the Creative Commons Attribution 4.0 License.
the Creative Commons Attribution 4.0 License.
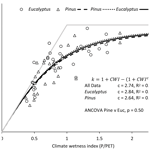
Is the reputation of Eucalyptus plantations for using more water than Pinus plantations justified?
Don A. White
Shiqi Ren
Daniel S. Mendham
Francisco Balocchi-Contreras
Richard P. Silberstein
Dean Meason
Andrés Iroumé
Pablo Ramirez de Arellano
The effect of Eucalyptus plantations on water balance is thought to be more severe than for commercial alternatives such as Pinus species. Although this perception is firmly entrenched, even in the scientific community, only four direct comparisons of the effect on the water balance of a Eucalyptus species and a commercial alternative have been published. One of these, from South Africa, showed that Eucalyptus grandis caused a larger and more rapid reduction in streamflow than Pinus patula. The other three, one in South Australia and two in Chile, did not find any significant difference between the annual evapotranspiration of E. globulus and P. radiata after canopy closure.
While direct comparisons are few, there are at least 57 published estimates of annual evapotranspiration of either the Eucalyptus or Pinus species. This paper presents a meta-analysis of these published data. Zhang et al. (2004) fitted a relationship between the vegetation evaporation efficiency and the climate wetness index to published data from catchment studies and proposed this approach for comparing land uses. We fitted this model to the published data for Eucalyptus and Pinus and found that the single parameter of this model did not differ significantly between the two genera (p=0.48). This was also the case for all parameters of an exponential relationship between evapotranspiration and rainfall (p=0.589) and a linear relationship between the vegetation evaporation index and rainfall (p=0.155).
These results provide strong evidence that, for a given climate wetness index, the two genera have similar annual water use. The residuals compared to the model of Zhang et al. (2004) were significantly correlated with soil depth for Eucalyptus, but this was not the case for Pinus. For Eucalyptus, the model overestimates the vegetation evaporation efficiency on deep soils and underestimates the vegetation evaporation efficiency on shallow soils.
- Article
(1547 KB) -
Supplement
(427 KB) - BibTeX
- EndNote
There are now more than 23 Mha of Eucalyptus plantations in the temperate and tropical zones of the world (Keenan et al., 2015; MacDicken et al., 2016). These plantations extend from near the Equator to approximately 43∘ of latitude north and south and play an important and growing role in minimising the gap between global demand for wood products and the supply (Kanninen, 2010). These Eucalyptus plantations are mostly established in seasonally dry climate zones (dry tropics, sub-tropics, and Mediterranean climate types). This, and the reputation of Eucalyptus for high rates of water use when compared to alternatives, means that wherever large-scale planting of Eucalyptus has occurred, it has been associated with concern, debate, and often protest about the effect of these plantations on the security of water supply (Albaugh et al., 2013). Afforestation with Pinus and other genera has also resulted in concern about changes in local hydrology (Huber and Iroumé, 2001; Little et al., 2009) but has not been associated with the same level of polemic or controversy as the planting of Eucalyptus.
In 2010, plantations managed for wood production occupied a total land area of 109 Mha (Kanninen, 2010). Approximately 35 % of these plantations were of Pinus species, while 10 % were Eucalyptus (Kanninen, 2010). The annual increase in production plantations between 2010 and 2015 was 1.2 %. During this time, the total area of Pinus plantations remained virtually unchanged, and much of the global increase was in either Eucalyptus plantations or other short-rotation options such as Acacia (Payn et al., 2015). The global trends in plantations are towards Eucalyptus or species managed on short rotations to grow pulp or biomass for energy. While these global trends are important, the conflict associated with the establishment of Eucalyptus plantations and the potential for reduced water availability manifests locally. In South Africa and South Australia, these concerns have resulted in legislation to either regulate water use (Greenwood, 2013) or planting (Albaugh et al., 2013). The effects of Eucalyptus on water are currently being actively debated in Chile, where Arauco SA (the largest plantation grower in Chile and the second-largest pulp producer in the world) plan to replace approximately 250 000 ha of P. radiata plantations with Eucalyptus. In China, regional governments are supporting research to investigate the water benefits of mixed plantings of local species with Eucalyptus. It is also likely that the global goal of reduced CO2 emissions will intensify the debate about Eucalyptus water use. Given the dominance of the global plantation estates by species of Pinus and Eucalyptus, and the direct substitution of Pinus with Eucalyptus, a quantitative comparison between the water use characteristics of these two genera is timely.
The evidence that plantations use more water than grasslands or dryland crops is very strong (Zhang et al., 2001; Zhang, 2004). Similarly, there is evidence that plantations use more water, and therefore generate less streamflow, than natural forest in Chile (Huber et al., 2008), Brazil (Almeida et al., 2007; Meinzer et al., 1999) and Spain (Rodriguez Suarez et al., 2014). The magnitude of the difference between plantations and natural forest is less than that observed between plantations and annual pastures (Zhang et al., 2004).
While there is a perception that Eucalyptus use more water than alternative commercial plantation options such as Pinus, three of four published comparisons of the water use (defined as evapotranspiration) reported no difference between the water use of species from these two genera. The evidence for higher rates of water use by Eucalyptus is mostly from South Africa, where, in a paired catchment study, Scott and Lesch (1997) showed that, at least in the early stages of growth, Eucalyptus grandis W. Hill. used up to 92 mm more water per year than Pinus patula Schiede ex Schltdl. et Cham. In another direct comparison of the water use of a Pinus and Eucalyptus species in plantations, Benyon et al. (2006) found that the annual water use of plantations of E. globulus Labill. and P. radiata D. Don., with or without access to shallow fresh groundwater, were not significantly different. Recent stand- and catchment-scale comparisons of P. radiata and E. globulus in central Chile have not observed significant differences between the average annual water use of P. radiata and E. globulus (Iroumé et al., 2021; White et al., 2021). Given these contrasting results, and the trend towards more planting of Eucalyptus, it is important to understand when and why differences might occur in the water balance of Pinus and Eucalyptus plantations.
Studies in Brazil (Lima et al., 1990) and Tasmania, Australia (Honeysett et al., 1996), have shown that, when planted in deep soils and with regular inputs of rainfall or irrigation, Eucalyptus plantations can use water at a rate that approaches the energy limit. Similar high rates of water use have also been observed in P. radiata plantations in southern Australia (Benyon et al., 2006) and in Chile (Huber and Iroumé, 2001). Studies in China have found that the annual rate of water use by Eucalyptus can be substantially less than both rainfall and available energy (Lane et al., 2004; Ren et al., 2019). This occurs during the dry season and has also been observed in Pinus species (Myers et al., 1998). Notwithstanding these similarities, it has been observed that the water use of Pinus species decreases more rapidly with the onset of water stress than is the case with commercial Eucalyptus alternatives for the same site (Teskey and Sheriff, 1996).
Reviews of the water use potential of Eucalyptus have highlighted the variability in reported rates of both transpiration and evapotranspiration (Albaugh et al., 2013; Shi et al., 2012), yet there has been no systematic attempt to determine if the high rate of water use observed in some studies is a characteristic of Eucalyptus in plantations or if it has more to do with the conditions that prevailed in those studies. Most of the published studies of water balance, with a couple of exceptions (Mendham et al., 2011; Scott and Lesch, 1997), have reported water balance measurements made within a single rotation, and most studies cover only a small proportion of that rotation. It is likely that plantations must eventually reach a long-term equilibrium with the local climate and that, except in circumstances where trees have access to off-site water such as a regional aquifer (see O'Grady et al., 2011, for a meta-analysis), these high rates of water use, often observed early in the first rotation, will not be sustained. What is needed is to determine if the longer-term equilibrium water balance of catchments planted with Eucalyptus will be associated with different levels of water storage, and therefore streamflow, from those under alternative species options for wood production plantations (McDonnell, 2017).
While there are only four direct comparisons of the annual water balance of Pinus and Eucalyptus, there are many studies that quantify annual water use by either a Eucalyptus or a Pinus species. These studies, and their estimates of water use, are very situation specific. Comparison of alternative land uses is complicated by the dominant role that climate and hydrogeology play in determining the local water balance. While vegetation cover has a smaller effect on the catchment water balance than either climate or hydrogeology, it is the part of the system that can be actively managed. If studies are available for the two genera from a comparable range of annual rainfall and evaporative environments, then comparison might be possible through normalising water use (evapotranspiration) with respect to potential or energy-limited evaporation and plotting this as a function of the climate wetness index (ratio of rainfall to potential evaporation). While this approach has previously been used to compare the water use of forests with dryland agriculture (Zhang et al., 2004), the normalisation of both axes with respect to potential evaporation may mask the effect of vegetation on evapotranspiration.
In this study, we collated published annual water balance estimates for plantations with either Eucalyptus and/or a Pinus species. To test the null hypothesis that the evaporation of commercial plantations of Pinus and Eucalyptus was the same, we fitted three models to the data, including the model described by Zhang et al. (2004), an exponential relationship between evapotranspiration and rainfall, and a linear relationship between the vegetation evaporation efficiency and rainfall. We also test the hypothesis that variation from the first model is determined by variation in soil depth.
This paper presents a meta-analysis of published measurements of the water balance of Eucalyptus and Pinus plantations in tropical and temperate regions. The focus of this analysis is on post-canopy closure plantations in a notional equilibrium with the site. The behaviour of the plantations is quantified by comparing an index of the function of the crop (the vegetation evaporation efficiency, VEE) with an index of climate wetness, in the manner proposed by Budyko (1974) and applied by Zhang et al. (2004), to compare forests with dryland agricultural systems.
2.1 Definitions of terms
The terms evapotranspiration, water use, potential evaporation, vegetation evaporation efficiency, and climate wetness index have various meanings in the scientific literature, and to avoid ambiguity, they are defined here as they are used in this paper.
2.1.1 Evapotranspiration and water use
Evapotranspiration (ET) and water use are used in this paper to describe the total evaporation from a vegetated land surface. They are the sum of the transpiration of all plants (T; the evaporation through leaf and other plant surfaces of water drawn from the soil and transported to sites of evaporation through the xylem), water intercepted by plant canopies and evaporated without reaching the ground (interception, I), and evaporation of water directly from soil and litter (often called soil evaporation, Es). All these processes are affected by the choice of crop and by the management of that crop and should therefore be included as part of the water use of that vegetation.
2.1.2 Potential evaporation (PET)
Evapotranspiration (ET) by any land use is situation specific; it is affected by the climate (energy and rainfall), the structure and function of the vegetation, and by characteristics of the soil and the litter. In this paper, for the purposes of comparison, estimates of water use or evapotranspiration are normalised relative to measures of the local water supply (rainfall) and potential evaporation, which represents the energy-limited maximum rate of evaporation. There are numerous measures of reference or potential evaporation, including the Penman potential evaporation (Penman, 1949), FAO56 reference evaporation (Allen et al., 2005), pan evaporation, and Priestley–Taylor potential evaporation (Priestley and Taylor, 1972). They are all intended to represent the maximum possible rate of evaporation by a land surface covered with vegetation. In this paper, potential evaporation (PET) always refers to Priestley–Taylor potential evaporation (see the notes under data analysis below to see how the Priestley–Taylor PET was calculated for each site). We have used the coefficient 1.26 in the Priestley–Taylor equation; this coefficient accounts for the extra roughness of forests when compared with short crops and pastures (Eichinger et al., 1996). The evapotranspiration of plantations may still, of course, exceed this measure of PET. This may be the case if there is an additional source of energy such as the advection or movement of hot air into the forest. This might occur at the edge of a plantation, especially of it is adjacent to an area of land from which there is a large sensible heat flux. The choice of method for calculating PET is less important than applying the same method for all calculations in this analysis.
2.1.3 Water and energy limit, vegetation evaporation efficiency (k), and climate wetness index (CWI)
The climate imposes limits on evapotranspiration. Evapotranspiration cannot exceed the amount of water available, which is usually limited to rainfall but may include irrigation and soil stored water and groundwater (O'Grady et al., 2011). Similarly, although evapotranspiration may exceed the calculated PET under some circumstances, it is ultimately limited by available energy.
The relationship between the ratio of actual evapotranspiration to potential evaporation and the climate wetness index (CWI, which is the ratio of rainfall to potential evaporation; Budyko, 1974) provides a simple way of partitioning rainfall between evaporation and runoff. Within this framework, evapotranspiration is water limited when it is less than rainfall and energy limited when it exceeds rainfall. The ratio of evapotranspiration to potential evaporation is termed the evaporation efficiency of a surface (Komatsu, 2003). For example, the ratio of evaporation from a soil to the potential soil evaporation is referred to as the soil evaporation efficiency (Merlin et al., 2016). In this paper, the ratio of evapotranspiration to reference evaporation for commercial plantations of Eucalyptus and Pinus species is referred to as their vegetation evaporation efficiency (VEE). A more evaporation-efficient plantation converts a relatively greater proportion of available energy to latent rather than sensible heat.
Zhang et al. (2004) developed a simple model that predicted the vegetation evaporation efficiency (VEE) as a function of the climate wetness index (CWI). This model is given by Eq. (1) (Eq. A22 in Zhang et al., 2004) below and includes the parameter c (an empirical catchment characteristic), which captures the effect of hydrogeology and vegetation cover on the vegetation evaporation efficiency.
2.2 Meta-analysis of published studies
While direct comparisons of the water balance of Eucalyptus and Pinus plantations are few, there are a reasonable number of previously published estimates of either streamflow or evapotranspiration. These data were collated and used in the meta-analysis described below. The studies included are described in some detail in the Supplement, and the main features are summarised in Tables 1 and 2. A list of potentially suitable references was first found by conducting a series of searches of the Web of Science and Google Scholar. The following searches were conducted:
-
title contains (evapotranspiration or water use) and (eucalypt or eucalyptus);
-
title contains (evapotranspiration or water use) and (pine or pinus);
-
paper contains (evapotranspiration or water use) and (eucalypt or eucalyptus); and
-
paper contains (evapotranspiration or water use) and (pine or pinus).
The first two searches yielded fewer than 100 papers in total. The latter two found many thousands of articles. The 200 most relevant in each search were checked to decide their suitability. For inclusion, the paper must measure or estimate evapotranspiration by a Eucalyptus or Pinus species for at least 1 year. Only planted forests managed primarily for wood production were included. Agroforestry systems were excluded, as were measurements made prior to canopy closure. Native forests and burned forests and plantations with access to the water table were also excluded. Several of the studies covered multiple years. A single value of rainfall and evaporation was calculated as the average of all the years in each study. Sometimes a paper reported multiple estimates of evapotranspiration for forests in the same location and growing under the same conditions. In these cases, average values were calculated for the multiple sites.
Table 1Brief description of all the papers and the associated studies included in the meta-analysis. See the Supplement for a full summary of the data used in the analysis. Data are sorted by region and annual rainfall. The annual rainfall data provided here are measurements from the cited paper, unless noted otherwise.
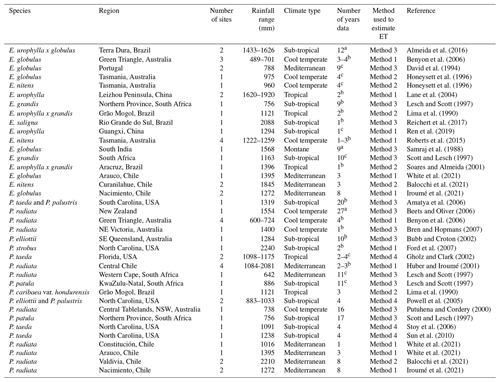
a Full rotation. b Post-canopy closure only. c Includes pre- and post-canopy closure.
Table 2Summary of the studies included in the meta-analysis (see the Supplement for more details and the references for each study). This table indicates the number of studies included by country or continent, species, and climate zone.
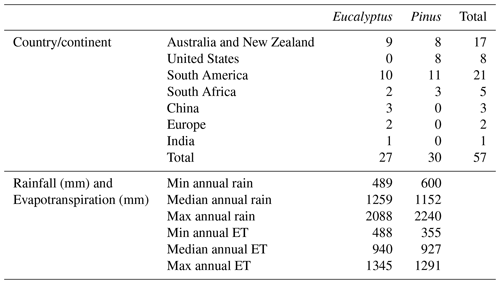
After applying these criteria to the articles found in the above searches, a total of 30 Pinus and 27 Eucalyptus stands were included in the meta-analysis. The location, rainfall data, and evapotranspiration data are provided in the Supplement. The estimates of evapotranspiration were made using one of four methods. The method applied in each study is indicated in Table 1.
2.2.1 Method 1 – measurement and addition of component fluxes
At the stand or plot scale, evapotranspiration (water use) is the sum of evaporation from the soil and leaf litter (Es), evaporation of rainfall intercepted by the vegetation canopy (I), and transpiration or the direct uptake of water by the trees and the evaporation of this water through the leaf surface (T). Evapotranspiration can therefore be calculated as the sum of the component processes.
2.2.2 Method 2 – one-dimensional water balance
Provided there is no leakage or runoff, evapotranspiration (ET) can be calculated in stand-scale studies as the sum of rainfall (P) and the change in the soil water content (ΔS) between two measurements.
2.2.3 Method 3 – catchment water balance
For a catchment, if there is no change in the amount of water stored in the soil or the groundwater (ΔS), then evapotranspiration (ET) is simply the difference between rainfall and streamflow (Q). Over long time periods, it is often assumed that the change in storage is negligible; this is less valid when the period of the estimate is reduced or if the annual total rainfall has a clear temporal trend.
2.2.4 Method 4 – eddy covariance (flux towers)
Properly located flux towers can be used to estimate the net carbon and water flux (evapotranspiration) above an ecosystem. The instruments on these towers measure the total solar and net radiation and partition this to latent (evapotranspiration) and sensible heat flux (air temperature change) and heat storage changes in soil and biomass. The covariances of high-frequency measurements of air temperature, humidity, and CO2 are used to calculate the total evaporation and carbon exchange between the atmosphere and the underlying vegetation (Aubinet et al., 2012). Measurements are typically made on a 30 min time interval to represent fluxes from an upwind surface area or footprint. The area of the footprint is dependent on the strength of the turbulence in the air, a function of wind speed and surface roughness elements, and the height of the instruments; thus, the location of the land surface influencing the measurements changes through time. Eddy covariance measurements give total fluxes from the contributing footprint and are thus useful for total ecosystem energy, water, and carbon balances. However, partitioning the fluxes between different contributing vegetation and soil components requires additional measurements, such as sap flow, rain throughfall, and soil evaporation. Also, the measurements are unreliable during periods of stable air and low turbulence, such as still cold nights, but, for the purposes of the analyses in this paper, these are periods typically with very low water fluxes and have only minor influence on the total system water balance. There is substantial literature describing these methods and complementary measurements. A detailed description is beyond the scope of this paper but can be found in Wilson et al. (2001), where the method is compared with alternatives.
2.3 Variations at two sites
A study by Scott and Lesch (1997) in three catchments on the Mpumalanga escarpment in South Africa reported more rapid changes in streamflow after planting of E. grandis than after planting of P. patula. The soil was very deep, and it is probable, though this was not measured, that evapotranspiration exceeded rainfall and that this was more pronounced in the E. grandis than the P. patula. To allow for this effect, we assumed a storage of 100 mm m−1 of soil and a rate of root extension of 2 m yr−1 for E. grandis, after Dye (1996), and 1 m yr−1 in P. patula. This relative rate is consistent with the observation that streamflow ceased 5 and 10 years, respectively, after the planting of E. grandis and P. patula (Scott and Lesch, 1997).
Another study included here was made at Lewisham in Tasmania, Australia, by Honeysett et al. (1996). In this study, the effect of drought on the water relations and water balance of E. globulus and E. nitens was investigated using irrigated controls and rainfed plots. The irrigated treatments were excluded from this meta-analysis. However, to avoid mortality, the rainfed treatments received some supplementary irrigation. This irrigation is included in the rainfall figure in Table 1 and in the Supplement.
2.4 Derived climate and vegetation indices
In each of the papers included in this analysis, evapotranspiration (ET) was estimated from the measurement of other variables by one of the four methods described above. Rainfall data were available for all the studies included in this review. Time series climate data from the 0.5∘ grid point closest to each site were also downloaded for the duration of each experiment (Climate Research Unit Time Series v4.03; Harris et al., 2020). Net radiation was calculated for the location, after Hargreaves and Samani (1985), and then the Priestley–Taylor PET was calculated as follows:
where Rn is net radiation in watts per square metre (W m−2), λ is the latent heat of vaporisation of water (2245 kJ kg−1), s is the slope of the relationship between saturated vapour pressure and temperature (kPa ∘C−1), and γ is the psychrometric constant (kPa ∘C−1). These constants are temperature dependent; s was calculated using the empirical model in Eq. (5) (Monteith and Unsworth, 2013), and γ was calculated using Eq. (6) in which Ta and Pa are the average daily air temperature (calculated as the average of Tmax and Tmin) and atmospheric pressure (assumed to be 101.3 kPa). cp is the specific heat of dry air (1.013 kJ kg ∘C−1), and ε is the ratio of the molecular weight of water to dry air (0.622).
For each measurement year at each study location, the vegetation evaporation efficiency (VEE) and the climate wetness index were also calculated using Eqs. (7) and (8), respectively.
2.5 Meta-analysis
In total, three models were fitted to the data using the non-linear regression function in R (R-Core-Team, 2013), Eq. (1), an exponential relationship between ET and P (Eq. 9), and a linear relationship between VEE and P (Eq. 9).
In each case, the parameters and the coefficients of determination, R2, values were calculated for each genus separately and for the pooled data (R-Core-Team, 2013). Analysis of variance was also completed to test for a significant difference between Pinus and Eucalyptus in the parameters of each model (R-Core-Team, 2013). The residuals (predicted minus observed) from the first model (Eq. 1) were plotted against soil depth for the sites where these data were available. Linear regression was used to explore the relationship between annual transpiration and annual evapotranspiration. Simple t tests for non-paired observations were used to test for differences between the genera in annual evapotranspiration and the ratio of evapotranspiration to rainfall.
3.1 The plantations used in the meta-analysis
In total, 27 Eucalyptus and 30 Pinus sites were included in the meta-analysis. The details of these sites are summarised in three tables. The most detailed information is in the Supplement, together with the measured and calculated climatic data, estimated evapotranspiration, and the detailed results of the data analysis. The papers from which the data were taken are listed in Table 1, with the rainfall data, species studied, and the method used to estimate evapotranspiration. Table 2 summarises the range of climatic conditions and evaporation rates by species and indicates the number of studies for each species by country or continent.
The analysis included sites from tropical, dry tropical, sub-tropical, warm temperate, cool temperate, Mediterranean, and montane climates, with both genera represented in all but one climate type and in most locations. There is a bias of Pinus studies to the United States and of Eucalyptus to Australia (Table 2). Species of Eucalyptus represented in order of decreasing number of estimates were E. globulus (10), E. nitens (H. Deane & Maiden) Maiden (7), E. urophylla S. T. Blake (3), E. grandis (2), E. urophylla x grandis (2), E. urophylla x globulus (2), and E. saligna Sm. (1) (Table 1). Estimates for species of Pinus were made for P. radiata (18), P. taeda L. (5), P. patula (2), a mixed stand of P. taeda and P. palustris Miller (1), a mixed stand of P. elliottii Engel. and P. palustris (1), P. elliottii (1), P. caribaea var. hondurensis W. H. Barrett and Golfari (1), and P. strobus L. (1) (Table 1). Thus, each genus is represented by species from tropical, sub-tropical, and temperate environments.
3.2 Annual rainfall and evapotranspiration
The annual rainfall at the 24 Eucalyptus sites ranged from 489 mm at one of the South Australian sites to 2088 mm at a site in the Rio Grande do Sul in southern Brazil. The range of rainfall was similar for the 27 Pinus sites and varied from 600 mm at a South Australian site to 2081 mm at a site near Valdivia in south–central Chile. Interestingly, both the low-rainfall site in South Australia and the high-rainfall site in Chile were planted with P. radiata. The situation was similar for the average annual potential evaporation, which ranged from 1005 to 2008 mm at the Eucalyptus sites and from 1021 to 2004 mm at the Pinus sites (see the Supplement). The median annual rainfall for the Eucalyptus and Pinus sites, respectively, was 940 and 927 mm, while average potential evaporation was 1480 and 1551 mm (Table 2). Thus, the range and median conditions covered by the sites included in this meta-analysis were very similar for both genera.
Annual rates of evapotranspiration reported for Eucalyptus species were between 488 mm at a low-rainfall site in South Australia planted with E. globulus (Benyon et al., 2006) and 1345 mm at a site in Brazil planted with E urophylla x E. grandis (Soares and Almeida, 2001). The lowest and highest annual evapotranspiration for Pinus species were 355 mm for P. radiata at Jonkershoek, Western Cape, South Africa (Lesch and Scott, 1997), and 1291 mm for P. strobus in North Carolina (Ford et al., 2007).
The minimum, mean, median, and maximum rates of evapotranspiration were all slightly greater for the Eucalyptus sites than for the Pinus sites (Fig. 1), but this difference was not significant (p=0.24). When evapotranspiration was divided by rainfall, the mean values of the ratio for the two genera were nearly identical at 0.77 and 0.76 (Fig. 2). The ratio of evapotranspiration to rainfall varied from 0.45 to 1.31 in Eucalyptus and from 0.44 to 1.2 in Pinus species. At one site in South Africa (Lesch and Scott, 1997), the rate of evapotranspiration by E. grandis exceeded rainfall by 31 % (Fig. 2). At the same site, evapotranspiration by P. patula exceeded rainfall by 19 % (Fig. 2).
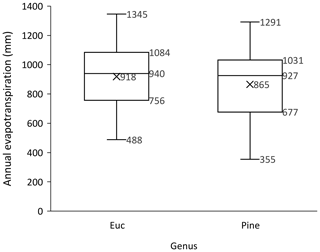
Figure 1Box-and-whisker plots of annual evapotranspiration for the Eucalyptus and the Pinus sites. The three horizontal lines in the box show the median and 25th and 75th percentile values. The whiskers show the minimum and maximum values, and x indicates the mean values. The associated labels indicate the actual values.
3.3 The effect of genus (Eucalyptus and Pinus) on the parameters of three models
Genus (Eucalyptus or Pinus) did not have a significant effect on any parameter in any of the three models (Table 3). Models 2 and 3 were included to check if normalisation with respect to potential evaporation in Model 1 (Eq. 1, Zhang et al., 2004) was masking the effect of genus on evapotranspiration. The results of the analysis of covariance for Model 2 and Model 3 suggest that this was not the case. However, the lower p value for the effect of genus on the slope parameter of Model 3 is noteworthy and was the result of two to three points of high leverage associated with sites where the soil was very deep (>20 m) or where some irrigation was applied during summer. The full results of the analysis and figures for Models 2 and 3 are included in the Supplement.
3.4 Vegetation evaporation efficiency as a function of the climate wetness index (Eucalyptus and Pinus)
In Fig. 3, the vegetation evaporation efficiency for each study site is plotted as a function of the climate wetness index. For both the Eucalyptus and Pinus sites, there is a strong, positive correlation between the vegetation evaporation efficiency and the climate wetness index. For the Eucalyptus sites, the model of Zhang et al. (2004; Eq. 1) explained 66 % of the variation in the vegetation evaporation efficiency, while for Pinus this decreased to 63 %. The parameter c in the model described by Eq. (1) fitted to the data was 2.84 for Eucalyptus and 2.64 for Pinus. While this may be an important difference, it was not statistically significant (p=0.50), and the value for c when the relationship was fitted to the pooled data was 2.74, and the R2 was 0.69. Figure 4 shows the ratio of the predicted vegetation evaporation efficiency for Eucalyptus to the predicted vegetation evaporation efficiency for Pinus as a function of climate wetness index. The maximum proportional effect of genus on the vegetation evaporation efficiency of 3.5 % is predicted to occur where the climate wetness index is 1.
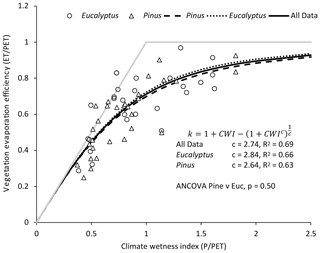
Figure 3The vegetation evaporation efficiency as a function of the climate wetness index (a Budyko plot) for 57 (27 Eucalyptus and 30 Pinus) published studies. The solid grey lines are the water limit (evapotranspiration is equal to rainfall) and the energy limit (evapotranspiration is equal to potential evaporation). The dotted and dashed lines are for Eq. (1) fitted separately to the data for Eucalyptus and Pinus.
3.5 The effect of soil depth
While the relationships in Fig. 3 are significant for both genera, there is nonetheless substantial scatter. The soil depth was not provided in all the papers included in this analysis. When the residuals (observed minus predicted) were plotted as a function of the soil depth, the relationship was significant for the Eucalyptus sites (Fig. 5) but not for the Pinus sites (data not shown). A linear relationship with soil depth explained 57 % of the error for Eucalyptus and indicated that the model shown in Fig. 3, for c of 3.1, overestimated the vegetation evaporation efficiency in shallow soils and underestimated it in deep soils (Fig. 5), with the model having zero residual with a soil depth around 10 m.
3.6 Transpiration as a proportion of evapotranspiration
A subset of the studies, again indicated in the Supplement, also provided estimates of transpiration made using sap flow sensors. For both Eucalyptus and Pinus, there was a strong linear relationship between transpiration and evapotranspiration, with an approximate slope of 0.5 (Fig. 6).
The results of the meta-analysis of the published records of evapotranspiration for Eucalyptus and Pinus species in this paper suggest that, for a given climate wetness index, the water use of Eucalyptus and Pinus plantations is not significantly different (p=0.50). This does not mean that there are not circumstances, or periods within a rotation, when Eucalyptus will use more water than the alternatives. The water balance of plantations and alternatives is very situation specific, and our focus should be on understanding the sources of variation rather than generalising about one land use option. The work of Scott and Lesch (1997) and the results of White et al. (2009) from three E. globulus plantations established in southwestern Australia highlight the potential of Eucalyptus plantations to exceed the water limit early in the rotation on deep soils. This is an issue that warrants deeper understanding and the development of management strategies. The results of the meta-analysis suggest that the average annual water use by the two genera will be similar over large areas and long time periods (decades). They do not, however, preclude periods of high water use by Eucalyptus.
The range of annual rainfall, climate wetness indices, and annual evapotranspiration in the published studies was similar for the 27 Eucalyptus and 30 Pinus sites included in meta-analysis (Tables 1 and 2 and the Supplement). Only a few sites had climate wetness indices more than 1.5. These were Jijia and Hetou in China, Huape and Valdivia in central Chile, and Coweeta in North Carolina. In the case of the Chinese sites, Lane et al. (2004) and Ren et al. (2019) concluded that plantations of Eucalyptus would neither have an important effect on water resources nor on water security in this part of China. Notwithstanding this conclusion, there is still a lot of investment made to quantify the water use of Eucalyptus in these regions. Wherever the climate wetness index exceeds 1.5, the amount of streamflow will always be substantial, even in lower-rainfall years (White et al., 2016). Thus, rather than annual water balance, the focus should be on water quality and dry season flow to better understand the effect of land use change, including the planting of Eucalyptus, on water security.
For the published Eucalyptus and Pinus studies analysed here, there was a strong positive correlation between evapotranspiration and rainfall and therefore between the vegetation evaporation efficiency and the climate wetness index (Fig. 3). The coefficient, or catchment characteristic, c was greater in Eucalyptus (2.84) than in Pinus (2.64), but the difference between the two genera was not statistically significant (p=0.50). When this result was discussed with colleagues in the forestry sector or with people in the forest research community, it was met with responses ranging from mild surprise to disbelief. The belief that Eucalyptus uses more water than any of the alternative crops is very firmly entrenched, even though it does not seem to have a firm scientific foundation. Given that the meta-analysis presented in this paper produced a result that was counter to the prevailing view, it is very important to consider the direct and corroborative evidence that either support or oppose this observation. The following paragraphs attempt to provide a mechanistic basis for the observation that, while under some circumstances Eucalyptus can use water much more rapidly than Pinus (Scott and Lesch, 1997), the average behaviour of the two genera appears similar (Benyon and Doody, 2015; Fig. 3). This mechanistic basis is then used to indicate under which circumstances the effects of plantations of Pinus or Eucalyptus species on water resources should be evaluated and actively managed.
The key to understanding the patterns of water use in Eucalyptus and Pinus plantations lies in the hydraulic architecture of the two genera and in the way that this affects the relationship between water use and carbon gain. There are some consistent differences between the group of Eucalyptus and Pinus species that are grown in commercial plantations. First, and very importantly, Pinus species are gymnosperms, and their water conducting elements are tracheids, while in Eucalyptus water is transported in vessels. The maximum hydraulic conductivity of angiosperms exceeds that of conifers with almost no overlap in the ranges (Sperry et al., 2006). It is the diameter of the vessels that affords angiosperms greater maximum hydraulic conductance (Sperry et al., 2006). It is also known that, in the Eucalyptus genus vessel size, the maximum hydraulic conductivity of the xylem is correlated with climate wetness (Pfautsch et al., 2016) so that the major plantation species can have hydraulic conductivities among the highest in the plant kingdom. Leaf conductance and maximum photosynthetic capacity scale directly with the hydraulic conductivity of the xylem (Hubbard et al., 2001; Tyree, 2003).
Thus, plantation Eucalyptus species, the most important of which are from the Symphyomyrtus subgenus and grow naturally in the wetter fringes of the Australian continent, have a higher maximum hydraulic conductivity, water use, and photosynthetic capacity than commercially grown Pinus species (Whitehead and Beadle, 2004). In the early growth phase, Symphyomyrtus Eucalyptus species also have a much higher specific leaf area (ratio of leaf area to mass) than Pinus, and this results in more rapid canopy development and the potential for faster early growth and water use, such as that observed by Scott and Lesch (1997). This can, of course, only happen if there is water available to support this growth and canopy development, and this can be supplied by rainfall throughout the year or by additional sources of water stored in deep soil (Dye and Olbrich, 1992; Scott and Lesch, 1997; White et al., 2014), shallow groundwater (Benyon et al., 2006; Brooksbank et al., 2011; Eamus et al., 2000; O'Grady et al., 2011), or applied as irrigation (Honeysett et al., 1996). If Eucalyptus plantations are grown on deep soils and in regions where the climate wetness index is much less than one (potential evaporation exceeds rainfall), then, by virtue of their hydraulic architecture, they have the potential to affect the water balance more than alternatives.
The capacity of Eucalyptus for high instantaneous sap velocities that are associated with elevated photosynthetic capacity also affects the seasonal patterns of water use in Eucalyptus compared to Pinus. Transpiration of Eucalyptus species increases rapidly in spring and is associated with high maximum stem and leaf conductivity (White et al., 1999). The relative behaviour of E. globulus and P. radiata is well understood, making them good exemplars. They are also two plantation species of great global importance that are grown in similar areas, including in central Chile. In Chile and Australia, P. radiata is known to be capable of surviving more severe droughts than E. globulus, and plantations of the species therefore extend into drier areas than E. globulus both in Chile and in Australia. The greater drought tolerance of P. radiata than E. globulus is mediated by a much stronger stomatal response to soil drying (Mitchell et al., 2014). In situations where the amount of soil water storage imposes an upper limit on annual use, although this store of water will be completely depleted by both species, it will then be used earlier in the growing season by Eucalyptus. Thus, the period of peak physiological activity and growth in Eucalyptus is associated with lower average temperatures and more moderate air saturation deficits. This pattern of water use, biased towards spring and early summer, can result in very efficient water use growth and wood production (White et al., 2015). This behaviour of the Eucalyptus is closer to a mimic of the seasonal water use pattern of an annual species. This mechanism underlies the greater water use efficiency of Eucalyptus species than of the Pinus but is also associated with an increased risk of mortality (White et al., 2003, 2009) if the soil water runs out. It also underlies the high rates of water use sometimes observed in deep soils (Scott and Lesch, 1997) and the high leverage in Model 3 of Eucalyptus grown on deep soil (see the Supplement).
At equilibrium, Eucalyptus and Pinus species generally have different seasonal patterns of water use. Nonetheless, the average annual water use does not differ significantly between the two genera amongst the published studies presented in Fig. 3. This observation is entirely consistent with the observed hydraulic architecture of these two genera in the field. Radiation interception and absorption, and therefore productivity and evapotranspiration in forests, including plantations, are strongly correlated with leaf area index. Battaglia et al. (1998) proposed that, after the canopy closes, plantations will arrive at an equilibrium leaf area index that maximises the net primary productivity. They further demonstrated that the value of this optimum leaf area index is strongly influenced by the climate wetness; higher optimum values of leaf area index were observed in wetter situations. The value of this optimum leaf area index tends to be higher for a given climate wetness in Pinus species than in Eucalyptus species. For those experiments included in this analysis that reported leaf area index, the average value for Pinus was approximately 4, which is nearly a full unit greater than the average value for the Eucalyptus plantations.
In comparing Eucalyptus and Pinus in commercial plantations, it is important to compare at least one, and possibly more, full crop rotation. Pinus is generally managed for solid wood production and therefore on a longer rotation than Eucalyptus, which is usually, but not exclusively, grown for pulpwood production. Around the world, the time from planting to harvest of Pinus species is between 2 and 3 times that of the Eucalyptus in the same location. In Chile, for example, Eucalyptus is harvested after about 12 years, while Pinus is grown for about 25 years. Pinus is usually grown for solid wood or veneer production and is therefore thinned at least once and is often pruned to produce clear wood. After the harvesting of the first Eucalyptus crop, a Pinus plantation on the same location would remain standing and operating at, or near, the water limit. For a period of between 2 and 3 years after the Eucalyptus harvest, the evapotranspiration of the Pinus will therefore exceed that of the Eucalyptus. This is evident in the results of Scott and Lesch (1997), who compared E. grandis with P. patula. The frequency of the harvest of Eucalyptus will be a key factor affecting the comparative water balance of Pinus and Eucalyptus plantations. Paradoxically, more frequent harvests will increase the average streamflow from Eucalyptus plantations relative to Pinus. It has been demonstrated that the effects of thinning on the water balance are transient, lasting for a maximum of 1 year in both Pinus and Eucalyptus (Scott and Lesch, 1997; White et al., 2014).
The proportion of evapotranspiration that occurs as transpiration was approximately 0.5 for both Pinus and Eucalyptus across a wide range of climate wetness indices (Fig. 6). This means that the annual partitioning of evapotranspiration to fluxes other than transpiration is similar for these two genera. The partitioning of these other fluxes to understorey transpiration, soil evaporation, and interception may have important implications for ecosystem productivity and efficiency. The water use efficiency of wood production is directly correlated with the ratio of transpiration to other fluxes (White et al., 2015). In a study that compared E. globulus and P. radiata, Benyon and Doody (2015) observed that interception was more than half the non-transpirational fluxes in P. radiata and less than half in E. globulus. This variation in partitioning is a direct consequence of the previously noted tendency for Pinus to have a higher leaf area index than Eucalyptus and the greater canopy storage per unit leaf area in Pinus than in broadleaved species (Iida et al., 2005). A weakness of this analysis and of the literature on water balance is the exclusion of stemflow from most water balance studies. It is likely that stemflow will contribute more to throughfall in Eucalyptus (7 % of rainfall) than in Pinus (2 % to 5 %; Crockford and Richardson, 1990). This difference is approximately equivalent in magnitude to the observed, albeit non-significant, difference between the genera in this analysis.
Water use by vegetation is very situation specific. The comparison between Eucalyptus and Pinus depends on the age of the plantation, the length of the rotation, the seasonality of rainfall, and the depth of the soil. In this paper, a meta-analysis of published estimates of evapotranspiration by Pinus and Eucalyptus species in commercial plantations did not find a significant difference between the genera. Specifically, while there was a small, but systematic, difference of about 3 % in water use between the genera (see Figs. 5 and 6), this analysis finds that, for a given climate wetness index, the evapotranspiration by Pinus and Eucalyptus was statistically the same. Moreover, our understanding of the hydraulic architecture and stomatal physiology of pines and eucalypts suggests that, although the long-term average behaviour may be similar, there will be differences in their temporal pattern of water use both within and between years. Eucalyptus will use more water than Pinus early in the growing season and in the early years of the rotation. On deep soils, this may result in lasting differences but under most circumstances the total effect on the water balance will be similar. The reputation of much higher water use by Eucalyptus may stem partly from the observation of vigorous early growth of Eucalyptus and the many studies on young plantation stands.
Codes and data sets are provided in the Supplement.
The supplement related to this article is available online at: https://doi.org/10.5194/hess-26-5357-2022-supplement.
DAW, SR, DM, FBC, RS, AI, and PRdA conceptualised the project. DAW and DM curated the data and did the formal analysis. DAW developed the methodology with PRdA. DAW validated the study with AI, and DAW prepared the original draft. DAW, DM, FBC, and RS reviewed and edited the paper. SR acquired the funding, and SR and PRdA supervised the project. PRdA administered the project.
The contact author has declared that none of the authors has any competing interests.
From July 2015 to April 2020, Don A. White and Richard P. Silberstein were paid to provide advice to Bioforest SA on Ecohydrology and Ecophysiology. Bioforest SA are an R and D company owned by Arauco, the largest plantation grower in central Chile. In the course of this work, Don A. White has also received some financial support from the Guangxi Forestry Research Institute in China.
Publisher's note: Copernicus Publications remains neutral with regard to jurisdictional claims in published maps and institutional affiliations.
The financial and in-kind support of Forestal Arauco in Chile and of the Guangxi Forestry Research Institute in Nanning, China, is acknowledged, as is the collaboration, fellowship, and hospitality of the people in these organisations and more widely in Chile and China. The contribution of Francisco Balocchi-Contreras has been supported by a doctoral scholarship from ANID-PFCHA/Doctorado Nacional (grant no. 2021-21210861). The final writing phase of this work was supported by the Forest Flows Programme, which is funded by the New Zealand Ministry for Business, Innovation, and Employment Endeavour Fund (grant no. C04X1905).
The contribution of Francisco Balocchi-Contreras has been supported by a doctoral scholarship from ANIDPFCHA/Doctorado Nacional (grant no. 2021-21210861). The final writing phase of this work has been supported by the Forest Flows Programme, which is funded by the New Zealand Ministry for Business, Innovation, and Employment Endeavour Fund (grant no. C04X1905).
This paper was edited by Adriaan J. (Ryan) Teuling and reviewed by Michael Ryan and one anonymous referee.
Albaugh, J., Dye, P., and King, J.: Eucalyptus and Water Use in South Africa, Int. J. Forest. Res., 2013, 852540, https://doi.org/10.1155/2013/852540, 2013.
Allen, R. G., Pereira, L. S., Smith, M., Raes, D., and Wright, J. L.: FAO-56 Dual Crop Coefficient Method for Estimating Evaporation from Soil and Application Extensions, J. Irrig. Drain. Eng., 131, 2–13, https://doi.org/10.1061/(asce)0733-9437(2005)131:1(2), 2005.
Almeida, A., Soares, J., Landsberg, J., and Rezende, G.: Growth and water balance of a Eucalyptus grandis hybrid plantation in Brazil during a rotation for pulp production, Forest Ecol. Manage., 251, 10–21, 2007.
Almeida, A. C., Smethurst, P. J., Siggins, A., Cavalcante, R. B. L., and Borges, N.: Quantifying the effects of Eucalyptus plantations and management on water resources at plot and catchment scales, Hydrol. Process., 30, 4687–4703, https://doi.org/10.1002/hyp.10992, 2016.
Amatya, D. M., Miwa, M., Harrison, C. A., Trettin, C. C., and Sun, G.: Hydrology and Water Quality of Two First Order Forested Watersheds in Coastal South Carolina, St. Joseph, MI, https://doi.org/10.13031/2013.21436, 2006.
Aubinet, M., Vesala, T., and Papale, D.: Eddy Covariance. A Practical Guide to Measurement and Data Analysis, Springer Verlag, London, https://doi.org/10.1007/978-94-007-2351-1, 2012.
Balocchi, F., White, D. A., Silberstein, R. P., and Ramírez de Arellano, P.: Forestal Arauco experimental research catchments; daily rainfall-runoff for 10 catchments with different forest types in Central-Southern Chile, Hydrol. Process., 35, e14047, https://doi.org/10.1002/hyp.14047, 2021.
Battaglia, M., Cherry, M. L., Beadle, C. L., Sands, P. J., and Hingston, A.: Prediction of leaf area index in eucalypt plantaions: effects of water stress and temperature, Tree Physiol., 18, 521–528, 1998.
Beets, P. N. and Oliver, G. R.: Water Use by Managed Stands of Pinus radiata, Indigenous Podocarp/Hardwood Forest, and Improved Pasture in the Central North Island of New Zealand, NZ J. Forest Sci., 37, 308–323, 2006.
Benyon, R. G. and Doody, T. M.: Comparison of interception, forest floor evaporation and transpiration in Pinus radiata and Eucalyptus globulus plantations, Hydrol. Process., 29, 1173–1187, https://doi.org/10.1002/hyp.10237, 2015.
Benyon, R. G., Theiveyanathan, S., and Doody, T. M.: Impacts of tree plantations on groundwater in south-eastern Australia, Aust. J. Bot., 54, 181–192, https://doi.org/10.1071/bt05046, 2006.
Bren, L. and Hopmans, P.: Paired catchments observations on the water yield of mature eucalypt and immature radiata pine plantations in Victoria, Australia, J. Hydrol., 336, 416–429, 2007.
Brooksbank, K., Veneklaas, E. J., White, D. A., and Carter, J. L.: Water availability determines hydrological impact of tree belts in dryland cropping systems, Agr. Water Manage., 100, 76–83, https://doi.org/10.1016/j.agwat.2011.08.016, 2011.
Bubb, K. A. and Croton, J. A.: Effects on catchment water balance from the management of Pinus plantations on the coastal lowlands of south-east Queensland, Australia, Hydrol. Process., 16, 105–117, 2002.
Budyko, M. I.: Climate and life, Academic Press, New York, ISBN 10:0121394506, ISBN 13:978-0121394509, 1974.
Crockford, R. H. and Richardson, D. P.: Partitioning of rainfall in a eucalypt forest and pine plantation in southeastern australia: II stemflow and factors affecting stemflow in a dry sclerophyll eucalypt forest and a pinus radiata plantation, Hydrol. Process., 4, 145–155, https://doi.org/10.1002/hyp.3360040205, 1990.
David, J. S., Henriques, M. O., David, T. S., Tomé, J., and Ledger, D. C.: Clearcutting effects on streamflow in coppiced Eucalyptus globulus stands in Portugal, J. Hydrol., 162, 143–154, https://doi.org/10.1016/0022-1694(94)90008-6, 1994.
Dye, P. J.: Response of Eucalyptus grandis trees to soil water deficit, Tree Physiol., 16, 233–238, 1996.
Dye, P. J. and Olbrich, B. W.: Heat pulse observations of Eucalyptus grandis transpiration in South Africa, in: Growth and Water Use of Forest Plantations, edited by: Calder, I. R., Hall, R. L., and Adlard, P. G., Wiley & Sons, Chichester, 216–225, CONF-9102202, 1992.
Eamus, D., O'Grady, A. P., and Hutley, L.: Dry season conditions determine wet season water use in the wet-dry tropical savannas of northern Australia, Tree Physiol., 20, 1219–1226, 2000.
Eichinger, W. E., Parlange, M. B., and Stricker, H.: On the Concept of Equilibrium Evaporation and the Value of the Priestley-Taylor Coefficient, Water Resour. Res., 32, 161–164, https://doi.org/10.1029/95WR02920, 1996.
Ford, C. R., Hubbard, R. M., Kloeppel, B. D., and Vose, J. M.: A comparison of sap flux-based evapotranspiration estimates with catchment-scale water balance, Agr. Forest Meteorol., 145, 176-185, https://doi.org/10.1016/j.agrformet.2007.04.010, 2007.
Gholz, H. L. and Clark, K. L.: Energy exchange across a chronosequence of slash pine forests in Florida, Agr. Forest Meteorol., 112, 87–102, 2002.
Greenwood, A.: The first stages of Australian forest water regulation: National reform and regional implementation, Environ. Sci. Policy, 29, 124–136, https://doi.org/10.1016/j.envsci.2013.01.012, 2013.
Hargreaves, G. and Samani, Z.: Reference crop evapotranspiration from temperature, Appl. Eng. Agricult., 1, 96–99, 1985.
Harris, I., Osborn, T. J., Jones, P., and Lister, D.: Version 4 of the CRU TS monthly high-resolution gridded multivariate climate dataset, Scient. Data, 7, 109, https://doi.org/10.1038/s41597-020-0453-3, 2020.
Honeysett, J. L., White, D. A., Worledge, D., and Beadle, C. L.: Growth and water use of Eucalyptus globulus and E. nitens in irrigated and rainfed plantations, Aust. Forest., 59, 64–73, 1996.
Hubbard, R. M., Ryan, M. G., Stiller, V., and Sperry, J. S.: Stomatal conductance and photosynthesis vary linearly with plant hydraulic conductance in ponderosa pine, Plant Cell Environ., 24, 113–121, https://doi.org/10.1046/j.1365-3040.2001.00660.x, 2001.
Huber, A. and Iroumé, A.: Variation of annual rainfall partitioning for different sites and forest covers in Chile, J. Hydrol., 248, 78–92, 2001.
Huber, A., Iroumé, A., and Bathurst, J.: Effect of Pinus radiata plantations on water balance in Chile, Hydrol. Process., 22, 142–148, https://doi.org/10.1002/hyp.6582, 2008.
Iida, S. i., Tanaka, T., and Sugita, M.: Change of interception process due to the succession from Japanese red pine to evergreen oak, J. Hydrol., 315, 154–166, https://doi.org/10.1016/j.jhydrol.2005.03.024, 2005.
Iroumé, A., Jones, J., and Bathurst, J. C.: Forest operations, tree species composition and decline in rainfall explain runoff changes in the Nacimiento experimental catchments, south central Chile, Hydrol. Process., 35, e14257, https://doi.org/10.1002/hyp.14257, 2021.
Kanninen, M.: Plantation Forests: Global Perspectives, in: Ecosystem Goods and Services from Plantation Forests, edited by: Bauhus, J., Meer, P., and Kanninen, M., Routledge, London, 1–15, https://doi.org/10.4324/9781849776417, 2010.
Keenan, R. J., Reams, G. A., Achard, F., de Freitas, J. V., Grainger, A., and Lindquist, E.: Dynamics of global forest area: Results from the FAO Global Forest Resources Assessment 2015, Forest Ecol. Manage., 352, 9–20, https://doi.org/10.1016/j.foreco.2015.06.014, 2015.
Komatsu, T. S.: Toward a robust phenomenological expression of evaporation efficiency for unsaturated soil surfaces, J. Appl. Meteorol., 42, 1330–1334, 2003.
Lane, P. N. J., Morris, J., Ningnan, Z., Guangyi, Z., Guoyi, Z., and Daping, X.: Water balance of tropical eucalypt plantations in south-eastern China, Agr. Forest Meteorol., 124, 253–267, 2004.
Lesch, W. and Scott, D. F.: The response in water yield to the thinning of Pinus radiata, Pinus patula and Eucalyptus grandis plantations, Forest Ecol. Manage., 99, 295–307, 1997.
Lima, W. P., Zakia, M. J. B., Libardi, P. L., and Souz a Filho, A. P.: Comparative evapotranspiration of eucalyptus, pine and natural cerrado vegetation measured by the soil water balance method, IPEF Int., 1, 5–11, 1990.
Little, C., Lara, A., McPhee, J., and Urrutia, R.: Revealing the impact of forest exotic plantations on water yield in large scale watersheds in South-Central Chile, J. Hydrol., 374, 162–170, https://doi.org/10.1016/j.jhydrol.2009.06.011, 2009.
MacDicken, K., Jonsson, Ö., Piña, L., Maulo, S., Contessa, V., Adikari, Y., Garzuglia, M., Lindquist, E., Reams, G., and D'Annunzio, R.: Global Forest Resources Assessment 2015: how are the world's forests changing?, http://www.fao.org/3/a-i4793e.pdf (last access: 26 October 2022), 2016.
McDonnell, J.: Beyond the water balance, Nat. Geosci., 10, 396–396, 2017.
Meinzer, F. C., Goldstein, G., Franco, A. C., Bustamante, M., Igler, E., Jackson, P., Caldas, L., and Rundel, P. W.: Atmospheric and hydraulic limitations on transpiration in Brazilian cerrado woody species, Funct. Ecol., 13, 273–282, 1999.
Mendham, D. S., White, D. A., Battaglia, M., McGrath, J. F., Short, T. M., Ogden, G. N., and Kinal, J.: Soil water depletion and replenishment during first- and early second-rotation Eucalyptus globulus plantations with deep soil profiles, Agr. Forest Meteorol., 151, 1568–1579, https://doi.org/10.1016/j.agrformet.2011.06.014, 2011.
Merlin, O., Stefan, V. G., Amazirh, A., Chanzy, A., Ceschia, E., Er-Raki, S., Gentine, P., Tallec, T., Ezzahar, J., and Bircher, S.: Modeling soil evaporation efficiency in a range of soil and atmospheric conditions using a meta-analysis approach, Water Resour. Res., 52, 3663–3684, 2016.
Mitchell, P. J., O'Grady, A. P., Tissue, D. T., Worledge, D., and Pinkard, E. A.: Co-ordination of growth, gas exchange and hydraulics define the carbon safety margin in tree species with contrasting drought strategies, Tree Physiol., 34, 443–458, https://doi.org/10.1093/treephys/tpu014, 2014.
Monteith, J. and Unsworth, M.: Principles of Environmental Physics: Plants, Animals, and the Atmosphere, 4th Edn., Elsevier, Amsterdam, the Netherlands, 401 pp, https://doi.org/10.1016/B978-0-12-386910-4.00013-5, 2013.
Myers, B. A., Benyon, R. G., Theiveyanathan, S., Criddle, R. S., Smith, C. J., and Falkner, R. A.: Response of effluent-irrigated Eucalyptus grandis and Pinus radiata to salinity and vapor pressure deficits, Tree Physiol., 18, 565–573, 1998.
O'Grady, A. P., Carter, J. L., and Bruce, J.: Can we predict groundwater discharge from terrestrial ecosystems using existing eco-hydrological concepts?, Hydrol. Earth Syst. Sci., 15, 3731–3739, https://doi.org/10.5194/hess-15-3731-2011, 2011.
Payn, T., Carnus, J.-M., Freer-Smith, P., Kimberley, M., Kollert, W., Liu, S., Orazio, C., Rodriguez, L., Silva, L. N., and Wingfield, M. J.: Changes in planted forests and future global implications, Forest Ecol. Manage., 352, 57–67, https://doi.org/10.1016/j.foreco.2015.06.021, 2015.
Penman, H. L.: The dependance of transpiration on weather and soil conditions, J. Soil Sci., 1, 74–89, 1949.
Pfautsch, S., Harbusch, M., Wesolowski, A., Smith, R., Macfarlane, C., Tjoelker, M. G., Reich, P. B., and Adams, M. A.: Climate determines vascular traits in the ecologically diverse genus Eucalyptus, Ecol. Lett., 19, 240–248, https://doi.org/10.1111/ele.12559, 2016.
Powell, T. L., Starr, G., Clark, K. L., Martin, T. A., and Gholz, H. L.: Ecosystem and understory water and energy exchange for a mature, naturally regenerated pine flatwoods forest in north Florida, Can. J. Forest Res., 35, 1568–1580, https://doi.org/10.1139/x05-075, 2005.
Priestley, C. H. B. and Taylor, R. J.: On the assessment of surface heat flux and evaporation using large scale parameters, Mon. Weather Rev., 100, 81–92, 1972.
Putuhena, W. M. and Cordery, I.: Some hydrological effects of changing forest cover from eucalypts to Pinus radiata, Agr. Forest Meteorol., 100, 59–72, https://doi.org/10.1016/S0168-1923(99)00086-6, 2000.
R-Core-Team: R: A language and environment for statistical computing, R Foundation for Statistical Computing, R-Core-Team, https://www.R-project.org/ (last access: 26 October 2022), 2013.
Reichert, J. M., Rodrigues, M. F., Peláez, J. J. Z., Lanza, R., Minella, J. P. G., Arnold, J. G., and Cavalcante, R. B. L.: Water balance in paired watersheds with eucalyptus and degraded grassland in Pampa biome, Agr. Forest Meteorol., 237–238, 282–295, https://doi.org/10.1016/j.agrformet.2017.02.014, 2017.
Ren, S., White, D. A., Xiang, D., Short, T. M., Xiao, W., Chen, J., Deng, Z., and Yang, Z.: Simple model of evapotranspiration by Eucalyptus plantations for data poor areas and tested using water balance data from a small catchment in Guangxi, China, Aust. Forest., 82, 66–79, https://doi.org/10.1080/00049158.2018.1555733, 2019.
Roberts, S., Barton-Johnson, R., McLarin, M., and Read, S.: Predicting the water use of Eucalyptus nitens plantation sites in Tasmania from inventory data, and incorporation of water use into a forest estate model, Forest Ecol. Manage., 343, 110–122, https://doi.org/10.1016/j.foreco.2015.01.025, 2015.
Rodriguez Suarez, J. A., Diaz-Fierros, F., Perez, R., and Soto, B.: Assessing the influence of afforestation withEucalyptus globuluson hydrological response from a small catchment in northwestern Spain using the HBV hydrological model, Hydrol. Process., 28, 5561–5572, https://doi.org/10.1002/hyp.10061, 2014.
Samraj, P., Sharda, V. N., Chinnamani, S., Lakshmanan, V., and Haldorai, B.: Hydrological behaviour of the Nilgiri sub-watersheds as affected by bluegum plantations, part I. The annual water balance, J. Hydrol., 103, 335–345, https://doi.org/10.1016/0022-1694(88)90142-4, 1988.
Scott, D. F. and Lesch, W.: Streamflow responses to afforestation with Eucalyptus grandis and Pinus patula and to felling in the Mokobulaan experimental catchments, South Africa, J. Hydrol., 199, 360–377, 1997.
Shi, Z., Xu, D., Yang, X., Jia, Z., Guo, H., and Zhang, N.: Ecohydrological impacts of eucalypt plantations: A review, J. Food Agricult. Environ., 10, 1418–1426, 2012.
Soares, J. V. and Almeida, A. C.: Modeling the water balance and soil water fluxes in a fast growing Eucalyptus plantation in Brazil, J. Hydrol., 253, 130–147, 2001.
Sperry, J. S., Hacke, U. G., and Pittermann, J.: Size and function in conifer tracheids and angiosperm vessels, Am. J. Bot., 93, 1490–1500, https://doi.org/10.3732/ajb.93.10.1490, 2006.
Stoy, P. C., Katul, G. G., Siqueria, M. B. S., Juang, J.-Y., Novick, K. A., McCarthy, H. R., Christopher Oishi, A., Uebelherr, J. M., Kim, H.-S., and Oren, R.: Separating the effects of climate and vegetation on evapotranspiration along a successional chronosequence in the southeastern US, Global Change Biol., 12, 2115–2135, https://doi.org/10.1111/j.1365-2486.2006.01244.x, 2006.
Sun, G., Noormets, A., Gavazzi, M. J., McNulty, S. G., Chen, J., Domec, J. C., King, J. S., Amatya, D. M., and Skaggs, R. W.: Energy and water balance of two contrasting loblolly pine plantations on the lower coastal plain of North Carolina, USA, Forest Ecol. Manage., 259, 1299–1310, https://doi.org/10.1016/j.foreco.2009.09.016, 2010.
Teskey, R. O. and Sheriff, D. W.: Water use by Pinus radiata trees in a plantation, Tree Physiol., 16, 273–279, 1996.
Tyree, M. T.: Hydraulic limits on tree performance: transpiration, carbon gain and growth of trees, Trees, 17, 95–100, https://doi.org/10.1007/s00468-002-0227-x, 2003.
White, D. A., Beadle, C. L., Sands, P. J., Worledge, D., and Honeysett, J. L.: Quantifying the effect of cumulative water stress on stomatal conductance of Eucalyptus globulus and E. nitens: a phenomenological approach, Aust. J. Plant Physiol., 26, 17–27, 1999.
White, D. A., Battaglia, M., Macfarlane, C., Mummery, D., McGrath, J. F., and Beadle, C. L.: Selecting species for recharge management in Mediterranean south western Australia – some ecophysiological considerations, Plant Soil, 257, 283–293, 2003.
White, D. A., Crombie, D. S., Kinal, J., Battaglia, M., McGrath, J. F., Mendharn, D. S., and Walker, S. N.: Managing productivity and drought risk in Eucalyptus globulus plantations in south-western Australia, Forest Ecol. Manage., 259, 33–44, https://doi.org/10.1016/j.foreco.2009.09.039, 2009.
White, D. A., McGrath, J. F., Ryan, M. G., Battaglia, M., Mendham, D. S., Kinal, J., Downes, G. M., Crombie, D. S., and Hunt, M. A.: Managing for water-use efficient wood production in Eucalyptus globulus plantations, Forest Ecol. Manage., 331, 272–280, https://doi.org/10.1016/j.foreco.2014.08.020, 2014.
White, D. A., Beadle, C. L., Worledge, D., and Honeysett, J. L.: Wood production per evapotranspiration was increased by irrigation in plantations of Eucalyptus globulus and E. nitens, New Forests, 47, 303–317, https://doi.org/10.1007/s11056-015-9516-2, 2015.
White, D. A., Battaglia, M., Ren, S., and Mendham, D. S.: Water use and water productivity of Eucalyptus plantations in South-East Asia, Australian Centre For International Agricultural Research, Canberra, Australia, 55 pp., https://www.aciar.gov.au/sites/default/files/legacy/tr_89-web.pdf (last access: 26 October 2022), 2016.
White, D. A., Silberstein, R. P., Balocchi-Contreras, F., Quiroga, J. J., Meason, D. F., Palma, J. H. N., and Ramírez de Arellano, P.: Growth, water use, and water use efficiency of Eucalyptus globulus and Pinus radiata plantations compared with natural stands of Roble-Hualo forest in the coastal mountains of central Chile, Forest Ecol. Manage., 501, 119676, https://doi.org/10.1016/j.foreco.2021.119676, 2021.
Whitehead, D. and Beadle, C. L.: Physiological regulation of productivity and water use in Eucalyptus: a review, Forest Ecol. Manage., 193, 113–140, https://doi.org/10.1016/j.foreco.2004.01.026, 2004.
Wilson, K. B., Hanson, P. J., Mulholland, P. J., Baldocchi, D. D., and Wullschleger, S. D.: A comparison of methods for determining forest evpotranspiration and its components: sap-flow, soil water budget, eddy covariance and catchment water balance, Agr. Forest Meteorol., 106, 153–168, 2001.
Zhang, J.: Risk assessment of drought disaster in the maize-growing region of Songliao Plain, China, Agricult. Ecosyst. Environ., 102, 133–153, 2004.
Zhang, L., Dawes, W. R., and Walker, G. R.: The response of mean annual evapotranspiration to vegetation changes at the catchment scale, Water Resour. Res., 37, 701–708, 2001.
Zhang, L., Hickel, K., Dawes, W. R., Chiew, F. H. S., Western, A. W., and Briggs, P. R.: A rational function approach for estimating mean annual evapotranspiration, Water Resour. Res., 40, W02502, https://doi.org/10.1029/2003WR002710, 2004.