the Creative Commons Attribution 4.0 License.
the Creative Commons Attribution 4.0 License.
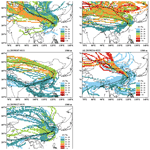
Water vapor isotopes indicating rapid shift among multiple moisture sources for the 2018–2019 winter extreme precipitation events in southeastern China
Tao Xu
Hongxi Pang
Zhaojun Zhan
Wangbin Zhang
Huiwen Guo
Shuangye Wu
In the East Asian monsoon region, winter extreme precipitation events occasionally occur and bring great social and economic losses. From December 2018 to February 2019, southeastern China experienced a record-breaking number of extreme precipitation events. In this study, we analyzed the variation in water vapor isotopes and their controlling factors during the extreme precipitation events in Nanjing, southeastern China. The results show that the variations in water vapor isotopes are closely linked to the change in moisture sources. Using a water vapor d-excess-weighted trajectory model, we identified the following five most important moisture source regions: South China, the East China Sea, the South China Sea, the Bay of Bengal, and continental regions (northwestern China and Mongolia). Moreover, the variations in water vapor d excess during a precipitation event reflect rapid shifts in the moisture source regions. These results indicate that rapid shifts among multiple moisture sources are important conditions for sustaining wintertime extreme precipitation events over extended periods.
- Article
(4606 KB) - Full-text XML
- BibTeX
- EndNote
South China is under the influence of the East Asian Winter Monsoon during winter, with a prevailing northeasterly wind. As a result, winter precipitation is significantly less than in summer (Yao et al., 2015), accounting for approximately 10 % of the total annual precipitation (Wang and Feng, 2011). However, persistent extreme winter precipitation events still occur occasionally (Huang et al., 2018a; X. Li et al., 2020a), such as the disastrous snowstorm in January 2008 (Ding et al., 2008), which caused great losses in agriculture, transportation, and electric power facilities (Zhou et al., 2011). Such extreme events are likely to become more frequent with global warming (Rahmostorf and Coumou, 2011; Qin et al., 2021). Therefore, improving the understanding of the characteristics, controlling factors, and moisture sources of winter extreme precipitation events over South China could have great scientific as well as economic significance.
It is well known that extreme weather events are often caused by a combination of anomalous conditions in multiple atmospheric circulation systems (Li et al., 2019). Previous studies attributed the winter extreme precipitation events over South China to anomalies in the western Pacific subtropical high, the East Asian major trough, the India–Myanmar trough, and the formation of the European blocking high (Wang et al., 2000; Zong et al., 2014; Ding and Li, 2017; Huang et al., 2018b). In addition, the variation in winter precipitation was also found to be closely related to sea surface temperature over the eastern tropical Indian Ocean and the South China Sea (Zhou et al., 2010; X. Li et al., 2015). In addition to dynamic and thermal conditions, abundant moisture supply is also an essential condition for winter extreme precipitation events (Yang et al., 2019; Zhao et al., 2021). Therefore, exploring the moisture sources for the extreme precipitation events is a crucial part of understanding factors controlling these events. Previous climatological studies suggest that the most important moisture source regions for wintertime precipitation in South China were the South China Sea, western North Pacific, and the Bay of Bengal (Baker et al., 2015; Sun and Wang, 2015). However, detailed information about moisture transport and source regions of individual extreme precipitation events is largely lacking. Moreover, the large-scale atmospheric circulation patterns are not changing significantly at short timescales, so it is difficult for climatological studies to capture the possible rapid switching of moisture sources during an extreme precipitation event.
Stable isotopes in precipitation and water vapor (δ18O and δ2H) are useful natural tracers for the hydrological cycle (Araguás-Araguás et al., 2000; Galewsky et al., 2016). They have been used to trace moisture sources (Bonne et al., 2014; He et al., 2016) and identify water vapor transport pathways (Tian et al., 2007; Cai and Tian, 2016). Earlier studies have improved our understanding of the variations in stable isotopic compositions at different timescales, especially at the monthly and daily scales. The variability in stable isotopic compositions is not only affected by local meteorological factors, such as temperature and precipitation amount (Dansgaard, 1964; Yu et al., 2015), but also by large-scale atmospheric circulations (Zhou and Li, 2017; Gao et al., 2018) and sources of moisture (Peng et al., 2010; Wang et al., 2017). In addition, deuterium excess (d excess), defined as , is influenced by the evaporative and meteorological conditions in the moisture source region (Dansgaard, 1964; Merlivat and Jouzel, 1979) and is often used as an indicator for changes in relative humidity at the source region or shifts in the moisture origin (Benetti et al., 2014). In addition to monthly and daily scales, precipitation and water vapor isotopes have also been used to study precipitation processes at the event scale. For instance, Tremoy et al. (2014) used high-frequency, near-surface water vapor isotopic composition measurements to classify precipitation events in the Niamey region in Niger into three categories and established the role of mesoscale subsidence and rain evaporation on the isotopic evolution. J. Li et al. (2015) revealed the abundant water vapor supply from different moisture sources through the continuous isotopic observation of a summer extreme precipitation event in Beijing. Han et al. (2020), based on a data set with a 10 min sampling interval, showed that the rapid change in stable isotopes in precipitation is related to different moisture sources and transport paths in the marginal zone of the East Asian monsoon. These studies demonstrated that high-resolution precipitation and/or water vapor isotopes observations are an effective tool for identifying more detailed information about precipitation processes. However, observations of precipitation and/or water vapor isotopes during an extreme precipitation event is sparse, which limits our ability to study extreme precipitation processes by water isotopes.
From December 2018 to February 2019, the joint influence of the central Pacific El Niño and East Asian winter monsoon quasi-biweekly oscillation led to a long period of persistent rainfall in southeastern China. The 51 accumulated rainy days had the highest regional effective precipitation since 1981 (Guo et al., 2019; Wang et al., 2020). The regional sunshine duration was only half of the normal conditions, with the lowest number of sunshine hours recorded since 1961 (X. Li et al., 2020b). This record-breaking event provided an opportunity for using stable isotopes to understand the possible causes and moisture sources of winter extreme precipitation. In this study, we monitored and analyzed the stable isotopic compositions of water vapor (with hourly resolution) and precipitation (with daily resolution) during this extreme precipitation period in Nanjing, southeastern China. We utilized these high temporal resolution data to investigate the evolution of the stable isotopic compositions in water vapor during this period and understand how event-scale isotopic variation is affected by climate factors and moisture sources during winter extreme precipitation events.
2.1 Study site
Sampling and measurement of stable isotopes in water vapor and precipitation were carried out at the Station for Observing Regional Processes of the Earth System of Nanjing University (SORPES-NJU; 32.12∘ N, 118.95∘ E; 55 m a.s.l. – above sea level) at the Xianlin Campus of Nanjing University, about 20 km east of downtown Nanjing in southeastern China (Y. Li et al., 2020).
Nanjing is located in the lower reaches of the Yangtze river in China and has a typical subtropical monsoon climate. Under the influence of the East Asian monsoon, it has a strongly seasonal climate, with hot and rainy summers and cold and dry winters. In the winter (December–February – DJF), Nanjing is under the influence of the East Asian winter monsoon. From December 2018 to February 2019, affected by the continuous rainy weather, Nanjing has 46 accumulated rainy days.
2.2 Sampling and measurement
Water vapor sampling was carried out on the roof of the SORPES-NJU double-story building from 1 November 2012 to the present. Data from December 2018 to February 2019 were used for this study. The stable isotopic compositions of water vapor ( and ) were measured by a Picarro wavelength-scanned cavity ring-down spectrometer (WS-CRDS; model – Picarro L2120–i). We calibrated the samples against various deviations (such as memory effect, drift effect, and concentration effect), following a set of procedures described in Gu et al. (2019) and Y. Li et al. (2020). We collected one sample every 2–3 s and summarized the raw data into hourly averages for this study. The instrumental uncertainties were less than 0.2 ‰ for and 1.0 ‰ for (Gu et al., 2019).
Along with regular water vapor sampling, precipitation samples were collected on rainy days with an amount of >0.1 mm from September 2011 to the present. Data from December 2018 to February 2019 were used for this study. All samples were poured into 100 mL polyethylene bottles immediately after collection. In order to avoid evaporation, all samples were refrigerated until being analyzed. The stable isotopic compositions in precipitation ( and ) were measured by a Picarro L2120–i in the Key Laboratory of Coast and Island Development of the Ministry of Education at Nanjing University, China. The precision of the measurements is 0.05 ‰ for and 0.5 ‰ for (Tang et al., 2015).
2.3 Trajectory-weighted concentration field
In order to trace the moisture source, we used the National Oceanic and Atmospheric Administration (NOAA) Hybrid Single-Particle Lagrangian Integrated Trajectory (HYSPLIT) model and calculated the backward trajectories of air masses associated with individual extreme precipitation events, using the Global Data Assimilation System (GDAS) with a spatial resolution of as the background meteorological data (ftp://ftp.arl.noaa.gov/pub/archives/gdas1; last access: 20 August 2020). The 8 d backward trajectories were calculated every 1 h with the starting height of 1500 m above the ground because the average residence time of water vapor in the atmosphere is about 8 to 10 d (van der Ent and Tuinenburg, 2017).
Based on the HYSPLIT results, we calculated the concentration-weighted trajectory (CWT) field at resolution to establish potential source regions that influence the isotopic variability in water vapor at the study site (Salamalikis et al., 2015; Bedaso and Wu, 2020; Y. Li et al., 2020). The CWT (Cij) was calculated with the following equation:
where (i,j) are grid indices, k is the trajectory index, K is the total number of trajectories that pass each grid, Ck is the concentration (d excess) measured upon arrival of trajectory k, and τijk is the residence time of trajectory k in grid cell (i,j).
2.4 Meteorological and reanalysis data
We obtained long-term monthly mean (1981–2010) and hourly meteorological data (air temperature, precipitation amount, and relative humidity) from December 2018 to February 2019 at the Nanjing meteorological station from the China Meteorological Data Service Centre. We also used ERA5 hourly data () from December 2018 to February 2019, including relative humidity, specific humidity, temperature, and zonal and meridional wind components from the European Centre for Medium-Range Weather Forecasts (ECMWF) Copernicus Climate Data Store (https://cds.climate.copernicus.eu; last access: 24 December 2020) and monthly Global Precipitation Climatology Centre (GPCC) precipitation data () from the NOAA Physical Sciences Laboratory (https://psl.noaa.gov/data/gridded/, last access: 22 January 2021).
Unless explicitly noted, the anomalies for climate variables are calculated with respect to the average of 1981–2010.
2.5 Definition of the 2018–2019 winter extreme precipitation in southeastern China
In the 2018–2019 winter, the regional average cumulative effective precipitation days in southeastern China exceeded 51 d, breaking the historical record that had been in place since 1981 (Guo et al., 2019). In Nanjing, where our research site is located, the seasonal average DJF precipitation of 1981–2010 is 126 mm. Accumulated precipitation is 259 mm in the 2018–2019 winter, which is more than double the seasonal average of 1981–2010 (Fig. 1). As a result, we defined the 2018–2019 winter as a typical long-term extreme precipitation period. Because some water vapor isotopic data at Nanjing were missing due to instrument repair or maintenance, five large-scale precipitation events were finally selected for analysis, including (a) 4–11 December, (b) 24–30 December 2018, (c) 7–11 January, (d) 16–22 February, and (e) 27–31 January 2019.
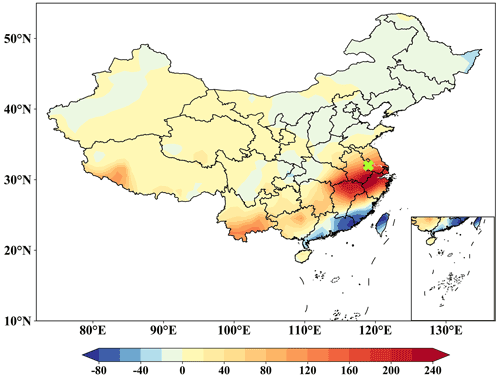
Figure 1Winter (December–February) precipitation anomalies (shading; units in millimeters) over China in the winter of 2018–2019. The cross symbols (×) represent the sampling site at Nanjing. The anomalies were calculated with respect to the 1981–2010 climatology (precipitation data from the Global Precipitation Climatology Centre).
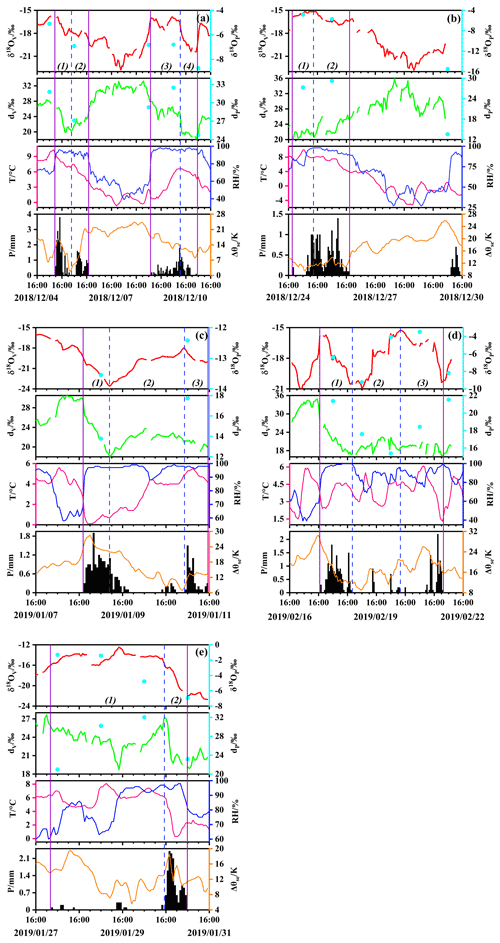
Figure 2Time series of hourly water vapor ( and dv), daily precipitation ( and dp; cyan dots), air temperature (T), relative humidity (RH), precipitation amount (P), and atmospheric stability (Δθse) at Nanjing. Atmospheric stability is the difference between the pseudo-equivalent potential temperature of 700 and 850 hPa (). (a) The event on 4–11 December 2018. (b) The event on 24–30 December 2018. (c) The event on 7–11 January 2019. (d) The event on 16–22 February 2019. (e) The event on 27–31 January 2019. The vertical purple lines and blue dashed lines indicate the concentrated rainfall period and different stages of each precipitation event, respectively.
Figure 2 shows the hourly average and dv (water vapor d excess), daily and dp (precipitation d-excess), and hourly air temperature, relative humidity, precipitation amount, and atmospheric stability for those five extreme precipitation events. varies from −23.6 ‰ to −12.4 ‰ (with an average of −18.1 ‰) and dv ranges from 16.3 ‰ to 35.9 ‰ (with an average of 24.6 ‰). has a range from −15.5 ‰ to −1.3 ‰ and an average of −7.0 ‰. dp ranges from 13.5 ‰ to 32.5 ‰, with an average of 23.6 ‰. Stable isotopes in precipitation and water vapor have a similar variation pattern. Therefore, only high temporal resolution water vapor isotope data are used for further analysis. Based on the large-scale atmospheric circulation patterns (Fig. 3), we group these precipitation events into three classes.
The first class (including events a and b) is defined as cold-air-mass-dominated events. The beginning of the precipitation event was characterized by the southerly wind and higher temperature (Fig. 3a and b), and the atmospheric stability was low (Fig. 2a and b). With the invasion of the cold air mass through the majority of the event period, the study site experienced a northerly wind and a temperature decrease, and the atmospheric stability was gradually increasing (Fig. 2a and b). Towards the end of the event, the site returned to a southerly wind with a temperature increase, and the atmospheric stability decreased. Under this circulation background, the value was generally high at the beginning, decreased significantly during the events, and gradually increased again toward the end of the events, whereas the dv value showed the opposite trends (Fig. 2a and b).
The second class (events c and d) is defined as warm-air-mass-dominated events. Northerly wind and low temperature occurred only at the beginning of the precipitation event, whereas the majority of the event period was characterized by a southerly wind and a warm temperature (Fig. 3c and d). The atmospheric stability was relatively high at the beginning but soon decreased and remained low throughout the events (Fig. 2c and d). Similar to the first class, the value was generally high at the beginning, decreased significantly during the events, and gradually increased again toward the end of the events (Fig. 2c and d). However, different from the first class, both and dv values in this class showed changes in the same direction throughout the event (Fig. 2c and d).
In addition to the above two classes, the third class (event e) is characterized by alternating cold and warm air masses. The event started with a northerly wind and a low temperature, followed by a southerly wind and a temperature increase, and ended with a northerly wind and a temperature decrease (Fig. 3e). The atmospheric stability was relatively high at the beginning, gradually decreased, and then increased in the later stage (Fig. 2e). The value remained constant in the early stage, until it decreased suddenly at the end, whereas the dv value showed great fluctuations (Fig. 2e).
4.1 Controlling factors for water vapor isotopic variations during precipitation events
Significant variations in and dv in water vapor are observed within each event. In order to explore the controlling factors for the isotopic variations, we divided the concentrated rainfall period of each event into different stages (Fig. 2) based on temporal variation patterns of and dv.
The first class (Fig. 2a and b) of the precipitation events can be divided into four stages (the last stage of event b is not delineated because of missing data). In stages 1 and 2, the value continued to decrease with decreasing temperature due to the temperature effect, i.e., the isotopic composition has a significant positive correlation with air temperature. The dv value first decreased (or remained stable) and then increased, suggesting a gradual shift of water vapor source from ocean to land (Fig. 2a and b). Generally, atmospheric water vapor from the dry and cold regions shows a more negative δ18O value and a relatively high d-excess value (Uemura et al., 2008; Kostrova et al., 2020). In stage 3, the and dv values fluctuated without any obvious trends as a whole, mainly due to the mixing of oceanic and inland water vapor. In stage 4, the value continued to decline due to the rainout effect of precipitation. The lower atmospheric stability at the same time suggests increasing convection, which tends to lower the stable isotopes in water vapor. The dv value is lower in stage 4 than it in stage 3, with no obvious trend, reflecting the stable influence of oceanic water vapor. The period in between precipitation (between stages 2 and 3) experienced the lowest and highest dv values with the lowest temperature and relative humidity (Fig. 2a and b), suggesting the dominant influence of the large-scale activity of cold air masses (Fig. 3a and b).
The second class (Fig. 2c and d) of the precipitation events can be divided into three stages. In stage 1, the value continued to decrease due to the rainout effect of precipitation. The dv value continued to decrease, suggesting a shift of the water vapor source from land to ocean. In stage 2, both the and dv values increased but were still lower than the initial values in stage 1, suggesting increasing contribution of local inland water vapor in the mixture of oceanic and inland water vapor. In stage 3, the showed a downward trend, and dv showed a slight decrease, reflecting the continuous influence of oceanic water vapor. Previous studies indicated that raindrop re-evaporation could contribute to changes in isotopic composition in precipitation and water vapor (Laskar et al., 2014; Conroy et al., 2016; Tian et al., 2020; Li et al., 2021). Thus, we compared the observed water vapor isotopic ratios () with the theoretical isotopic composition of the water vapor in equilibrium with that of the precipitation at local temperature () during the five precipitation events (Fig. 4). The values of the third and fourth precipitation days of event (d) are slightly larger than the observed water vapor values, indicating the re-evaporation of falling raindrops. The relative fluctuations and dv (especially lower in Fig. 2d) during stages 2 and 3 of event (d) may be caused by the effect of re-evaporation of precipitation.
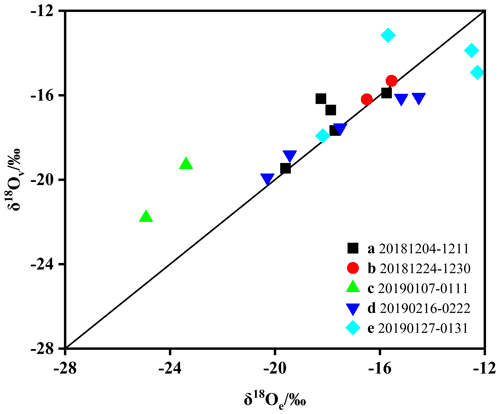
Figure 4The relationship between observed () and equilibrium () vapor isotopic ratios in the five typical precipitation events. The isotopic composition of the water vapor theoretically in equilibrium with that of the precipitation () is calculated by , where ε is the equilibrium enrichment factor, and α is the liquid-to-vapor equilibrium fractionation factor (Mercer et al., 2020). The solid black line is the line of equilibrium.
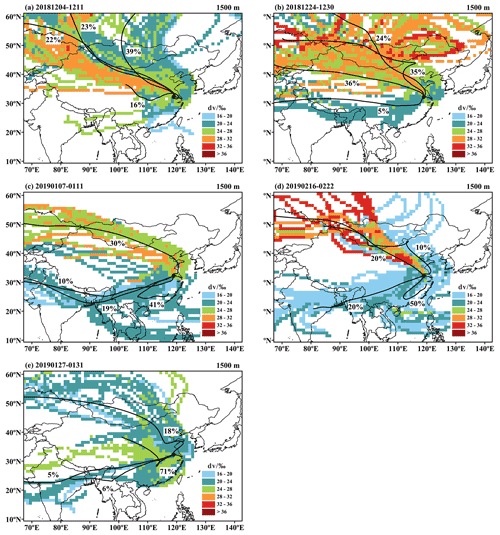
Figure 5Concentration field of water vapor dv for 192 h HYSPLIT back trajectories and clusters for the five typical precipitation events. The black circle indicates the location of Nanjing.
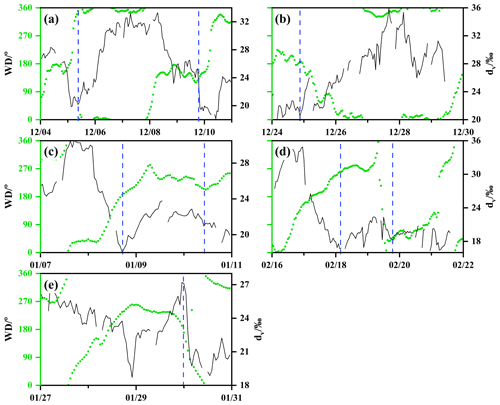
Figure 6Time series of hourly water vapor dv (black lines) and 850 hPa wind direction (green dots) of precipitation events. The vertical blue dashed lines indicate the different stages of precipitation events. (a) The event on 4–11 December 2018. (b) The event on 24–30 December 2018. (c) The event on 7–11 January 2019. (d) The event on 16–22 February 2019. (e) The event on 27–31 January 2019.
The third class (Fig. 2e) of the precipitation event can be divided into two stages. The value was relatively high with some fluctuations in stage 1, possibly due to the influence of local inland water vapor in South China where the air temperature remained high (Fig. 2e), leading to enriched isotopic values. The value started to decline in stage 2, likely caused by the rainout effect, reflecting the influence of increased convection (indicating the decrease in atmospheric stability) on the stable isotopes in water vapor. The dv value was relatively high in stage 1, likely due to the main contribution of local inland water vapor. The rapid decrease in dv in the middle may indicate the influence of oceanic water vapor. In stage 2, the dv value decreased rapidly, reflecting the rapid change in moisture sources from land water vapor to oceanic water vapor. The values of the first and second precipitation days of event (e) are significantly greater than the observed water vapor values (Fig. 4), which also indicates the re-evaporation of falling raindrops. Thus, in addition to the alternating influence of inland and oceanic water vapor, the effect of re-evaporation of precipitation could also partly account for the relatively large fluctuations of and dv during stage 1.
4.2 Moisture sources for five precipitation events
Previous studies demonstrated that seasonal variations in the stable isotopic compositions of precipitation are caused not only by local meteorological conditions (Dansgaard, 1964) but also by the different moisture sources (Bonne et al., 2020). The above analysis results show that the large-scale circulation patterns have an important influence on the event-scale stable isotopes in water vapor, and the variation in meridional wind often corresponds to the change in moisture source regions. Some studies indicated that the air masses could obtain specific isotopic signatures based on the meteorological conditions in the moisture source region before reaching a given sampling site (Salamalikis et al., 2015; Kostrova et al., 2020). Therefore, based on the HYSPLIT model, we calculated the dv value concentration fields to investigate differences among moisture source regions and water vapor transport pathways.
As seen in Fig. 5, we identified five major moisture source regions that affect the sampling site based on cluster analysis of backward trajectories during these precipitation events at South China, the East China Sea, the South China Sea, the Bay of Bengal, and continental regions (northwestern China and Mongolia). The air parcels passing areas indicated with warm colors exhibit high d-excess values in the sampling site. Trajectories passing North China, northwestern China, and Mongolia correspond to higher dv values in the sampling site (Fig. 5), as they are associated with relatively dry and cold air masses from the inland region. Moisture from other sources show lower dv values, due to higher relative humidity at the oceanic source regions (Fig. 5). These results clearly indicate that the changes in moisture source regions could play an important role in the variation in water vapor isotopic compositions in winter extreme precipitation events. We believe that abundant moisture supply through multiple moisture sources is one of the necessary conditions for the 2018–2019 winter extreme precipitation events to last for a long time.
4.3 Rapid shift of moisture sources during a precipitation event
Figure 2 shows the variation in stable isotopic values in different stages of the precipitation events divided by vertical blue dashed lines. From the above analysis, it can be seen that the intra-event variation in stable isotopic values in water vapor was mainly controlled by the change in moisture sources. Therefore, we believe that the turning points at the blue dashed lines reflected rapid shifts in moisture source regions. In order to verify this hypothesis, we plotted the relationship between the dv value and 850 hPa wind direction in the study region. Figure 6 shows that the variation in the dv value is closely related to rapid change in the wind direction, especially near the turning point. For example, during event (a) (Fig. 6a), the dv value was relatively low in the early stage, and the main wind directions are easterly and southeasterly, reflecting the influence of water vapor from the East China Sea. From the first vertical blue dashed line, the wind direction turned northerly. As a result, the dv value gradually increased and remained high, mainly due to the influence of water vapor transported by cold air mass from northwestern China and Mongolia. In the later stage, the wind direction near the second vertical blue dashed line turned to southerly and southeasterly, and the dv value decreased due to the water vapor from the East China Sea. Therefore, the high temporal resolution dv value in water vapor can be used to identify the rapid shift of moisture source regions during the continuous extreme precipitation process. Our results are in good agreement with previous studies by J. Li et al. (2015) and Han et al. (2020), who found that the moisture source and transport path can change rapidly during a precipitation event. In addition, the HYSPLIT trajectories before and after the turning points of dv are significantly different (not shown), providing further evidence that the turning points of dv correspond to the rapid shift in moisture source regions.
In this study, we presented stable isotopes in atmospheric water vapor and precipitation for five extreme winter precipitation events in Nanjing, from December 2018 to February 2019. Our analyses suggest that the variations in water vapor isotope are largely influenced by the change in moisture sources. Based on the results of the CWT model, the sampling site received different proportions of water vapor from multiple sources throughout the study period, including South China, the East China Sea, the South China Sea, the Bay of Bengal, and continental regions (northwestern China and Mongolia). In particular, the turning points of the water vapor d excess during a precipitation event reflected the rapid shift in moisture source regions. Our results imply that multiple moisture sources and the rapid shift among them are important conditions for sustaining extreme precipitation events, especially in the relatively cold and dry winter.
The data set of daily water vapor ( and ), air temperature, water vapor concentration, relative humidity, precipitation amount, and precipitation ( and ) at Nanjing is available at https://data.tpdc.ac.cn/en/data/d117f51c-b47f-4bfd-9030-0c54f15067cf/ (Pang, 2021).
HP and SH conceived this study. Material preparation, data collection, and analysis were performed by TX, ZZ, and WZ. The first draft of the paper was written by TX. All authors contributed to the discussion of the results.
The contact author has declared that neither they nor their co-authors have any competing interests.
Publisher's note: Copernicus Publications remains neutral with regard to jurisdictional claims in published maps and institutional affiliations.
This work has been supported by the National Natural Science Foundation of China (grant nos. 41771031, 91837102, and 41830644) and the Priority Academic Program Development of Jiangsu Higher Education Institutions (PAPD). We would also like to thank the NOAA Air Resource Laboratory (ARL) for providing the HYSPLIT model used in this paper.
This research has been supported by the National Natural Science Foundation of China (grant nos. 41771031, 91837102, and 41830644).
This paper was edited by Bob Su and reviewed by Li Maoshan and two anonymous referees.
Araguás-Araguás, L., Froehlich, K., and Rozanski, K.: Deuterium and oxygen-18 isotope composition of precipitation and atmospheric moisture, Hydrol. Process., 14, 1341–1355, https://doi.org/10.1002/1099-1085(20000615)14:8<1341::AID-HYP983>3.0.CO;2-Z, 2000.
Baker, A., Sodemann, H., Baldini, J., Breitenbach, S., Johnson, K., Hunen, J. V., and Zhang, P. Z.: Seasonality of westerly moisture transport in the East Asian summer monsoon and its implications for interpreting precipitation δ18O, J. Geophys. Res.-Atmos., 120, 5850–5862, https://doi.org/10.1002/2014JD022919, 2015.
Bedaso, Z. and Wu, S. Y.: Daily precipitation isotope variation in Midwestern United States: Implication for hydroclimate and moisture source, Sci. Total. Environ., 713, 136631, https://doi.org/10.1016/j.scitotenv.2020.136631, 2020.
Benetti, M., Reverdin, G., Pierre, C., Merlivat, L., Risi, C., Steen-Larsen, H. C., and Vimeux, F.: Deuterium excess in marine water vapor: Dependency on relative humidity and surface wind speed during evaporation, J. Geophys. Res.-Atmos., 119, 584–593, https://doi.org/10.1002/2013JD020535, 2014.
Bonne, J.-L., Masson-Delmotte, V., Cattani, O., Delmotte, M., Risi, C., Sodemann, H., and Steen-Larsen, H. C.: The isotopic composition of water vapour and precipitation in Ivittuut, southern Greenland, Atmos. Chem. Phys., 14, 4419–4439, https://doi.org/10.5194/acp-14-4419-2014, 2014.
Bonne, J.-L., Meyer, H., Behrens, M., Boike, J., Kipfstuhl, S., Rabe, B., Schmidt, T., Schönicke, L., Steen-Larsen, H. C., and Werner, M.: Moisture origin as a driver of temporal variabilities of the water vapour isotopic composition in the Lena River Delta, Siberia, Atmos. Chem. Phys., 20, 10493–10511, https://doi.org/10.5194/acp-20-10493-2020, 2020.
Cai, Z. and Tian, L.: Processes governing water vapor isotope composition in the Indo-Pacific region: Convection and water vapor transport, J. Climate, 29, 8535–8546, https://doi.org/10.1175/JCLI-D-16-0297.1, 2016.
Conroy, J. L., Noone, D., Cobb, K. M., Moerman, J. W., and Konecky, B. L.: Paired stable isotopologues in precipitation and vapor: A case study of the amount effect within western tropical Pacific storms, J. Geophys. Res.-Atmos., 121, 3290–3303, https://doi.org/10.1002/2015jd023844, 2016.
Dansgaard, W.: Stable isotopes in precipitation, Tellus, 16, 436–468, https://doi.org/10.3402/tellusa.v16i4.8993, 1964.
Ding, F. and Li, C.: Subtropical westerly jet waveguide and winter persistent heavy rainfall in south China, J. Geophys. Res.-Atmos., 122, 7385–7400, https://doi.org/10.1002/2017JD026530, 2017.
Ding, Y., Wang, Z., Song, Y., and Zhang, J.: Causes of the unprecedented freezing disaster in January 2008 and its possible association with the global warming, Acta Meteorol. Sin., 66, 808–825, 2008 (in Chinese with English abstract).
Gao, J., He, Y., Masson-Delmotte, V., and Yao, T.: ENSO effects on annual variations of summer precipitation stable isotopes in Lhasa, southern Tibetan Plateau, J. Climate, 31, 1173–1182, https://doi.org/10.1175/JCLI-D-16-0868.1, 2018.
Galewsky, J., Steen-Larsen, H. C., Field, R. D., Worden, J., Risi, C., and Schneider, M.: Stable isotopes in atmospheric water vapor and applications to the hydrologic cycle, Rev. Geophys., 54, 809–865, https://doi.org/10.1002/2015RG000512, 2016.
Gu, X., Pang, H., Li, Y., Zhang, W., and Wang, J.: Study on calibration method for atmospheric water vapor stable isotopes observed by cavity ring-down spectroscopy, Spectrosc. Spect. Anal., 39, 1700–1705, 2019.
Guo, L., Liu, B., and Zhu, C.: Extraordinary long wet spell in south of Yangtze River during 2018/2019 winter and its possible causes, Chinese Sci. Bull., 64, 3498–3509, 2019 (in Chinese with English abstract).
Han, T., Zhang, M., Wang, S., Qu, D., and Du, Q.: Sub-hourly variability of stable isotopes in precipitation in the marginal zone of East Asian monsoon, Water, 12, 2145, https://doi.org/10.3390/w12082145, 2020.
He, S. and Richards, K.: Stable isotopes in monsoon precipitation and water vapour in Nagqu, Tibet, and their implications for monsoon moisture, J. Hydrol., 540, 615–622, https://doi.org/10.1016/j.jhydrol.2016.06.046, 2016.
Huang, W., He, X., Yang, Z., Qiu, T., Wright, J. S., Wang, B., and Lin, D.: Moisture sources for wintertime extreme precipitation events over South China during 1979–2013, J. Geophys. Res.-Atmos., 123, 6690–6712, https://doi.org/10.1029/2018JD028485, 2018a.
Huang, W., Yang, Z., He, X., Lin, D., Wang, B., Wright, J. S., Chen, R., Ma, W., and Li, F.: A possible mechanism for the occurrence of wintertime extreme precipitation events over South China, Clim. Dynam., 52, 2367–2384, https://doi.org/10.1007/s00382-018-4262-8, 2018b.
Kostrova, S. S., Meyer, H., Fernandoy, F., Werner, M., and Tarasov, P. E.: Moisture origin and stable isotope characteristics of precipitation in southeast Siberia, Hydrol. Process., 34, 51–67, https://doi.org/10.1002/hyp.13571, 2020.
Laskar, A., Huang, J., Hsu, S., Bhattacharya, S., Wang, C., and Liang, M.: Stable isotopic composition of near surface atmospheric water vapor and rain–vapor interaction in Taipei, Taiwan. J. Hydrol., 519, 2091–2100, https://doi.org/10.1016/j.jhydrol.2014.10.017, 2014.
Li, C., Yang, H., and Zhao, J.: Combinational anomalies of atmospheric circulation system and occurrences of extreme weather/climate events, Trans. Atmos. Sci., 42, 321–333, https://doi.org/10.13878/j.cnki.dqkxxb.20190302001, 2019 (in Chinese with English abstract).
Li, J., Tao, T., Pang, Z., Tan, M., Kong, Y., Duan, W., and Zhang, Y.: Identification of different moisture sources through isotopic monitoring during a storm event, J. Hydrometeorol., 16, 1918–1927, https://doi.org/10.1175/JHM-D-15-0005.1, 2015.
Li, X., Li, J., and Li, Y.: Recent winter precipitation increase in the Middle-Lower Yangtze River Valley since the Late 1970s: A response to warming in the Tropical Indian Ocean, J. Climate, 28, 3857–3879, https://doi.org/10.1175/JCLI-D-14-00701.1, 2015.
Li, X., Wang, C., Ling, T., Sun, C., Zhang, Y., and Wang, J.: Features and possible causes of the extreme precipitation anomaly in China during winter 2019/2020, Front. Earth. Sci., 8, 596753, https://doi.org/10.3389/feart.2020.596753, 2020a.
Li, X., Wen, Z., and Huang, W.: Modulation of South Asian Jet wave train on the extreme winter precipitation over Southeast China: Comparison between 2015/16 and 2018/19, J. Climate, 33, 4065–4081, https://doi.org/10.1175/JCLI-D-19-0678.1, 2020b.
Li, X., Tang, C., and Cui, J.: Intra-event isotopic changes in water vapor and precipitation in South China, Water, 13, 940, https://doi.org/10.3390/w13070940, 2021.
Li, Y., An, W., Pang, H., Wu, S. Y., Tang, Y., Zhang, W., and Hou, S.: Variations of stable isotopic composition in atmospheric water vapor and their controlling factors – A 6-Year continuous sampling study in Nanjing, Eastern China, J. Geophys. Res.-Atmos., 125, e2019JD031697, https://doi.org/10.1029/2019JD031697, 2020.
Mercer, J. J., Liefert, D. T., and Williams, D. G.: Atmospheric vapour and precipitation are not in isotopic equilibrium in a continental mountain environment, Hydrol. Process., 34, 3078–3101, https://doi.org/10.1002/hyp.13775, 2020.
Merlivat, L. and Jouzel, J.: Global climatic interpretation of the deuterium-oxygen 18 relationship for precipitation, J. Geophys. Res., 84, 5029–5033, https://doi.org/10.1029/JC084iC08p05029, 1979.
Pang, H.: Daily water vapor and precipitation isotopes in Nanjing of eastern China, National Tibetan Plateau Data Center [data set], https://data.tpdc.ac.cn/en/data/d117f51c-b47f-4bfd-9030-0c54f15067cf/ last access: 25 January 2021.
Peng, T. R., Wang, C. H., Huang, C. C., Fei, L. Y., Chen, C. T. A., and Hwong, J. L.: Stable isotopic characteristic of Taiwan's precipitation: A case study of western pacific monsoon region, Earth Planet. Sc. Lett., 289, 357–366, https://doi.org/10.1016/j.epsl.2009.11.024, 2010.
Qin, P., Xie, Z., Zou, J., Liu, S., and Chen, S.: Future precipitation extremes in China under climate change and their physical quantification based on a regional climate model and CMIP5 model simulations, Adv. Atmos. Sci., 38, 460–479, https://doi.org/10.1007/s00376-020-0141-4, 2021.
Rahmstorf, S. and Coumou, D.: Increase of extreme events in a warming world, P. Natl. Acad. Sci. USA, 108, 17905–17909, https://doi.org/10.1073/pnas.1101766108, 2011.
Salamalikis, V., Argiriou, A. A., and Dotsika, E.: Stable isotopic composition of atmospheric water vapor in Patras, Greece: A concentration weighted trajectory approach, Atmos. Res., 152, 93–104, https://doi.org/10.1016/j.atmosres.2014.02.021, 2015.
Sun, B. and Wang, H.: Analysis of the major atmospheric moisture sources affecting three sub-regions of East China, Int. J. Climatol., 35, 2243–2257, https://doi.org/10.1002/joc.4145, 2015.
Tang, Y., Pang, H., Zhang, W., Li, Y., Wu, S., and Hou, S.: Effects of changes in moisture source and the upstream rainout on stable isotopes in precipitation – a case study in Nanjing, eastern China, Hydrol. Earth Syst. Sci., 19, 4293–4306, https://doi.org/10.5194/hess-19-4293-2015, 2015.
Tian, L., Yao, T., Macclune, K., White, J., Schilla, A., Vaughn, B., Vachon, R., and Ichiyanagi, K.: Stable isotopic variations in west China: A consideration of moisture sources, J. Geophys. Res.-Atmos., 112, D10112, https://doi.org/10.1029/2006JD007718, 2007.
Tian, L., Yu, W., Schuster, P. F., Wen, R., Cai, Z., Wang, D., Shao, L., Cui J., and Guo, X.: Control of seasonal water vapor isotope variations at Lhasa, southern Tibetan Plateau, J. Hydrol., 580, 1–11, https://doi.org/10.1016/j.jhydrol.2019.124237, 2020.
Tremoy, G., Vimeux, F., Soumana, S., Souley, I., Risi, C., Favreau, G., and Oï, M.: Clustering mesoscale convective systems with laser-based water vapor δ18O monitoring in Niamey (Niger), J. Geophys. Res.-Atmos., 119, 5079–5103, https://doi.org/10.1002/2013JD020968, 2014.
Uemura, R., Matsui, Y., Yoshimura, K., Motoyama, H., and Yoshida, N.: Evidence of deuterium excess in water vapor as an indicator of ocean surface conditions, J. Geophys. Res.-Atmos., 113, D19114, https://doi.org/10.1029/2008JD010209, 2008.
van der Ent, R. J. and Tuinenburg, O. A.: The residence time of water in the atmosphere revisited, Hydrol. Earth Syst. Sci., 21, 779–790, https://doi.org/10.5194/hess-21-779-2017, 2017.
Wang, B., Wu, R. G., and Fu, X. H.: Pacific-East Asian teleconnection: How does ENSO affect East Asian climate? J. Climate, 13, 1517–1536, https://doi.org/10.1175/1520-0442(2000)013<1517:PEATHD>2.0.CO;2, 2000.
Wang, L. and Feng, J.: Two major modes of the wintertime precipitation over China, Chin. J. Atmos. Sci., 35, 1105–1116, https://doi.org/10.3878/j.issn.1006-9895.2011.06.10, 2011 (in Chinese with English abstract).
Wang, S., Zhang, M., Crawford, J., Hughes, C. E., Du, M., and Liu, X.: The effect of moisture source and synoptic conditions on precipitation isotopes in arid central Asia, J. Geophys. Res.-Atmos., 122, 2667–2682, https://doi.org/10.1002/2015JD024626, 2017.
Wang, Z., Sun, J., Wu, J., Ning, F., and Chen, W.: Attribution of persistent precipitation in the Yangtze-Huaihe river basin during February 2019, Adv. Atmos. Sci., 37, 1389–1404, https://doi.org/10.1007/s00376-020-0107-6, 2020.
Yang, Z., Huang, W., He, X., Wang, Y., Qiu, T., Wright, J. S., and Wang, B.: Synoptic conditions and moisture sources for extreme snowfall events over East China, J. Geophys. Res.-Atmos., 124, 601–623, https://doi.org/10.1029/2018JD029280, 2019.
Yao, Y., Lin, H., and Wu, Q.: Subseasonal variability of precipitation in China during boreal winter, J. Climate, 28, 6548–6559, https://doi.org/10.1175/JCLI-D-15-0033.1, 2015.
Yu, W., Tian, L., Ma, Y., Xu, B., and Qu, D.: Simultaneous monitoring of stable oxygen isotope composition in water vapour and precipitation over the central Tibetan Plateau, Atmos. Chem. Phys., 15, 10251–10262, https://doi.org/10.5194/acp-15-10251-2015, 2015.
Zhao, N., Manda, A., Guo, X., Kikuchi, K., Nasuno, T., Nakano, M., Zhang, Y., and Wang, B.: A Lagrangian view of moisture transport related to the heavy rainfall of July 2020 in Japan: Importance of the moistening over the subtropical regions, Geophys. Res. Lett., 48, e2020GL091441, https://doi.org/10.1029/2020GL091441, 2021.
Zhou, B., Gu, L., Ding, Y., Shao, L., Wu, Z., Yang, X., Li, C., Li, Z., Wang, X., Cao, Y., Zeng, B., Yu, M., Wang, M., Wang, S., Sun, H., Duan, A., An, Y., Wang, X., and Kong, W.: The great 2008 Chinese ice storm: Its socioeconomic-ecological impact and sustainability lessons learned, B. Am. Meteorol. Soc., 92, 47–60, https://doi.org/10.1175/2010BAMS2857.1, 2011.
Zhou, J. and Li, T.: A tentative study of the relationship between annual δ18O & δD variations of precipitation and atmospheric circulations – A case from Southwest China, Quatern. Int., 479, 117–127, https://doi.org/10.1016/j.quaint.2017.05.038, 2017.
Zhou, L., Tam, C. Y., Zhou, W., and Chan, J. C. L.: Influence of South China Sea SST and the ENSO on winter rainfall over South China, Adv. Atmos. Sci., 27, 832–844, https://doi.org/10.1007/s00376-009-9102-7, 2010.
Zong, H., Bueh, C., and Ji, L.: Wintertime extreme precipitation event over southern China and its typical circulation features, Chinese Sci. Bull., 59, 1036–1044, https://doi.org/10.1007/s11434-014-0124-x, 2014.