum possible power production due to turbine capacity (1603 m3 s−1) at the maximum safe operating level of 84.73 m.
-
Irrigation. The volumetric reliability of water supply is maximized to meet irrigation demand as described in Eq. (9).
subject to the constraint in Eq. (10):
where Vt, qt and Qt are the irrigation demand, the diverted water and the flow at diversion point at time t, a day within the simulation horizon H. The current irrigation demand is 10 m3 s−1, but there have been plans since 2009 for this to be increased to 38 m3 s−1 for the Accra Plain Irrigation Project and the expansion of the Kpong left bank irrigation project (GIDA, 2009). These projects are yet to be fully realized however, and in this study the anticipated irrigation demand of 38 m3 s−1 is used as the baseline value.
-
Flood control. Flood occurrences are minimized, defined by the average number of days where downstream flow releases from Kpong, , exceed 2300 m3 s−1, the bank full capacity of the river (QF) (Eq. 11). Opening of the spillways of the Akosombo and Kpong dams is quite rare and has occurred only twice, in 1991 and 2010. Consequently the riparian communities are ill-prepared for flood releases and incur high losses whenever floods are released (Ayivor and Ofori, 2017).
-
E-flows. The trade-off between the three objectives defined above and three different e-flows (Fig. 3) is investigated in separate runs in this study. As such, three different configurations of the trade-off problem are investigated.
- a.
Clam e-flows (Fig. 3a). This e-flow was designed for the Lower Volta River using the Volta clam, a stenotopic, freshwater bivalve, as an indicator species (Owusu et al., 2022b). The recommended flow is a low flow range of 50–330 m3 s−1 from November to March to support the clam's veliger larvae stage, a key life stage in its lifecycle. An 80 % reliability of this flow occurring in the stipulated months is an acceptable compromise for the survival of the clam veliger larvae (Owusu et al., 2022b). While only a low flow is prescribed for 5 months in this e-flow recommendation, this necessarily implies that flow releases at other times of the year will be higher, although the magnitude, duration and timing of this particular flow is not defined and thus does not form a constraint for clam e-flows. The historical minimum for high flows in September and October under pre-dam flows was 1052 m3 s−1.
- b.
Natural flow dynamics considering bank full flows (e-flows 2) (Fig. 3b). This e-flow reinstates natural flow dynamics in the Lower Volta to support multiple ecosystem services including fisheries, aquatic weed control, flood recession agriculture and sediment transport (Mul et al., 2017). The minimum discharge for the high flow period in September to October is 2300 m3 s−1 (which is the bank full flow rate) to ensure that river overtopping and thus some minimum flooding of pre-dam floodplains occurs. The maximum dry-season discharge for the rest of the year is 700 m3 s−1.
- c.
Natural flow dynamics considering future dry-season irrigation (e-flow 3) (Fig. 3c). This e-flow also reinstates natural flow dynamics of the Lower Volta while providing water for future dry-season irrigation demands (Mul et al., 2017). The minimum high flow in September and October is 3000 m3 s−1, which inundates an area of approximately 156 km2, to support creek fishing and flood recession agriculture. The maximum dry-season flow rate is 500 m3 s−1.
The three alternative e-flow objectives (clam e-flows, e-flows 2 and e-flows 3) were modelled as a maximization of the reliability of the recommended flow rates occurring (Eq. 12):
where nE is number of days when downstream flow falls outside the e-flow range and nT is the total number of days when the recommended e-flows are required.
- a.
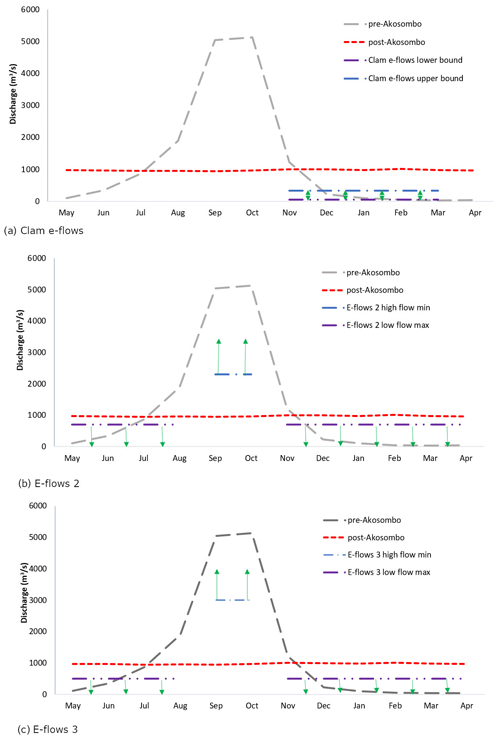
Figure 3E-flow configurations considered in this study with pre-dam (natural) and current post-dam flow regime in the Lower Volta provided for comparison (using monthly average flow data from Volta River Authority, Ghana) for a hydrological year which starts in May in the Lower Volta. For clam e-flows, the green arrows show the range of low flows recommended from November to March. For e-flows 2 and 3, the prescribed flow for September and October is a minimum threshold, while for the other 10 months, this flow is a maximum threshold. The green arrows begin at these thresholds and point in the direction of where the flows should be per the e-flow recommendation.
3.2 Future climate scenarios
In addition to a baseline scenario optimizing the trade-offs between hydropower, irrigation, flooding and e-flows under the present climate, future scenarios representing different climate futures were analysed. The recent Sixth Assessment Report of Working Group I of the Intergovernmental Panel on Climate Change (IPCC) projects that mean temperature in West Africa will increase by 1.5 ∘C by 2040 and projects with high confidence that monsoon rainfall over West Africa will increase in the middle (2041–2060) to long term (2081–2100) but have a delayed start (IPCC, 2021). Future projections made with medium confidence relate to the delayed retreat of the monsoon rains and an increase in the frequency and duration of droughts in the latter part of the 21st century (IPCC, 2021).
Table 1Design of future scenarios encompassing climate-induced changes in the Lower Volta discharge.
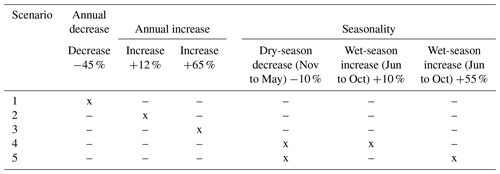
These latest climate projections draw a mixed picture of future climate in the West African subregion. A further review of the anticipated impacts of climate change specifically on runoff in the Lower Volta was carried out with the goal of identifying studies that focussed on the entire Volta basin, either as a whole or all sub-basins. A search was conducted in Scopus using the search string: TITLE-ABS-KEY (Volta AND climate AND (change OR impact), AND (flow OR discharge OR water) AND (availability OR resources)). From the 60 papers returned, a review by Roudier et al. (2014) on climate change impacts on runoff in West African rivers provided the first point of reference. From this review by Roudier et al. (2014), four studies meeting the search criteria were identified. An additional four papers from the Scopus search results, not reviewed by Roudier et al. (2014), were also retained for analysis.
Four papers (Kunstmann and Jung, 2005; Aerts et al., 2006; Jung et al., 2012; Abubakari, 2021) found that annual runoff in the Volta will increase (by 4 % to 65 %), and of these papers, three (with the exclusion of Aerts et al., 2006) also presented monthly trends which generally showed an increase in wet-season flow from June to October and a decrease in dry-season flow. The findings on only monthly trends of Jin et al. (2018) are also in line with these predictions. McCartney et al. (2012) and Sood et al. (2013), in contrast, find that there will be a decrease in annual runoff (ranging from −13 % to −45 %), while Amisigo et al. (2015) find that the results across the various scenarios are inconsistent. Table S1 in the Supplement provides further details on the papers, the climate change scenarios considered and the models used. It is important to note that using a combination of models, i.e. global and regional climatic models and then hydrological models, introduces uncertainty in the findings of climate projections for runoff (McCartney et al., 2012), and the wide-ranging results from the reviewed papers show that particularly in the case of the Volta, the direction of change in runoff is still unclear.
The climate–runoff studies, just as the latest IPCC report, present a mixed picture for the Lower Volta. Therefore, bearing the high level of uncertainty in mind, five scenarios indicative of the range of climate-induced changes predicted for the Volta discharge for the middle to long term are investigated (Table 1). These include both increases and decreases in annual runoff as well as seasonal variations in runoff into the Lake Volta.
The relationship between different water users in the Lower Volta is presented using parallel axis plots (Figs. 4 and 5). Every line crossing the axes is a Pareto approximate (non-dominated) solution and shows the performance of each water user under an alternative dam operation policy for the Lower Volta system. The range of values for each water user has been normalized using its maximum and minimum values, with the best performance featuring at the top of each axis. For irrigation and the environmental objectives, the highest value is interpreted as a dam operation policy, whereby 100 % of irrigation demand and e-flows are provided, while for the flood control objective, this is interpreted as there being no downstream flow releases above the flooding flow threshold of 2300 m3 s−1 over the simulation horizon. For hydropower at Akosombo and Kpong dams, the maximum and minimum values used in the normalization encompass the maximum and minimum annual hydropower generated across all the scenarios considered: at Akosombo Dam these are 5100 and 845 GWh yr−1 respectively and at Kpong Dam, 1000 and 130 GWh yr−1 respectively. The trade-offs between the three e-flow objectives and other water users are shown with the “best” or highest-performing operation policy for each objective highlighted. Additionally, room for compromise, characterized in this study as operation policies meeting the firm hydropower demand of 4415 GWh yr−1 for Akosombo Dam (“fair hydropower”) and e-flow demands at least 80 % of the time (“fair environment”), has also been highlighted. It is important to note that the terms “best” and “fair” as used here are not qualifying adjectives but solution descriptors in the Pareto approximate space, with the latter used to denote “reasonable” or “satisfactory” rather than “equitable” solutions.
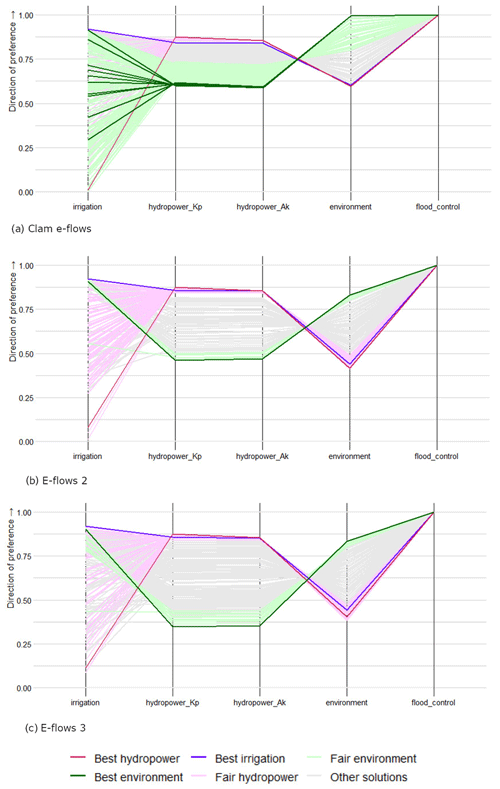
Figure 4Full set of non-dominated solutions in the baseline scenario with the “best” solution for each water user and “fair” solutions for hydropower and environment highlighted. The objective values for each water user have been normalized using the minimum and maximum values over all simulations, with the highest performance for each objective placed at the top of the axes. The order in which water users are presented has been chosen to highlight trade-offs between them.
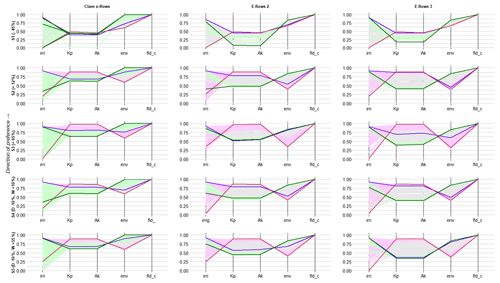
Figure 5Full set of non-dominated solutions in the future scenarios, with the “best” solution for each water user and “fair” solutions for hydropower and environment highlighted. The objective values for each water user have been normalized using the minimum and maximum values over all simulations, with the highest performance for each objective placed at the top of the axes. The order in which water users are presented has been chosen to accentuate the trade-offs. Notation of water users is as follows: Irri – irrigation, Kp – hydropower from Kpong Dam, Ak – hydropower from Akosombo Dam, env – environment (clam e-flows, e-flows 2 or e-flows 3), fld_c – flood control. Notation of scenarios is as follows: S1 to S5 – Scenario 1 to 5, D – dry-season flow, W – wet-season flow.
4.1 Baseline scenario
For the baseline scenario (Fig. 4), the highest performing dam operation policies for hydropower trade-off sharply with the provision of e-flows (all configurations) in the Lower Volta such that there is no overlap, even among fair solutions for either objective for all e-flow configurations considered in this study. To meet the current firm energy requirement of 4415 GWh yr−1 at Akosombo, e-flows can only be released about 60 %, 49 % and 47 % of the required time for the clam e-flows, e-flows 2 and e-flows 3, respectively. From the environmental perspective, the results show that hydropower demand from Akosombo and Kpong has to fall to approximately 3903 and 760 GWh yr−1 respectively for the release of clam e-flows, or approximately 3000 and 563 GWh yr−1 for the release of e-flows 2, or alternatively approximately 2711 and 508 GWh yr−1 for the release of e-flows 3 to become possible 80 % of the recommended time under current climatic conditions. The solutions for clam e-flows generally lead to higher hydropower generation compared to the other e-flows because for 7 months of the year there is no constraint on water releases in the Lower Volta for the environment; hence hydropower generation can be maximized in these months. Comparing the dynamic e-flow configurations, e-flows 2 yields higher hydropower generation because its dry-season flow recommendation is higher at 700 m3 s−1 as compared to that of e-flow 3 which is 500 m3 s−1, thus allowing for higher power generation in the dry season.
Considering the relatively low water demand for irrigation as compared to hydropower, marginal increases in hydropower generation at Kpong lead to significant reduction in the amount of irrigation demand that is met in the baseline scenario. The solutions for all e-flow configurations perform well for the flood control objective even though e-flow 2 and 3 prescribe flood releases for 2 months of the year. As such, comparing clam e-flows to e-flows 2 and 3, there is a reduction (0.99 for clam e-flows vs. 0.83 for e-flows 2 and 3) in the performance of the “best environment” solution for the latter two, as expected, showing that the requirement for floods for 2 months in a year in those e-flow configurations is not met.
4.2 Future scenarios
The effects of different climate futures on the Pareto approximate solutions for the Volta basin are presented in Fig. 5. Scenario 1, where there is 45 % decrease in annual inflows to Akosombo Dam, stands out as the system becomes water stressed so that the best-performing operation policies even for hydropower lead to only about 2776 and 550 GWh annual power generation at Akosombo and Kpong respectively for all e-flow configurations. The best operating policies for the environment however improve slightly from 0.99 to 1 for clam e-flows and remain unchanged at 0.83 for e-flows 2 and 3 relative to the baseline. This is because these solutions, even in the baseline scenario are those for which only dry-season low flows are released. In contrast to Scenario 1, under Scenario 3 and to a lesser extent Scenario 2, where annual flows to the Akosombo Dam increase by 65 % and 12 % respectively, the solutions move up on the two hydropower axes, and some fair solutions for the environment lead to higher annual hydropower production of up to 4242, 3392 and 2926 GWh yr−1 for clam e-flows, e-flows 2 and e-flows 3 respectively at Akosombo.
Seasonal climate change effects on the Lower Volta system under Scenario 4 and 5 are comparable to annual climate change effects. As a result, the solutions for Scenario 4 are similar to Scenario 2, while those for Scenario 5 are similar to Scenario 3 save for the slightly lower hydropower generation values for Scenario 5 and hence fewer “fair hydropower” solutions in line with its relatively lower inflows (+65% annual inflows for Scenario 3 vs. 55 % wet-season inflows for Scenario 5). This is due to the high residence time of water in the Lake Volta (3.9 years) and the fact that the Lower Volta has highly seasonal inflows naturally so that an annual inflow increase applied to inflows across the year, as applied in Scenario 2 and 3, results in a minimal increase in the absolute values of inflows to the dam in the dry season but a relatively significant increase in the absolute values of wet-season inflows, thus amplifying seasonality.
The Lower Volta River system is characterized by the dominance of hydropower generation for Ghana and its neighbouring countries. This has come at a high cost to downstream ecosystem services and communities (Tsikata, 2008; Lawson, 1972; Ntiamoa-Baidu et al., 2017). The results from this study show that likewise, some cost to hydropower production would have to be borne for e-flow implementation and the restoration of some of these ecosystem services under current climatic conditions. For the implementation of clam e-flows 80 % of the time, i.e. a fair environmental solution, the country would forfeit at least 11.6 % of the firm annual power demand from Akosombo Dam. For the implementation of only the dry-season flow recommendations of e-flows 2 and 3, about 32 % and 38 % respectively of current firm energy requirement would have to be supplemented with power generation from other sources. The release of floods as recommended in the dynamic e-flow configurations (e-flows 2 and 3) is not a Pareto approximate operation policy within the current operating constraints of the Akosombo Dam because in addition to flooding pre-dam floodplains which are now permanently inhabited (Ayivor and Ofori, 2017), releasing flows above the maximum turbine capacity of 1603 m3 s−1 at Akosombo means that these water volumes are lost to power generation. Flood releases also far exceed irrigation water demands.
While the majority of climate predictions for the Volta River generally point to an increase in annual water availability (Kunstmann and Jung, 2005; Aerts et al., 2006; Jung et al., 2012; Abubakari, 2021; Jin et al., 2018; Sylla et al., 2018), based on this study, an argument can be made that both an increase or a decrease in inflows to the Lower Volta enhance the potential for e-flow implementation compared to the current baseline. On the one hand, an increase in inflows to the Akosombo Dam as applied in Scenario 3 reduces the amount of the firm energy requirement that would have to be supplemented by other sources for the implementation of “fair environmental solutions” to about 3.9 % (vs. 11.6 %) for clam e-flows and then 23.2 % (vs. 32 %) for e-flows 2 and 33.7 % (vs. 38 %) for e-flows 3. On the other hand, a decrease in inflows to the Akosombo Dam, whereby at best only 2774 GWh yr−1 of hydropower can be generated, provides opportunity to strategically release recommended dry-season e-flows to reap some environmental benefits out of a “bad” situation where annual flow releases from the dam will be low anyway. This operation policy under dry climate scenarios could also be adopted in dry years, in essence modelling the Episodic E-flows Implementation approach, which is an opportunistic approach to dam re-operation that takes advantage of prevailing hydrological conditions (Warner et al., 2014; Yang and Yang, 2014; Owusu et al., 2021). This contrasts with the alternative approaches, Adaptive Management and Blanket Operation, which represent more structural inclusion of e-flows in the dam operation policy (Warner et al., 2014).
Only future climate scenarios were modelled in this study; however, inferences can also be made on the effect of simple energy and water demand futures on the Lower Volta system. For instance, an increase in irrigation demand will trade-off against hydropower production at Kpong Dam, and an increase in the firm energy requirement or the continuation of the de facto policy of hydropower maximization at Akosombo Dam, despite the availability of alternative power generation sources (Dye, 2020; Kumi, 2017), will weaken the potential for re-operation of the dam for the riverine environment. Changes in upstream water consumption as well as the construction of new dams such as the Pwalugu Dam in northern Ghana will also affect inflows to the Akosombo Dam. Gonzalez et al. (2021), however, show that practical coordination of the operation of major infrastructure in the Volta Basin, as compared to the current approach whereby dam operators fail to consider downstream built infrastructure, reduces the impact on inflows to the Akosombo Dam in particular and also maximizes basin-wide benefits. Undoubtedly this coordination should extend beyond the Volta Basin to include the entire electricity generation portfolio of Ghana and neighbouring countries to further reduce the impact of e-flow implementation in the Lower Volta on power supply.
Expanding on the current electricity generation portfolio of Ghana, the contribution of other renewable energy sources besides hydropower to the power mix has remained under 1 % since 2000, despite an on-grid target of 10 % by 2020 (now extended to 2030) (Acheampong et al., 2021; Energy Commission Ghana, 2022). The alternative sources of electricity in Ghana use carbon fuels for thermal power generation, accounting for approximately 65 % of the electricity generation portfolio in 2020 (Dye, 2020; Acheampong et al., 2021; Energy Commission Ghana, 2022). It is expected that these alternative carbon-based power sources contribute more to climate change compared to power generation from Akosombo and Kpong dams due to the fact greenhouse gas emissions from hydropower dams is negatively correlated with dam age, and even the more recent dam, Kpong, has been in operation for over 35 years (dos Santos et al., 2006; Barros et al., 2011). As such, dam re-operation in Ghana may have the long-term environmental and economic consequences of higher greenhouse gases emissions if it results in a higher reliance on the existing carbon-based power generation options, rather than other renewables like solar and wind power. Furthermore, in Ghana, hydropower has traditionally been a cheaper source of electricity compared to fossil-fuel-based power generation, and as Ghana has increased its reliance on the latter, electricity generation costs have increased resulting in higher tariffs for consumers (Energy Commission Ghana, 2022; Public Utilities Regulatory Commission, 2015). Finally, any reduction in hydropower production from Akosombo and Kpong dams due to re-operation may result in reduced overall electricity supply in Ghana as experienced during periods of drought in the past (Dye, 2020). It is estimated that the negative economic impacts of power shortages and load shedding, such as decreased productivity in industries, loss of revenue for businesses and increased costs for backup power sources, led to a GDP reduction of about 1.8 %–2 % during the 2014–2016 power crisis (Acheampong et al., 2021). Considering these potential adverse impacts of dam re-operation, it is recommended that future studies encompass a deeper analysis of the energy landscape of Ghana and investigate carbon emissions and the path to greener energy in the country, as well as energy pricing and economic implications.
By exploring the room for compromise in the Lower Volta with respect to e-flow implementation this research has taken a first step towards a comprehensive assessment of the trade-offs involved at a national and local level. The potential re-operation of the Akosombo and Kpong dams can also benefit from (i) the groundwork laid by research on the pre- and post-dam river system (Lawson, 1972; Tsikata, 2008; De-Graft Johnson, 1999; Nyekodzi et al., 2018; Obirikorang et al., 2013; Adjei-Boateng et al., 2012; Owusu et al., 2022b), (ii) insights deriving from interviews and extensive stakeholder engagement (Ayivor and Ofori, 2017; Ohemeng et al., 2017; Nukpezah et al., 2017), and (iii) existing supporting legislation for e-flow implementation (L.I. 1692 Water Use Regulations, 2001). Indeed, research on successful and stalled cases of dam re-operation indicates that stakeholder engagement and supporting legislation enhance the chances of successful e-flow implementation (Owusu et al., 2021, 2022a).
Finally, the successful application of the EMODPS framework in exploring trade-offs inherent to e-flow implementation in a heavily modified river under uncertainty holds promise for similar applications elsewhere. In order to find a policy for multiple objectives in such cases, a flexible structure to map states to actions is needed. With traditional control optimization techniques, the uncertainties need to be modelled explicitly, which creates a high computational burden and limits the ability to evaluate a large set of uncertainties (Giuliani et al., 2016). EMODPS overcomes this challenge by directly conditioning the decisions to exogenous information without requiring an explicit probabilistic model. With EMODPS, only the goals and direction of preference are required in setting up the multi-objective decision problem, making the use of this method feasible, even in data-scarce conditions. This study therefore concurs with Herman et al. (2020), who argue that direct policy search methods are a promising technique to enable adaptivity in water resources assessment by allowing the flexible integration of new information about the system into management decision making.
A dam is designed with future uses in mind – this provides the justification for its construction. The future, however, can turn out differently from that envisaged in the dam design. Therefore, re-operation of the dam to meet changing demands is a likely necessity. This study investigates current and future trade-offs between water users in the Lower Volta River basin and specifically explores the potential to deliver environmental flows to support various ecosystem services that have been negatively impacted by the current operation of the Akosombo and Kpong dams. The results highlight the dominance of hydropower production in the Lower Volta; if this relaxes there is more opportunity for restoration of the riverine environment under current climate and water use conditions and even more so under future scenarios where inflows to the Lake Volta increase. In future scenarios whereby inflows to the Lake Volta decrease, it is still possible to strategically manage and time water releases to provide dry-season low flows which will support the clam fishery and help control aquatic weeds and some waterborne diseases in the Lower Volta. This study applied advanced optimization techniques to identify and analyse dam operation policies for e-flows under discrete climatic scenarios. Future studies should focus on the robustness and limits of these policies under multitudinous future climatic and water use scenarios. Such robustness studies, together with flow experimentation, will reveal dam operation policies that may be adopted with some confidence presently. It will also build on groundwork already laid, through e-flows legislation and extensive collaborative scientific studies, for the successful re-operation of the Akosombo and Kpong dams for the environment and other water users.
The hydrological and hydraulic data associated with this paper, specifically, historical water levels, dam releases and storage–area equations for the Akosombo and Kpong dams, will be made available upon consultation with the national organization, Volta River Authority (VRA), that owns the data. Requests for these data may be made to the corresponding author.
The model code for the running the Evolutionary Multi-Objective Direct Policy Search for the Akosombo and Kpong dams is available on Zenodo at https://doi.org/10.5281/zenodo.7947905 (Owusu et al., 2023).
The supplement related to this article is available online at: https://doi.org/10.5194/hess-27-2001-2023-supplement.
AO: conceptualization, methodology, data collection, formal analysis, interpretation and writing (original draft preparation). JZS: methodology, formal analysis and writing (review and editing). MM: conceptualization, data collection, writing (review and editing), supervision and project administration. PvdZ: conceptualization, writing (review and editing), supervision and funding acquisition. JS: conceptualization, methodology, writing (review and editing), supervision and funding acquisition.
At least one of the (co-)authors is a member of the editorial board of Hydrology and Earth Systems Sciences. The peer-review process was guided by an independent editor, and the authors also have no other competing interests to declare.
Publisher's note: Copernicus Publications remains neutral with regard to jurisdictional claims in published maps and institutional affiliations.
This research is part of the EuroFLOW project (EUROpean training and research network for environmental FLOW management in river basins).
Jazmin Zatarain Salazar and Jill Slinger are supported by the Multi-Actor Systems Research Programme of TU Delft.
This research has been supported by European Union's Horizon 2020 Research and Innovation Programme under the Marie Skłodowska-Curie Actions (grant no. 765553).
This paper was edited by Yi He and reviewed by Kevis Pachos and one anonymous referee.
Abubakari, S.: Assessing impacts of climate change on hydrology in data-scarce Volta River Basin using downscaled reanalysis data, Int. J. Hydrol. Sci. Technol., 12, 176–201, https://doi.org/10.1504/IJHST.2021.116667, 2021.
Acheampong, T., Menyeh, B. O., and Agbevivi, D. E.: Ghana's Changing Electricity Supply Mix and Tariff Pricing Regime: Implications for the Energy Trilemma, Oil, Gas & Energy Law, 9, 1–21, 2021.
Acreman, M., Arthington, A. H., Colloff, M. J., Couch, C., Crossman, N. D., Dyer, F., Overton, I., Pollino, C. A., Stewardson, M. J., and Young, W.: Environmental flows for natural, hybrid, and novel riverine ecosystems in a changing world, Front. Ecol. Environ., 12, 466–473, https://doi.org/10.1890/130134, 2014.
Adjei-Boateng, D., Agbo, N. W., Agbeko, N. A., Obirikorang, K. A., and Amisah, S.: The Current State of the Clam, Galatea paradoxa, Fishery at the Lower Volta River, Ghana, in: IIFET 2012 Tanzania Proceedings, 1–12 July 2012, Dar es Salaam, Tanzania, https://doi.org/10.13140/2.1.1966.6883, 2012.
Aerts, J. C. J. H., Renssen, H., Ward, P. J., De Moel, H., Odada, E., Bouwer, L. M., and Goosse, H.: Sensitivity of global river discharges under Holocene and future climate conditions, Geophys. Res. Lett., 33, 19401, https://doi.org/10.1029/2006GL027493, 2006.
Akpabey, F. J., Addico, G., and Amegbe, G.: Flow Requirements for Aquatic Biodiversity and Aquatic Weeds, in: Dams, development and downstream communities: implications for re-optimising the operations of the Akosombo and Kpong Dams in Ghana, edited by: Ntiamoa-Baidu, Y., Ampomah, B. Y., and Ofosu, E. A., Digibooks Ghana Ltd, Tema, Ghana, 97–116, ISBN 978-9988-2-5046-1, 2017.
Alhassan, H. S.: Viewpoint – Butterflies vs. Hydropower: Reflections on large dams in contemporary Africa, Water Alternat., 2, 148–160, 2009.
Almeida, R. M., Shi, Q., Gomes-Selman, J. M., Wu, X., Xue, Y., Angarita, H., Barros, N., Forsberg, B. R., García-Villacorta, R., Hamilton, S. K., Melack, J. M., Montoya, M., Perez, G., Sethi, S. A., Gomes, C. P., and Flecker, A. S.: Reducing greenhouse gas emissions of Amazon hydropower with strategic dam planning, Nat. Commun., 10, 1–9, https://doi.org/10.1038/s41467-019-12179-5, 2019.
Amisigo, B. A., McCluskey, A., and Swanson, R.: Modeling impact of climate change on water resources and agriculture demand in the Volta Basin and other basin systems in Ghana, Sustainability, 7, 6957–6975, https://doi.org/10.3390/su7066957, 2015.
Annor, F. O., Boateng-Gyimah, M., Mul, M., Padi, P., Adwubi, A., Darkwa, K., and Addo, C.: Trade-offs between hydropower production and downstream flow requirements, in: Dams, Development and Downstream Communities. Implications for re-optimising the operations of Akosombo and Kpong dams in Ghana, edited by: Ntiamoa-Baidu, Y., Ampomah, B. Y., and Ofosu, E. A., Digibooks Ghana Ltd, Tema, Ghana, 211–230, ISBN 978-9988-2-5046-1, 2017.
Ansar, A., Flyvbjerg, B., Budzier, A., and Lunn, D.: Should we build more large dams? The actual costs of hydropower megaproject development, Energy Policy, 69, 43–56, https://doi.org/10.1016/J.ENPOL.2013.10.069, 2014.
Appeaning Addo, K., Brempong, E. K., and Jayson-Quashigah, P. N.: Assessment of the dynamics of the Volta river estuary shorelines in Ghana, Geoenviron. Disast., 7, 19, https://doi.org/10.1186/s40677-020-00151-1, 2020.
Arthington, A. H., Bhaduri, A., Bunn, S. E., Jackson, S. E., Tharme, R. E., Tickner, D., Young, B., Acreman, M., Baker, N., Capon, S., Horne, A. C., Kendy, E., McClain, M. E., Poff, N. L. R., Richter, B. D., and Ward, S.: The Brisbane Declaration and Global Action Agenda on environmental flows (2018), Front. Environ. Sci., 6, 45, https://doi.org/10.3389/fenvs.2018.00045, 2018.
Ayivor, J. S. and Ofori, B. D.: Impacts of Hydrological Changes of the Volta River on Local Livelihoods: Lessons for Re-Operation and Re-Optimisation of the Akosombo And Kpong Dams, in: Dams, development and downstream communities: implications for re-optimising the operations of the Akosombo and Kpong Dams in Ghana, edited by: Ntiamoa-Baidu, Y., Ampomah, B. Y., and Ofosu, E. A., Digibooks Ghana Ltd, Tema, Ghana, 63–93, ISBN 978-9988-2-5046-1, 2017.
Baah-Boateng, W., Twum-Barimah, R., Sawyerr, L. M., and Ntiamoa-Baidu, Y.: Perceptions of the Effects of Re-Operation of The Akosombo and Kpong Dams on the Livelihoods of Downstream Communities, in: Dams, development and downstream communities: implications for re-optimising the operations of the Akosombo and Kpong Dams in Ghana, edited by: Ntiamoa-Baidu, Y., Ampomah, B. Y., and Ofosu, E. A., Tema, Ghana, 233–256, ISBN 978-9988-2-5046-1, 2017.
Barros, N., Cole, J. J., Tranvik, L. J., Prairie, Y. T., Bastviken, D., Huszar, V. L. M., Del Giorgio, P., and Roland, F.: Carbon emission from hydroelectric reservoirs linked to reservoir age and latitude, Nat. Geosci., 4, 593–596, https://doi.org/10.1038/ngeo1211, 2011.
Beadle, L. C.: The inland waters of tropical Africa. An introduction to tropical limnology, Longman Group, London, 365 pp., ISBN 13:978-0582448520, ISBN 10:0582448522, 1974.
Best, J.: Anthropogenic stresses on the world's big rivers, Nat. Geosci., 12, 7–21, https://doi.org/10.1038/s41561-018-0262-x, 2019.
Bingham, G., Bishop, R., Brody, M., Bromley, D., Clark, E., Cooper, W., Costanza, R., Hale, T., Hayden, G., Kellert, S., Norgaard, R., Norton, B., Payne, J., Russell, C., and Suter, G.: Issues in ecosystem valuation: improving information for decision making, Ecol. Econ., 14, 73–90, https://doi.org/10.1016/0921-8009(95)00021-Z, 1995.
Bollen, M., Trouw, K., Lerouge, F., Gruwez, V., Bolle, A., Hoffman, B., Leysen, G., De Kesel, Y., and Mercelis, P.: Design of a Coastal Protection Scheme for Ada at the Volta River Mouth (Ghana), Coast. En. Proc., 1, 36–48, https://doi.org/10.9753/icce.v32.management.36, 2011.
Costanza, R., D'Arge, R., De Groot, R., Farber, S., Grasso, M., Hannon, B., Limburg, K., Naeem, S., O'Neill, R. V., Paruelo, J., Raskin, R. G., Sutton, P., and Van Den Belt, M.: The value of the world's ecosystem services and natural capital, Nature, 387, 253–260, https://doi.org/10.1038/387253a0, 1997.
Costanza, R., de Groot, R., Sutton, P., van der Ploeg, S., Anderson, S. J., Kubiszewski, I., Farber, S., and Turner, R. K.: Changes in the global value of ecosystem services, Global Environ. Change, 26, 152–158, https://doi.org/10.1016/J.GLOENVCHA.2014.04.002, 2014.
Darko, D., Kpessa-Whyte, M., Obuobie, E., Siakwah, P., Torto, O., and Tsikata, D.: The Context and Politics of Decision Making on Large Dams in Ghana: An Overview, FutureDAMS Working Paper No. 002, SSRN, https://ssrn.com/abstract=3793481 (last access: 18 May 2023), 2019.
De-Graft Johnson, K. K.: Overview of the weed problems in the Volta basin, in: The sustainable integrated development of the Volta Basin in Ghana, edited by: Gordon, C. and Amatekpor, J., Volta Basin Research Project, University of Ghana, Legon, Accra, iii, 159 pp., ISBN 9988-0-0237-8, 1999.
dos Santos, M. A., Rosa, L. P., Sikar, B., Sikar, E., and dos Santos, E. O.: Gross greenhouse gas fluxes from hydro-power reservoir compared to thermo-power plants, Energy Policy, 34, 481–488, https://doi.org/10.1016/J.ENPOL.2004.06.015, 2006.
Duflo, E. and Pande, R.: Dams, Quart. J. Econ., 122, 601–646, https://doi.org/10.1162/qjec.122.2.601, 2007.
Dye, B. J.: Structural reform and the politics of electricity crises in Ghana: tidying whilst the house is on fire?, FutureDAMS Working Paper no. 013, Manchester, https://doi.org/10.2139/ssrn.401126, 2020.
Energy Commission Ghana: 2022 National Energy Statistics, Accra, https://energycom.gov.gh/files/2022 Energy Statistics.pdf (last access: 18 May 2023), 2022.
Eshun, M. E. and Amoako-Tuffour, J.: A review of the trends in Ghana's power sector, Energ. Sustain. Soc., 6, 1–9, https://doi.org/10.1186/s13705-016-0075-y, 2016.
Fitzhugh, T. W. and Richter, B. D.: Quenching urban thrist: Growing cities and their impact on freshwater ecosystems, Bioscience, 54, 741–754, https://doi.org/10.1641/0006-3568(2004)054[0741:QUTGCA]2.0.CO;2, 2004.
Flyvbjerg, B. and Bester, D. W.: The Cost-Benefit Fallacy: Why Cost-Benefit Analysis Is Broken and How to Fix It, J. Benefit Cost. Anal., 12, 395–419, https://doi.org/10.1017/BCA.2021.9, 2021.
GIDA: Detailed feasibility study of Accra Plains Irrigation project, 200,000 ha, Final, Accra, 431 pp., https://openjicareport.jica.go.jp/pdf/12085874_01.pdf (last access: 18 May 2023), 2009.
Giuliani, M., Castelletti, A., Pianosi, F., Mason, E., and Reed, P. M.: Curses, Tradeoffs, and Scalable Management: Advancing Evolutionary Multiobjective Direct Policy Search to Improve Water Reservoir Operations, J. Water Resour. Pl. Manage., 142, 04015050, https://doi.org/10.1061/(asce)wr.1943-5452.0000570, 2016.
Gonzalez, J. M., Matrosov, E. S., Obuobie, E., Mul, M., Pettinotti, L., Gebrechorkos, S. H., Sheffield, J., Bottacin-Busolin, A., Dalton, J., Smith, D. M., and Harou, J. J.: Quantifying Cooperation Benefits for New Dams in Transboundary Water Systems Without Formal Operating Rules, Front. Environ. Sci., 9, 107, https://doi.org/10.3389/fenvs.2021.596612, 2021.
Grill, G., Lehner, B., Lumsdon, A. E., Macdonald, G. K., Zarfl, C., and Reidy Liermann, C.: An index-based framework for assessing patterns and trends in river fragmentation and flow regulation by global dams at multiple scales, Environ. Res. Lett., 10, 015001, https://doi.org/10.1088/1748-9326/10/1/015001, 2015.
Gyau-Boakye, P.: Environmental Impacts of the Akosombo Dam and Effects of Climate Change on the Lake Levels, Environ. Dev. Sustain., 3, 17–29, https://doi.org/10.1023/A:1011402116047, 2001.
Hafner, M., Tagliapietra, S., and De Strasser, L.: Energy in Africa: Challenges and Opportunities, Springer Nature Switzerland AG, Cham, Switzerland, 125 pp., ISBN 978-3-319-92218-8, 2018.
He, F., Zarfl, C., Bremerich, V., David, J. N. W., Hogan, Z., Kalinkat, G., Tockner, K., and Jähnig, S. C.: The global decline of freshwater megafauna, Global Change Biol., 25, 3883–3892, https://doi.org/10.1111/GCB.14753, 2019.
Herman, J. D., Quinn, J. D., Steinschneider, S., Giuliani, M., and Fletcher, S.: Climate Adaptation as a Control Problem: Review and Perspectives on Dynamic Water Resources Planning Under Uncertainty, Water Resour. Res., 56, e24389, https://doi.org/10.1029/2019WR025502, 2020.
Horne, A., Kaur, S., Szemis, J., Costa, A., Webb, J. A., Nathan, R., Stewardson, M., Lowe, L., and Boland, N.: Using optimization to develop a “designer” environmental flow regime, Environ. Model. Softw., 88, 188–199, https://doi.org/10.1016/J.ENVSOFT.2016.11.020, 2017a.
Horne, A. C., Webb, J. A., McClain, M., Richter, BrianStewardson, M. J., Poff, N. L., Hart, B., Acreman, M., O'Donnell, E., Bond, N., and Arthington, A. H.: Research Priorities to Improve Future Environmental Water Outcomes, Front. Environ. Sci., 5, 89, https://doi.org/10.3389/fenvs.2017.00089, 2017b.
Hurford, A., Huskova, I., and Harou, J. J.: Using many-objective trade-off analysis to help dams promote economic development, protect the poor and enhance ecological health, Environ. Sci. Policy, 38, 3259–3277, https://doi.org/10.1016/j.envsci.2013.10.003, 2014.
Hurford, A. P. and Harou, J. J.: Balancing ecosystem services with energy and food security – Assessing trade-offs from reservoir operation and irrigation investments in Kenya's Tana Basin, Hydrol. Earth Syst. Sci., 18, 3259–3277, https://doi.org/10.5194/hess-18-3259-2014, 2014.
Hurford, A. P., McCartney, M. P., Harou, J. J., Dalton, J., Smith, D. M., and Odada, E.: Balancing services from built and natural assets via river basin trade-off analysis, Ecosyst. Serv., 45, 101144, https://doi.org/10.1016/j.ecoser.2020.101144, 2020.
IPCC: Climate Change 2021: The Physical Science Basis, in: Contribution of Working Group I to the Sixth Assessment Report of the Intergovernmental Panel on Climate Change, edited by: Masson-Delmotte, V. P., Zhai, A., Pirani, S. L., Connors, C., Péan, S., Berger, N., Caud, Y. Chen, L., Goldfarb, M. I., Gomis, M., Huang, K., Leitzell, E., Lonnoy, J. B. R., Matthews, T. K., Maycock, T., Waterfield, O., Yelekçi, R. Y., and Zhou, B., Cambridge University Press, https://doi.org/10.1017/9781009157896, 2021.
Jin, L., Whitehead, P. G., Appeaning Addo, K., Amisigo, B., Macadam, I., Janes, T., Crossman, J., Nicholls, R. J., McCartney, M., and Rodda, H. J. E.: Modeling future flows of the Volta River system: Impacts of climate change and socio-economic changes, Sci. Total Environ., 637–638, 1069–1080, https://doi.org/10.1016/J.SCITOTENV.2018.04.350, 2018.
Jung, G., Wagner, S., and Kunstmann, H.: Joint climate–hydrology modeling: an impact study for the data-sparse environment of the Volta Basin in West Africa, Hydrol. Res., 43, 231–248, https://doi.org/10.2166/NH.2012.044, 2012.
Kiptala, J. K., Mul, M. L., Mohamed, Y. A., and van der Zaag, P.: Multiobjective Analysis of Green-Blue Water Uses in a Highly Utilized Basin: Case Study of Pangani Basin, Africa, J. Water Resour. Pl. Manage., 144, 05018010, https://doi.org/10.1061/(ASCE)WR.1943-5452.0000960, 2018.
Kumi, E. N.: The Electricity Situation in Ghana: Challenges and Opportunities, Washington, DC, https://www.cgdev.org/sites/default/files/electricity-situation-ghana-challenges-and-opportunities.pdf (last access: 18 May 2023), 2017.
Kunstmann, H. and Jung, G.: Regional Hydrological Impacts of Climatic Variability and Change, in: Proceedings of symposium S6 held 1 during the Seventh IAHS Scientific Assembly, April 2005, Foz do Iguaçu, Brazil, https://www.zef.de/fileadmin/template/Glowa/Downloads/Kunstmann_jung_2005.pdf (last access: 18 May 2023), 2005.
Lawson, R. M.: The changing economy of the Lower Volta 1954–67: A study in the dynanics of rural economic growth, Routledge, 1–127, https://doi.org/10.4324/9780429490637, 1972.
Legislative Instrument (L. I.) 1692 Water Use Regulations, Ghana, 2001.
Luisetti, T., Bateman, I. J., and Kerry Turner, R.: Testing the fundamental assumption of choice experiments: Are values absolute or relative?, Land Econ., 87, 284–296, https://doi.org/10.3368/LE.87.2.284, 2011.
Matrosov, E. S., Huskova, I., Kasprzyk, J. R., Harou, J. J., Lambert, C., and Reed, P. M.: Many-objective optimization and visual analytics reveal key trade-offs for London's water supply, J. Hydrol., 531, 1040–1053, https://doi.org/10.1016/j.jhydrol.2015.11.003, 2015.
McCartney, M., Forkuor, G., Sood, A., Amisigo, B., Hattermann, F., and Muthuwatta, L.: The water resource implications of changing climate in the Volta River Basin, IWMI Research Report, Colombo, Sri Lanka, 1–33, https://doi.org/10.5337/2012.219, 2012.
Moxon, J.: Man's greatest lake: The story of Ghana's Akosombo dam, Andre Deutsch, London, ISBN 10:0233959890, ISBN 13:978-0233959894, 1969.
Mul, M. L., Ofosu, E. A., Mante, Y., Ghansah, B., Annor, F. O., and Boateng-Gyimah, M.: Defining Restoration flow targets to restore ecological functions and livelihoods in the Lower Volta Basin, in: Dams, Development and Downstream Communities: Implications for Re-optimising the Operations of the Akosombo and Kpong Dams in Ghana, edited by: Ntiamoa-Baidu, Y., Ampomah, B. Y., and Ofosu, E. A., Digibooks Ghana Ltd, Tema, Ghana, 185–209, ISBN 978-9988-2-5046-1, 2017.
Ntiamoa-Baidu, Y., Ampomah, B. Y., and Ofosu, E. A.: Dams, development and downstream communities: implications for re-optimising the operations of the Akosombo and Kpong Dams in Ghana, xv,Digibooks Ghana Ltd, Tema, Ghana, 466 pp., ISBN 978-9988-2-5046-1, 2017.
Nukpezah, D., Sawyerr, L. M., Twum-Barimah, R., and Ntiamoa-Baidu, Y.: Re-Optimisation and Re-Operation Study of Akosombo and Kpong Dams: Voices from the Downstream Communities, in: Dams, development and downstream communities: implications for re-optimising the operations of the Akosombo and Kpong Dams in Ghana, edited by: Ntiamoa-Baidu, Y., Ampomah, B. Y., and Ofosu, E. A., Digibooks Ghana Ltd, Tema, Ghana, 27–42, ISBN 978-9988-2-5046-1, 2017.
Nyekodzi, G., Lawson, E. T., and Gordon, C.: Evaluating the impacts of dredging and saline water intrusion on rural livelihoods in the Volta Estuary, Int. J. River Basin Manage., 16, 93–105, https://doi.org/10.1080/15715124.2017.1372445, 2018.
Obirikorang, K. A., Amisah, S., and Adjei-Boateng, D.: Habitat Description of the Threatened Freshwater Clam, Galatea paradoxa (Born 1778) at the Volta Estuary, Ghana, Curr. World Environ. J., 8, 331–339, https://doi.org/10.12944/cwe.8.3.01, 2013.
Ohemeng, F., Nartey, N. N. A., Sawyerr, L. M., Twum-Barimah, R., and Ntiamoa-Baidu, Y.: Re-Operation and Re-Optimisation of Akosombo and Kpong Dams – Engaging Downstream Communities in Re- Operation Scenario Options, in: Dams, development and downstream communities: implications for re-optimising the operations of the Akosombo and Kpong Dams in Ghana, edited by: Ntiamoa-Baidu, Y., Ampomah, B. Y., and Ofosu, E. A., Digibooks Ghana Ltd, Tema, Ghana, 257–275, ISBN 978-9988-2-5046-1, 2017.
Owusu, A.G., Mul, M., van der Zaag, P., and Slinger, J.: May the odds be in your favour: Why many attempts to re-operate dams for the environment stall. J. Water Resour. Pl. Manage., 148, 04022009, https://doi.org/10.1061/(ASCE)WR.1943-5452.0001521, 2022a.
Owusu, A., Mul, M., Strauch, M., van der Zaag, P., Volk, M., and Slinger, J.: The clam and the dam: A Bayesian belief network approach to environmental flow assessment in a data scarce region, Sci.e Total Environ., 810, 151315, https://doi.org/10.1016/J.SCITOTENV.2021.151315, 2022b.
Owusu, A., Zatarain Salazar, J., Mul, M., van der Zaag, P., and Slinger, J.: Vol_Opt v1.0: Evolutionary Multi-Objective Direct Policy Search for the Akosombo and Kpong dams, Zenodo [code], https://doi.org/10.5281/zenodo.7947905, 2023.
Owusu, A. G., Mul, M., van der Zaag, P., and Slinger, J.: Re-operating dams for environmental flows: From recommendation to practice, River Res. Appl., 37, 176–186, https://doi.org/10.1002/rra.3624, 2021.
People, W. and Rogoyska, M.: The effect of the Volta River Hydroelectric Project on the salinity of the Lower Volta River, Ghana, Science, 9, 9–20, 1969.
Postel, S. and Richter, B.: Rivers for Life- Managing Water for People and Nature, Island Press, Washington, ISBN 10:1559634448, ISBN 13:978-1559634441, 2003.
Public Utilities Regulatory Commission: Public Utilities Regulatory Commission Press Release: Approved Electricity and Water Tariffs Effective 14 December 2015, https://www.purc.com.gh/attachment/394504-20201203041213.pdf (last access: 18 May 2023), 2015.
Quinn, J. D., Reed, P. M., Giuliani, M., and Castelletti, A.: Rival framings: A framework for discovering how problem formulation uncertainties shape risk management trade-offs in water resources systems, Water Resour. Res., 53, 7208–7233, https://doi.org/10.1002/2017WR020524, 2017.
Reed, P. M., Hadka, D., Herman, J. D., Kasprzyk, J. R., and Kollat, J. B.: Evolutionary multiobjective optimization in water resources: The past, present, and future, Adv. Water Resour., 51, 438–456, https://doi.org/10.1016/j.advwatres.2012.01.005, 2013.
Richter, B. D., Postel, S., Revenga, C., Scudder, T., Lehner, B., Churchill, A., and Chow, M.: Lost in development's shadow: The downstream human consequences of dams, Water Alternat., 3, 14–42, 2010.
Roest, L. W. M.: The coastal system of the Volta delta, Ghana: Strategies and opportunities for development, TU Delft Delta Infrastures and Mobility Initiative (DIMI), 40 pp., https://pure.tudelft.nl/ws/portalfiles/portal/37464456/Roest_2018_The_coastal_system_of_the_Volta_delta.pdf (last access: 18 May 2023), 2018.
Roudier, P., Ducharne, A., and Feyen, L.: Climate change impacts on runoff in West Africa: a review, Hydrol. Earth Syst. Sci., 18, 2789–2801, https://doi.org/10.5194/hess-18-2789-2014, 2014.
Schlömer, S., Bruckner, T., Fulton, L., Hertwich, E., McKinnon, A. U., Perczyk, D., Roy, J., Schaeffer, R., Hänsel, G., de Jager, D., Bruckner, T., Fulton, L., Hertwich, E., McKinnon, A., Perczyk, D., Roy, J., Schaeffer, R., Sims, R., Smith, P., Wiser, R., Pichs-Madruga, R., Sokona, Y., Farahani, E., Kadner, S., Seyboth, K., Adler, A., Baum, I., Brunner, S., Eickemeier, P., Kriemann, B., Savolainen, J., Schlömer, S., von Stechow, C., Zwickel, T., and Minx, J.: Annex III: Technology-specific cost and performance parameters, in: Climate Change 2014: Mitigation of Climate Change, Contribution of Working Group III to the Fifth Assessment Report of the Intergovernmental Panel on Climate Change, https://www.ipcc.ch/site/assets/uploads/2018/02/ipcc_wg3_ar5_annex-iii.pdf (last access: 18 May 2023), 2014.
Smith, L. E. D.: Assessment of the contribution of irrigation to poverty reduction and sustainable livelihoods, Int. J. Water Resour. Dev., 20, 243–257, https://doi.org/10.1080/0790062042000206084, 2004.
Sood, A., Muthuwatta, L., and McCartney, M.: A SWAT evaluation of the effect of climate change on the hydrology of the Volta River basin, Water Int., 38, 297–311, https://doi.org/10.1080/02508060.2013.792404, 2013.
Stone, R.: Hydropower. The legacy of the Three Gorges Dam, Science, 333, 817, https://doi.org/10.1126/science.333.6044.817, 2011.
Sylla, M. B., Faye, A., Klutse, N. A. B., and Dimobe, K.: Projected increased risk of water deficit over major West African river basins under future climates, Climatic Change, 151, 247–258, https://doi.org/10.1007/s10584-018-2308-x, 2018.
The World Bank: International Development Association Project Appraisal Document for the Ghana Energy Sector Transformation Initiative Project, Project Document PAD2576, Washington, DC, https://documents1.worldbank.org/curated/en/293271531711852754/pdf/GHANA-ENERGY-PAD-06252018.pdf (last access: 18 May 2023), 2018.
Tsikata, D.: Living in the Shadow of the Large Dams. Long Term Responses of Downstream and Lakeside Communities of Ghana's Volta River Project, Brill, 685–685, https://doi.org/10.1080/03056240802574250, 2008.
Vörösmarty, C. J., Douglas, E. M., Green, P. A., and Revenga, C.: Geospatial indicators of emerging water stress: An application to Africa, Ambio, 34, 230–236, https://doi.org/10.1579/0044-7447-34.3.230, 2005.
VRA – Volta River Authority: 1,020 MW Akosombo Hydro Electric Power Plant, https://vra.com/our_mandate/akosombo_hydro_plant.php# (last access: 2 April 2021), 2021.
Warner, A. T., Bach, L. B., and Hickey, J. T.: Restoring environmental flows through adaptive reservoir management: planning, science, and implementation through the Sustainable Rivers Project, Hydrolog. Sci. J., 59, 770–785, https://doi.org/10.1080/02626667.2013.843777, 2014.
WCD: Dams and Development: A new framework for decision-making, in: 1st Edn., Earthscan Publications Ltd, London, https://doi.org/10.1097/GCO.0b013e3283432017, 2000.
Wild, T. B., Reed, P. M., Loucks, D. P., Mallen-Cooper, M., and Jensen, E. D.: Balancing hydropower development and ecological impacts in the Mekong: Tradeoffs for Sambor Mega Dam, J. Water Resour Pl. Manage., 145, 05018019, https://doi.org/10.1061/(ASCE)WR.1943-5452.0001036, 2019.
WWF: Living Planet Report – 2018: Aiming higher, Environmental Conservation, Gland, Switzerland, 1–14, ISBN 978-2-940529-90-2, 2018.
Yang, W. and Yang, Z.: Effects of long-term environmental flow releases on the restoration and preservation of Baiyangdian Lake, a regulated Chinese freshwater lake, Hydrobiologia, 730, 79–91, https://doi.org/10.1007/s10750-014-1823-7, 2014.
Zatarain Salazar, J., Reed, P. M., Herman, J. D., Giuliani, M., and Castelletti, A.: A diagnostic assessment of evolutionary algorithms for multi-objective surface water reservoir control, Adv. Water Resour., 92, 172–185, https://doi.org/10.1016/j.advwatres.2016.04.006, 2016.
Zatarain Salazar, J., Reed, P. M., Quinn, J. D., Giuliani, M., and Castelletti, A.: Balancing exploration, uncertainty and computational demands in many objective reservoir optimization, Adv. Water Resour., 109, 196–210, https://doi.org/10.1016/j.advwatres.2017.09.014, 2017.