the Creative Commons Attribution 4.0 License.
the Creative Commons Attribution 4.0 License.
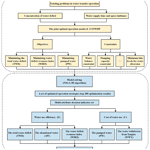
Joint optimal operation of the South-to-North Water Diversion Project considering the evenness of water deficit
Bing-Yi Zhou
Guo-Hua Fang
Xin Li
Jian Zhou
Hua-Yu Zhong
Inter-basin water transfer projects are the main measure to address the water deficit crisis caused by uneven distribution of water resources. The current water transfer operation mainly tends to be present in areas with small water transfer costs and is prone to encounter the problem of spatial and temporal imbalances in water allocation. To address these issues, this paper defines a water deficit evenness index (WDEI), aimed at minimizing regional differences in water scarcity and sharing the pressure of water scarcity as a social demand objective. This index is incorporated into a joint optimization model for the Jiangsu section of the South-to-North Water Diversion Project (J-SNWDP), which comprises both the ecological objective of the total water deficit (TWD) and the economic objective of the pumping water (PW). Further, the Nondominated Sorting Genetic Algorithm III (NSGA-III) and multi-attribute decision-making were applied to solve the model and obtain an optimal operation strategy. The results showed the following: (1) the WDEI defined in this paper can mitigate the synchronized water scarcity in certain water users. In typical normal years (wet year and dry year), the WDEI shows a reduction of 94.2 % (81.8 %, 76.7 %) compared to the historical operation strategy. (2) The optimized operation strategy can significantly reduce TWD and PW by 82.06% (37.69 %, 52.36 %) and 45.13 % (3.25 %, 21.51 %) compared with the historical values, respectively, which can improve the water supply satisfaction and reduce the project cost. At the same time, the lake storage capacity of the optimal operation strategy performs well, and the water transfer efficiency of the river is significantly improved. (3) In this paper, targeted optimal operation strategies and potential ways to secure the project tasks are proposed for different natural flow. Overall, it is of great significance to study the water supply equity in the J-SNWDP to alleviate the concentrated water deficit in Jiangsu Province and other similar regions.
- Article
(7755 KB) - Full-text XML
- BibTeX
- EndNote
Influenced by the impacts of global climate change, human activities, and increasing water demand, issues like regional water resource deficits, flood and drought disasters, and the conflicts between water supply and demand are progressively intensifying (Florke et al., 2018; Ma et al., 2020; Kato and Endo, 2017; Rossi and Peres, 2023). These social issues have become one of the key factors constraining regional and even global sustainable development and environmental protection (Li et al., 2020; Liu et al., 2021; Tian, 2017). Inter-basin water transfer (IBWT) projects have been widely constructed worldwide as an effective way to address water deficit issues caused by uneven distribution of water resources and improve their utilization efficiency (Sun et al., 2021; Wei et al., 2022; Medeiros and Sivapalan, 2020). At least 10 % of the cities worldwide receive water from IBWT projects (McDonald et al., 2014). The birth of the Lancang–Mekong Cooperation promotes the joint development of six countries, namely China, Cambodia, Laos, Myanmar, Thailand, and Vietnam (Ghoreishi et al., 2023). The California State Water Project, the Colorado River Aqueduct (Lopez, 2018), the Senqu–Vaal transfer in South Africa and Lesotho (Gupta and van der Zaag, 2008), the Snowy Mountains Scheme in southeastern Australia (Pigram, 2000), and other inter-basin water transfer projects have all effectively alleviated water scarcity issues in various regions (Lu et al., 2021). The South-to North Water Diversion Project (SNWDP) in China (Guo and Li, 2013) is considered the largest inter-basin water transfer project in the world. The project runs along numerous water users, and the water resources it provides have already benefited hundreds of millions of people, with even more expected to be served in the future (Pohlner, 2016).
There are considerable studies on the water resources operating strategy of the supply-oriented IBWT projects in terms of social, economic, ecological, and environmental factors (Gan et al., 2011; Zhu et al., 2014; Liu and Zheng, 2002; Xu et al., 2013). In general, meeting the water demand of various users is the main task of the IBWT project, with the consideration of minimizing water deficit in previous studies (Guo et al., 2020; Wang et al., 2008). Rather than the total amount of water deficit, the crux of the problem may actually be the concentration of water deficit in a certain period of time or region, which has not yet received sufficient attention and remains a major challenge. Therefore, both the total and spatial–temporal distribution of water deficit should be considered in the optimization process (Xu et al., 2013). In addition, users' demands and decision makers' benefits should be considered priorities (Zhang et al., 2012), so minimizing pumped water (PW) is a direct way to reduce costs. At the same time, the proportion of the amount of abandoned water and the water withdrawn from the river in the process of water diversion should be taken as secondary considerations (Guo et al., 2018). However, due to the data on natural water and user water demand as the determining factors of the operation strategy, and the obvious regional differences, most of the objectives determined by the existing studies can only solve small-scale projects.
As the project continues to operate, the focus of research should be concentrated on the planning of operational strategies to sustain the long-term operation of the project and enhance its comprehensive benefits. For IBWT projects, due to regional differences, improving operational efficiency and benefits while ensuring water supply is a challenging task. Currently, most IBWT projects primarily adhere to various laws and policies established by the government. These projects comply with annual water demand plans submitted by sectors like agriculture, domestic use, and ecology. The water supply principle is based on “prioritizing users that are closer in distance, have lower water supply costs, and have larger water demands” to develop operation strategies. Such a method of water diversion results in lower satisfaction levels for users that are farther in distance and have higher costs, leading to an imbalance in water supply and causing some users to face significant pressure from concentrated water deficits. Furthermore, these projects lack annual predictive assessments of local hydrological conditions and fail to develop targeted operational strategies for diverse natural inflows or extreme events. Developing operational strategies without considering the evenness of water deficit and natural inflows is unscientific. This inspires the primary objective of optimization in this paper.
The South-to-North Water Diversion Project (SNWDP) presents a highly complex and dynamic water situation, especially in the Jiangsu section (Vogel et al., 2015). Due to differences in the location and timing of natural inflows and water users, and the aforementioned issues in operational strategies, an imbalance in water supply has arisen. There have been some studies attempting to address this issue, but they tend to focus on meeting the total water demand and improving the overall benefits (Li et al., 2017; Zhuan et al., 2016), neglecting the fairness of water supply among different regions. Water supply may become concentrated on a specific user or time period. Therefore, it is of great theoretical significance and practical application value to optimize the existing operation strategy to alleviate the concentration of water deficit so as to realize the comprehensive benefits of the IBWT project (Nazemi and Wheater, 2015; Peng et al., 2015).
To address the above problem, this paper studies the Jiangsu section of South-to-North Water Diversion Project (J-SNWDP). The three main contributions of this paper are as follows: (1) the definition of the water deficit evenness index (WDEI) and its incorporation into the joint optimal operation model of the J-SNWDP, along with the total water deficit (TWD) and the pumped water (PW), aim to satisfy the requirements of both decision-makers and users. (2) The incorporation of the amount of abandoned water and the water withdrawn from the Yangtze River into the decision indicator set, along with the application of the multi-attribute decision making method for filtering the Pareto front strategies of the Nondominated Sorting Genetic Algorithm III (NSGA-III), results in the identification of the optimal operation strategy that balances economic and ecological benefits. (3) The comparison of the optimal operation strategy selected in 3 typical years (wet, normal, and dry) with the historical operation strategy under identical natural conditions in the paper serves to verify the superiority of the optimization results, offering reasonable optimization suggestions for the J-SNWDP and other similar regions.
2.1 Study area and data
2.1.1 Regional overview
The Jiangsu section of the South-to-North Water Diversion Project (J-SNWDP) crosses the Yangtze River and Huai River basins. A simplified map of the J-SNWDP is shown in Fig. 1. The Beijing–Hangzhou Grand Canal runs through the north and south of Jiangsu Province, connecting the Yangtze River basin, the Huai River basin, and the Yishusi River basin. The total length of the Jiangsu section is 404 km, along which there are six cities, namely Yangzhou, Huaian, Yancheng, Suqian, Lianyungang, and Xuzhou. The J-SNWDP consists of three impounded lakes (Hongze Lake, Luoma Lake, Nansi Lake), six sluices (e.g. Erhe sluice, Gaoliangjian sluice), and 13 pumping stations (Huaian station, Jiangdu station), forming a double-route water diversion system including the West Route and the Canal Route. The water supply scope of the whole J-SNWDP covers the three provinces of Jiangsu, Anhui, and Shandong, supplementing the agricultural, industrial, and domestic water supply as well as navigation and ecological water supply in the areas along the water transfer route. The scale parameters of the pumping stations and sluices in J-SNWDP are listed in Table 1. The characteristics of the lakes in the J-SNWDP are shown in Table 2.
Table 1Pumping stations and sluices of the Jiangsu section of the South-to-North Water Diversion Project.
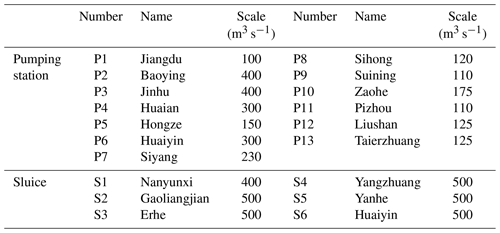
When the J-SNWDP is supplying water along the water transfer route, users closer to the water source (such as Jinbaohangdao (JBHD) user, Sihong–Suining (S-S) user, Luoma (LM) user) are generally prioritized. On the other hand, users like the Feihuanghe user, Lianyungang user, and Siyang–Zaohe user, who are located farther from the water source and receive less water from Luoma Lake, often require water to be pumped from the Yangtze River and Hongze Lake for replenishment. Combined with the uneven spatial and temporal distribution of precipitation, these recipient zones are more susceptible to water deficits.
In Fig. 2, the complex relationship of the J-SNWDP is generalized into a schematic diagram according to the geographical location. In order to represent the main components of the system and the connection between the backbone rivers, we regard the lakes, pumping stations, and sluices as the nodes and the backbone river as the connecting line, while the water users are reasonably distributed among the nodes of the water transmission route.
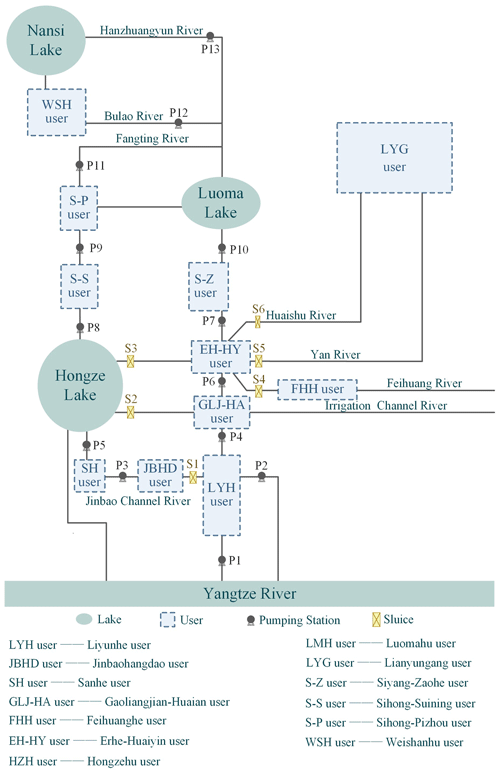
Figure 2Schematic diagram of the Jiangsu section of the South-to-North Water Diversion Project (J-SNWDP).
The joint operation process of the J-SNWDP is described as follows. In addition to natural precipitation and lake inflow, the Yangtze River is the main water source of water, which is pumped from the river to the West Route and the Canal Route by the P1 pumping station. The West Route is pumped step by step from P2, P3, and P4 to Hongze Lake through Sanyang River and Jinbao Channel and pumped from P9, P10, and P11 to the north through Xuhong and Fangting rivers. The Canal Route along the Beijing–Hangzhou Grand Canal route is pumped north from P5, P6, P7, and P8. The two water transmission routes supplement the water demand of users along the routes while pumping to the north and merge into one line at the intersection of Fangting River and Zhongyun River. It is then pumped further north until P12 and P13 are transported to Nansi Lake via Bulao and Hanzhuangyun rivers, respectively, thus delivering water outside Jiangsu Province. When the natural inflow is high, Hongze Lake can be discharged via P1 on the West Route or through S2, S3, S4, S5, and S6 to the relevant rivers and users. Luoma and Nansi lakes are discharged through the original water transmission routes.
2.1.2 Data
The joint optimal operating model of the J-SNWDP uses a monthly time step. According to the annual natural inflow data of Hongze (HZ), Luoma (LM), and Nansi (NS) lakes since 2013 from Jiangsu Water Resources Bulletin, 3 hydrological years were selected to represent wet (October 2017–September 2018, annual mean inflow: 465.12×108 m3), normal (October 2019–September 2020, annual mean inflow: 172.80×108 m3), and dry (October 2013–September 2014, annual mean inflow: 103.06×108 m3) years, respectively. The typical annual runoff curves of HZ, LM, and NS lakes are shown in Fig. 3. The water supply area of the J-SNWDP is divided into 13 receiving areas according to geographical location, and water demand data are provided by the Jiangsu Provincial Water Resources Department: wet years (October 2017–September 2018, water demand: 171.29×108 m3), normal years (October 2019–September 2020, water demand: 173.51×108 m3), dry years (October 2013–September 2014, water demand: 181.75×108 m3).
2.2 Water deficit evenness index
For IBWT projects, issues of equitable water supply often arise, especially for projects like the J-SNWDP, which has 13 water users along its route. Different users have significant spatial variations in location, as well as large differences in water supply costs. Consequently, the concentration of water deficits is particularly severe when there are multiple users, which results in some of them being stressed by water deficits.
Luoma Lake, which almost receives no inflow during dry periods yet bears the primary responsibility for out-of-province water supply task, requires multilevel pumping stations to pump water upward (water needs to be pumped from Luoma Lake through two pumping stations, from Hongze Lake through six pumping stations, and from the Yangtze River through nine pumping stations), creating a substantial burden on water supply. In past water supply dispatching of the J-SNWDP, the pursuit of maximum benefit while neglecting this issue would obviously cause certain users to bear severe water deficit risks, resulting in significant damage to the water supply system.
Therefore, considering the fairness of water supply is very important for the practical operation of the project. In this paper, the WDEI (water deficit evenness index) is defined as an indicator representing the degree of water deficit concentration, and the variance can reflect the difference in water deficits among various recipient zones. By incorporating the WDEI into the optimization objective and minimizing it, the difference in water deficit can be reduced as much as possible. Therefore, the WDEI is as follows:
where QR(i,t) is the water deficit of the ith user at time step t; i=1, 2, …, n, with n being the total number of users (n=13 in this study); and t=1, 2, …, T, with T being the whole operating period.
2.3 The joint optimal operation model of J-SNWDP
Herein, the joint optimal operating model of the J-SNWDP was constructed with 228 decision variables (13 pumping stations and six sluices). The objective function and associated constraints are formulated as follows.
2.3.1 Objectives
(1) Minimizing the total water deficit (TWD)
TWD is a measure of how well the operation strategy completion is being implemented. This objective aims to minimize the total amount of water deficit at the end of a given operation period, potentially improving the satisfaction of water demand for users and increasing the operation strategy completion.
where QR(i,t) is the water deficit of the ith user at time step t, i=1, 2, …, n, with n being the total number of users (n=13 in this study), and t=1, 2, …, T, with T being the whole operating period.
(2) Minimizing water deficit evenness index (WDEI)
WDEI indicates the degree of concentration of the water deficit and can be used as an indicator of the uniformity of water diversion. The lower the WDEI, the better the strategy is.
where QR(i,t) is the water deficit of the ith user at time step t; i=1, 2, …, n, with n being the total number of users (n=13 in this study); and t=1, 2, …, T, with T being the whole operating period.
(3) Minimizing pumped water (PW)
PW reflects the economy of operation strategy. The lower the PW is, the lower the operating costs.
where QS(p,t) is the water pumped by the pth pumping station at time step t, and p=1, 2, …, P, with P being the total number of pumping stations (P=13 in this study).
2.3.2 Constraints
Systems operation should obey operating rules and physical constraints, such as water balance, pumping capacity, and lake storage constraints. The mathematical expressions of the constraints are shown below.
(1) Water balance constraint
The water balance constraint should be satisfied in the water diversion process.
at time step t, where V(i,t) is the water storage of the ith lake at time step t, Q(i,t) is the inflow of the ith lake, W1(i,t) is the water demand of the ith lake (water to be supplemented by J-SNWDP after deducting the locally available water), DO(i,t) is water diversion to the north from the ith lake, DI(i,t) is the water pumped into the ith lake, PC(i,t) is the water discharged into the ith lake, and PR(i,t) is the water discharged from the ith lake.
(2) Pumping capacity constraint
at time step t, where DO(i,t) is water diversion to the north from the ith lake, DI(i,t) is the water pumped into the ith lake, DOmax(i,t) is the maximum pumping capacity that is pumped into the ith lake, and DImax(i,t) is the maximum pumping capacity that is diverted north from the ith lake.
(3) Sluice capacity constraint
where PR(i,t) is the water discharged from the ith lake, and PRmax(i,t) is the maximum sluice capacity at time step t.
(4) Lake storage constraint
where V(i,t) is the water storage of the ith lake at time step t, and Vmin(i,t) and Vmax(i,t) are the minimum and maximum water storage capacities at time step t, respectively. When is the water deficit, , ensure that the lake level is above the limit level, and when is abandoned water, ensure that the water storage of the lakes is within a reasonable range.
(5) Minimum lake levels for water diversion
where Z(i,t) is the level of the ith lake at time step t, and Zmin(i,t) and Zmax(i,t) are the minimum and maximum level of the ith lake at time step t, respectively.
2.4 Model solving and solution selection
Figure 4 shows the optimization method and process of the Jiangsu section of the South-to-North Water Diversion Project. Considering the complex objectives and various physical constraints, the NSGA-III algorithm (Deb and Jain, 2014) is used in this paper to solve the model. NSGA-III has been widely used to solve various water-resource optimal diversion problems and to obtain optimal operation strategies with the advantages of fast execution speed and high efficiency (Ni et al., 2019; Tang et al., 2021; Zhou et al., 2020). The NSGA-III is used to solve the joint optimal operation model of the J-SNWDP under three typical conditions: wet years, normal years, and dry years. The model takes the actual value of the pumped water of each pumping station as the decision variable. The population size, generation, crossover rate, and mutation rate are set to 200, 20000, 0.9, and 0.1, respectively. The application of NSGA-III to the model can be summarized in the following steps: (1) take 12 monthly water pumped of a year as the decision variables and initialize the population of size N based on the physical constraints of operation. (2) Calculate the objectives of TWD, WDEI, and PW for each chromosome and sort by the non-dominated strategy. (3) Select excellent chromosomes from the non-dominated population as parent chromosomes and create child chromosome by the crossover and mutation operation. (4) Combine the parent chromosome with the child chromosome and update the population by the non-dominated strategy. (5) Repeat the four steps above until the number of iterations is reached.
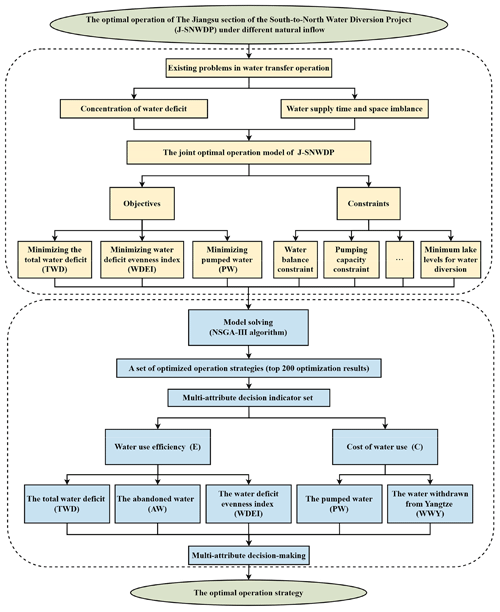
Figure 4Optimization process of the Jiangsu section of the South-to-North Water Diversion Project (J-SNWDP).
After solving the model using NSGA-III, we obtained a set of optimized running strategies (i.e. strategies based on the Pareto-ranked top 200). In order to measure the operation effect more comprehensively, more indicators are needed to assist in selecting an optimal operation strategy. Therefore, this paper further applies multi-attribute decision-making methods to screen and determine the optimal operation strategy. The abandoned water reflects the regulation and storage capacity of the lakes, and the water withdrawn from the Yangtze River reflects the impact of the water transferred outside the system on the operation strategy. A multi-attribute decision indicator set is constructed, which included five optimization objectives for the total water deficit, the water deficit evenness index, the pumping water, the abandoned water, and the water withdrawn from the Yangtze River. Indicators are divided into water use efficiency and cost of water use indicators as shown in Fig. 4. In this paper, we adopt the analytic hierarchy process (AHP) method to determine the subjective weights and the entropy weighting method to determine the objective weights. Further, the combination weights are obtained by a linear weighted average.
3.1 Pareto front strategies of NSGA-III under different years
The historical operation strategy of the J-SNWDP follows the principle of “replenishing the lake during water deficit, and discharging during water surplus”, with the primary goal of ensuring the completion of water supply tasks. Firstly, the simulation of a single lake is carried out, and then the simulation of each storage lake is carried out according to the top-down order, so as to complete the conventional operation of the engineering system. Such a method is simply “robbing Peter to pay Paul”. The external water transfer is not fully deployed but increases the operating costs. In contrast, the joint optimal operation exhibits several advantages, such as controlling the minimum WDEI of each user, ensuring that even if the total water deficit and the historical operating strategy are the same, the water deficit pressure is evenly distributed to avoid undesirable water deficit concentration. In addition, the optimal operation process pays more attention to the water storage function of the lake. In the non-flood season, the water level of the reservoir is kept at the normal water storage level as much as possible, and the water storage (discharge) is appropriate according to different natural inflow, so that the whole system can realize the lake storage in the flood season and non-flood season at the same time and improve the utilization rate of water resources while fulfilling the water supply task.
Figure 5 provides visualizations of the optimal operation strategy based on Pareto sets in different years. Obviously, the compromised operation strategies are well distributed in 3 typical years, and the compromised operation strategies and the optimal operation strategy are located in the middle of the curve. The relative relationship between the optimization objectives has been analysed, and the total water deficit has a positive correlation with the water deficit evenness index. In contrast, the total water deficit and water deficit evenness index have a negative correlation with the pumped water, indicating that the water deficit gradually decreases with the increase of PW. The distribution pattern of the marker is formed under the interaction of three objectives (see Fig. 5).
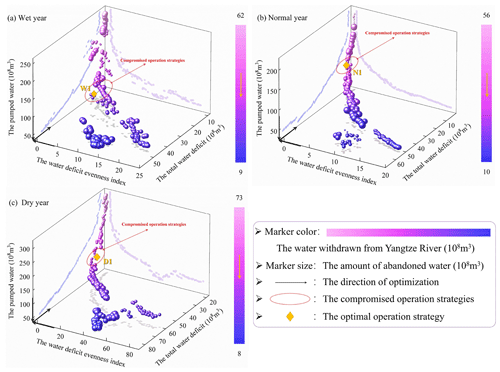
Figure 5Illustration of Pareto front strategies for (a) wet, (b) normal, and (c) dry years. (W1, N1, and D1 represent the location of the optimal operation strategy in different typical years.)
Figure 5a shows the competitive relationships between TWD, WDEI, and PW in wet years; PW decreases sharply and WDEI increases slowly at the beginning when TWD increases by 20×108 m3, WDEI increases by about 3×108 m3, and PW decreases by about 164×108 m3, but when TWD exceeds 31.33×108 m3, WDEI increases sharply and PW decreases slowly, with TWD increasing by 20×108 m3, WDEI increasing by about 9×108 m3, and PW decreasing by about 51×108 m3. The above relationships obey the law of diminishing marginal utility. As a part of PW, the water withdrawn from the Yangtze River represented by the marker colour needs to be appropriately pumped according to the natural flow and water demand of users. Generally, the proportion of water withdrawn from the Yangtze in PW cannot be too high, affecting the lake storage capacity and pumping (operating) cost. Therefore, the middle part or the lighter marker colour tends to represent better results in the figure. Meanwhile, to reduce the waste of water resources, the amount of abandoned water represented by the marker size should be reduced as much as possible. Therefore, the yellow marker point is obtained as the optimal operation strategy to compare with the historical operation strategy by multi-attribute decision-making. The weights of decision indicators and the results of the comparison are shown in Tables 3 and 4. Similarly, the Pareto front strategies in Fig. 5b and c also follow the above rules, but due to the difference in natural inflow, the specific data are also quite different (see Table 4).
Table 3Determining the weights of indicators for selecting the optimal operation strategy in typical wet, normal, and dry years.
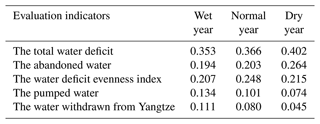
3.2 Comparison with historical operation strategy
In order to verify the rationality of the strategies obtained by the joint optimal operation model, this section compares the optimal operation strategy selected in different typical years with the historical operation strategy.
3.2.1 Comparison of the main operation performance indicators
Table 4 shows the optimal historical operation strategy in typical years. Compared with the optimal operation strategy, the TWD and WDEI of the historical operation strategy are very high, which means that the water deficit concentration in the historical operation strategy leads to the inability to reduce the TWD. Meanwhile, the PW and abandoned water are slightly large, indicating that the historical operation strategy has more disadvantages on economic and ecological benefits. WDEI can directly reflect the difference of water deficit among users. WDEI has sound optimization effects, of which the reduction in the optimal operation strategy was 94.2 % (81.8 %, 76.7 %) of the historical values in the typical normal year (wet year and dry year). The optimized operation strategy can significantly reduce TWD and PW by 82.06 % (37.69 %, 52.36 %) and 45.13 % (3.25 %, 21.51 %) compared with the historical values, respectively, while maintaining a very low WDEI. It has been demonstrated to be an excellent strategy for inter-basin water diversion, which can make multiple users share the risk of water deficit and alleviate the problem of water deficit concentration. The reduction of the abandoned water and the water withdrawn from the Yangtze River represents an increase in the lake storage capacity. The amount of abandoned water of the operation strategy is greatly reduced, and the amount of abandoned water is only 0.051×108 m3 in the dry year. This indicates that the optimal operation can maximize the utilization efficiency of the limited water resources. However, the amount of water withdrawn from the Yangtze River increased by 10.72×08 m3 after optimization in dry years, indicating that the proportion of the water withdrawn from Yangtze River in PW was raised in the optimal operation strategy to ensure uniform distribution of water and higher economic benefits.
3.2.2 Comparison of water deficit in water users
The comparison of water deficit in water users between the optimal operation strategy and the historical operation strategy in 3 typical years is illustrated in Table 5, which more intuitively reflects the optimization effect of WDEI. The “variance” and “decrement” represent the difference between the maximum and minimum values and the percentage reduction, respectively. The study found that after optimization, the variance of the users was reduced by 62.93 % (78.07 %, 54.09 %) in different typical years (i.e. bold font in Table 5). The Feihuanghe (FHH) user, Siyang–Zaohe (S-Z) user, and Hongzehu (HZH) user, which have a large water deficit in the historical operation strategy, decrease by 14.63 (14.41, 14.69) × 108 m3, 5.61 (9.33, 1.77) × 108 m3, and 0.98 (7.98, 5.26) × 108 m3 of the optimal operation strategy, respectively (see Table 5). The possible reason for the large water deficit of users is that they are far away from the main lakes and rivers or that there are many users on the water transmission route that need to be supplied. Users jointly share the water deficit risk in the optimal operation strategy in the case of little natural flow. The difference between the maximum and minimum water deficit is controlled within 8×108 m3, where the difference in the normal year is only 3.13×108 m3, and the problem of water deficit concentrated in a particular user is alleviated. For example, in the dry year, the water deficit of users under optimal operation strategy ranges from 0 to 7.64×108 m3. Although the water deficit of the Gaoliangjian–Huaian (GLH-HA) user, Luomahu (LMH) user, and Weishanhu (WSH) user is slightly higher after optimization, the total water deficit is much lower than the historical value and is not concentrated in the FHH user, HZH user, and S-Z user, compared with the results of historical operation. Overall, the optimal operation strategy is more reasonable and may accord with the aspirations of both the government and the general public.
3.2.3 Comparison of the operation water level of the Hongze and Luoma lake
Figure 6 shows the water level variations of HZ Lake and LM Lake (two major storage lakes in Jiangsu Province). The water level of HZ Lake and LM Lake is slightly higher than the historical value after optimization for most of the time. Both lakes show a regular trend of change: the lake remains at a high level during the dry season, releases water before the flood season, then remains at a low level throughout the flood season for storing flood, and returns to a high level at the end of the flood season. Therefore, the water transmission system can simultaneously implement lake water storage in the flood and non-flood seasons to ensure the fulfilment of water supply tasks and improve the utilization efficiency of water resources.
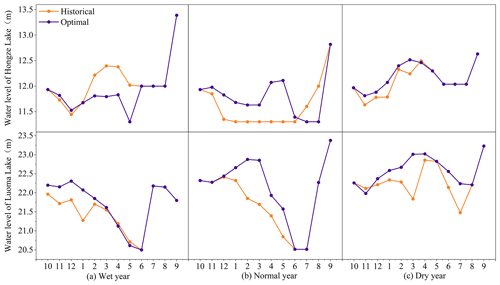
Figure 6The operation water level of the Hongze and Luoma lake for (a) wet, (b) normal, and (c) dry years under historical (orange line) and optimal (purple line) operation.
The optimized water level of HZ Lake was significantly lower than the historical value from February to June in Fig. 6a. This is due to the large amount of natural precipitation with uneven spatial–temporal distribution in the wet year, and the discharge time and discharge volume of HZ Lake are unreasonable in historical operating strategy, resulting in a large amount of water deficit and abandonment. Therefore, in the optimized operation strategy, a certain amount of lake water is discharged during the impoundment period for water supply. Similarly, LM Lake level in April–June is below the historical level. In Fig. 6b and c, the optimized HZ Lake level is significantly higher than the historical value before the flood season, while the flood season is decreased dramatically, indicating that the lake stores a certain amount of water while supplying water during the impoundment period and discharges water before the flood season to ensure that the flood season is at a safe level. Similarly, for LM Lake, the water level increased after optimization in typical years and tended to be consistent after the flood season.
Compared with the historical operation strategy, the water level of HZ Lake and LM Lake is slightly higher than the historical value after optimization for most of the time. Combined with the results of Tables 4 and 5, the coordinated water diversion between LM Lake and HZ Lake increased obviously while reducing TWD and PW.
3.2.4 Comparison of the water distribution of different routes between two adjacent lakes
This paper considers that the water distribution of different routes between two adjacent lakes is an important factor affecting the water deficit in the users. The water transmission capacity of the route is mainly affected by the water demand of users and the pumping capacity of the pumping station along the way. Table 6 shows the distribution of different water transmission routes between neighbouring lakes in typical years. The ratio of water pumped from LM Lake into Hanzhuangyun River and Bulao River is decreased from 19:8 to 1:1, which is convenient for the simultaneous double-route water supply outside the province and reduces the water supply pressure of Hanzhuangyun River. The ratio of water from the Zhongyun River and the Xuhong River to the LM Lake increased from 7:9 to about 7:1, gradually shifting the focus of the water transmission route to the Zhongyun River. The reason is that Hongze Lake transports water to the Zhongyun River through the Erhe and Gaoliangjian sluice without pumping, which can reduce the pumped water and save project cost. In addition, there are many users in Zhongyun River, the water demand is higher. When the water supply is sufficient, the drainage through Huaiyin, Yanhe and Yangzhuang sluice significantly reduces the water deficit of FHH and LYG users (see Table 5). Similarly, the ratio of water from HZ Lake to Zhongyun River and Xuhong River increased from 6:1 to 14:1. The ratio of pumping water from Liyun River and Jinbao Waterway to HZ Lake also increased from 19:1 to 25:1, indicating that the water transport efficiency of Zhongyun River is higher, and the operation strategy should be based on water transfer from the central canal, with the western route as the auxiliary support route. Similarly, the ratio of pumping water from the Yangtze River to the Liyun River and the Jinbao Channel has also increased (see Table 6).
Inter-basin water transfer projects are widely used around the world and are also quite costly to construct. For instance, the Colorado River aqueduct cost approximately USD 3.5 billion (Witcher, 2017); the Australian Snowy Mountains Scheme was completed in 1974 at a cost of about USD 500 million (Pigram, 2000); and the South-to-North Water Diversion Project in China, as the largest and most expensive inter-basin water transfer system in the world, is projected to cost USD 62 billion (Markosov, 2014). The installation and operation maintenance costs are also significantly high. Of this, the investment for just the Eastern Route of the project is around USD 1 billion (Liu et al., 2022), which is a typical example of a vast and complex water transfer system and has a certain degree of representativeness. Therefore, the Jiangsu section of the Eastern Route of the South-to-North Water Diversion Project is selected as the study area for this paper.
The joint optimal operating model is operated based on ecological (the total water deficit), social demand (the evenness of water deficit), and economic (the pumping water) objectives, focusing on the issue of water deficit concentration. Herein, the limited available water is used to minimize the total water deficit of the system and water deficit differences between users and applies to inter-basin water transfer projects with complex systems and a large number of water users. The multi-attribute decision implemented in this study incorporates ecological (the abandoned water) and the water withdrawn from Yangtze River into the multi-attribute decision indicator set, which can provide optimal operation strategy with preferred weights for decision makers who have different preferences.
Evidence from this research suggests that the water extracted from Hongze Lake is much greater than that from other lakes, indicating that Hongze Lake is the main source of water to support water supply and flood control within the system. Therefore, it is important to understand the water storage period. It is important to ensure that the water level of each storage lake reaches the water level at the end of the flood season to complete the water allocation in the non-flood season. If the reservoir water storage is insufficient, the Yangtze River will be pumped in time to ensure the normal operation of the entire water diversion project. The Yangtze River is the main source of water outside the J-SNWDP system. The proportion of pumping water from the Yangtze River in the pumping water volume of the system plays an important regulatory role. The actual operation should follow the following priority rules: when the natural inflow is less, pumping should mainly occur in the Yangtze River to complete the task of water supply. Conversely, when the natural inflow is large, the water withdrawn from Yangtze River is reduced, and the focus of water allocation is shifted to the mutual replenishment between lakes.
This paper has revealed that the water deficit does not only occur under less natural inflow conditions, but also there are still serious problems of water deficit and abandonment in wet years. The main reasons for the coexistence of water deficit and water abandonment are lakes' limited water storage capacity and the uneven spatial–temporal distribution of natural inflow. Water deficit and the pumped water are greatly affected by natural inflow; we should not expect to find a general operating strategy optimal in all natural conditions. This paper proposes an optimal operation strategy that considers the evenness of water deficit, accounting for the hydrological conditions of 3 typical years (i.e. wet years, normal years, and dry years). This strategy performs well in water resources allocation and utilization, which is representative and universal. However, due to the impact of global climate change, future runoff is highly uncertain, necessitating further discussion. Moreover, the Eastern Route of the South-to-North Water Diversion Project is a large-scale inter-basin water transfer project that spans provinces such as Jiangsu and Shandong, and it involves numerous uncertain factors. These include changes in biological communities, hydrological variations, and dynamic changes in water demand caused by extreme events, all of which add complexity to determining the water supply capacity of the project.
Regarding the two major regulating reservoirs within Jiangsu, Hongze, and Luoma lakes, the operational control water levels used in this paper were approved and established by the Ministry of Water Resources of China in 1954. Over the years, with socio-economic development, changes in the South-to-North Water Diversion Project, and the flood control capabilities of the lakes themselves, the original flood limit water levels have become inadequate for current development needs. This paper proposes the idea of appropriately raising the flood limit water levels of the lakes during the flood season, but the specific values require further research, incorporating runoff forecasting, flood risk early warning, and other factors into future studies of inter-basin water transfer.
Furthermore, this paper places greater emphasis on the positive impacts of inter-basin water transfer on social demands and ecology, with less focus on the analysis of economic costs. Due to the variability in water prices across different regions and the ongoing changes in economic development in recent years, the cost of the project is currently represented by the volume of water pumped and has not been converted into actual cost prices. Future studies will delve into the dynamic adjustment mechanisms of water pricing; subsequently analysing the economic benefits of the project is worthy of further study.
In summary, the optimal operation strategy studied in this paper can generally alleviate the concentration of water deficit under most natural inflow conditions. However, faced with complex and changeable natural and social conditions in the future, and in addition to further research on the operational strategies and sources of uncertainty, appropriate engineering measures should also be implemented. For instance, the flood control limit water level should be increased appropriately according to the natural inflow to improve the lake's storage capacity in the flood season. This is also a potential way to effectively enhance the operational efficiency of inter-basin water transfer project.
Scientific operational decisions in inter-basin water transfer projects are important for improving the water allocation balance and reducing the stress of concentrated water deficits. As the largest and most heavily invested inter-basin water transfer project in the world, the South-to-North Water Transfer Project, has been selected as the research area for this paper, focusing specifically on the Jiangsu section.
-
From the perspectives of social demand, economy and ecology, this paper establishes a joint optimal operation model for the Jiangsu section of the South-to-North Water Diversion Project (J-SNWDP) and further uses a combination of NSGA-III algorithm and multi-attribute decision-making for strategy preference, which is widely applied and reliable. This method shows a good performance in solving the complex water transfer problems with multiple objectives and engineering units and is currently less applied.
-
After incorporating the water deficit evenness index into the model, the concentration of water deficit is reduced by 94.2 % (81.8 %, 76.7 %) in typical wet years (normal year and dry year) compared with the historical strategy, which greatly ameliorated the engineering problem of user water deficit concentration. The other two indicators of the model, total water deficit (TWD) and pumping water (PW), were reduced by 82.1 % (37.7 %, 52.4 %) and 45.1 % (3.2 %, 21.5 %), respectively, with excellent performance.
-
After optimization, the rising trend of water level in Hongze Lake and Luoma Lake reflects the enhanced storage capacity of the lake, and the water allocation between different water transmission routes is more balanced, which improves water utilization and water supply efficiency. Moreover, this paper proposes water transfer prioritization rules and suggests appropriately increasing the flood control limit water level, aimed at protecting the water diversion and enhancing operational efficiency. The sources of uncertainty, such as natural inflow, water quality, and societal water demand, are worthy of further study.
Overall, the successful application of the optimal operation strategy in the Jiangsu section of the South-to-North Water Transfer Project also demonstrates the feasibility of the research. It is hoped that this method can be attempted in other similar watersheds worldwide in order to revalidate this method in other circumstances and demonstrate its universality. This would provide the scientific basis and operating suggestions for inter-basin water diversion projects.
Data will be made available on request.
BYZ: writing (original draft), validation, formal analysis, software, methodology, conceptualization, visualization, term definition. GHF: writing (review and editing), funding acquisition, resources, supervision, project administration. XL: resources, writing (review and editing), visualization, investigation. JZ: writing (review and editing), supervision, formal analysis. HYZ: resources, data curation, visualization, investigation.
The contact author has declared that none of the authors has any competing interests.
Publisher's note: Copernicus Publications remains neutral with regard to jurisdictional claims made in the text, published maps, institutional affiliations, or any other geographical representation in this paper. While Copernicus Publications makes every effort to include appropriate place names, the final responsibility lies with the authors.
The authors would like to thank the Jiangsu Province Water Science and Technology Key Projects for providing data support that made the study possible. We thank Guohua Fang, Xin Li, Jian Zhou, Huayu Zhong, Min Yan, two anonymous reviewers, and the editor for their help in improving the quality of the article.
This research has been supported by the Jiangsu Province Water Science and Technology Key Projects (grant no. 2020005).
This paper was edited by Yongping Wei and reviewed by two anonymous referees.
Deb, K. and Jain, H.: An Evolutionary Many-Objective Optimization Algorithm Using Reference-Point-Based Nondominated Sorting Approach, Part I: Solving Problems With Box Constraints, IEEE T. Evol. Comput., 18, 577–601, https://doi.org/10.1109/tevc.2013.2281535, 2014.
Florke, M., Schneider, C., and McDonald, R. I.: Water competition between cities and agriculture driven by climate change and urban growth, Nat. Sustain., 1, 51–58, https://doi.org/10.1038/s41893-017-0006-8, 2018.
Gan, Z. G., Zhao, H. L., Jiang, Y. Z., You, J. J., and Kang, J.: River Basin Water Resources Multi-Objective Regulation Theory And Method Research, in: International Conference on Green Building, Materials and Civil Engineering (GBMCE 2011), 22–23 August 2011, Shangri La, Peoples Republic of China, 71–78, https://doi.org/10.4028/www.scientific.net/AMM.71-78.4721, 2011.
Ghoreishi, M., Elshorbagy, A., Razavi, S., Bloeschl, G., Sivapalan, M., and Abdelkader, A.: Cooperation in a transboundary river basin: a large-scale socio-hydrological model of the Eastern Nile, Hydrology and Earth System Sciences, 27, 1201-1219, 10.5194/hess-27-1201-2023, 2023.
Guo, X. Y., Ma, C., and Tang, Z. B.: Multi-Timescale Joint Optimal Dispatch Model Considering Environmental Flow Requirements for a Dewatered River Channel: Case Study of Jinping Cascade Hydropower Stations, J. Water Resour. Pl. Manage., 144, 13, https://doi.org/10.1061/(asce)wr.1943-5452.0000981, 2018.
Guo, Y. C. and Li, J. L.: Practice and Improvement of Agent Construction System in Hydraulic Engineering, in: 2nd International Conference on Civil Engineering and Transportation (ICCET 2012), 27–28 October 2023 Guilin, Peoples Republic of China, 2611–2615, WOS:000317696001196, https://doi.org/10.4028/www.scientific.net/AMM.256-259.2611, 2013.
Guo, Y. X., Tian, X., Fang, G. H., and Xu, Y. P.: Many-objective optimization with improved shuffled frog leaping algorithm for inter-basin water transfers, Adv. Water Resour., 138, 103531, https://doi.org/10.1016/j.advwatres.2020.103531, 2020.
Gupta, J. and van der Zaag, P.: Interbasin water transfers and integrated water resources management: Where engineering, science and politics interlock, Phys. Chem. Earth, 33, 28–40, https://doi.org/10.1016/j.pce.2007.04.003, 2008.
Kato, T. and Endo, A.: Contrasting Two Dimensions of Disaster-Induced Water-Shortage Experiences: Water Availability and Access, Water, 9, 16, https://doi.org/10.3390/w9120982, 2017.
Li, J. H., Lei, X. H., Qiao, Y., Kang, A. Q., and Yan, P. R.: The Water Status in China and an Adaptive Governance Frame for Water Management, Int. J. Environ. Res. Publ. Health, 17, 19, https://doi.org/10.3390/ijerph17062085, 2020.
Li, Y. Y., Cui, Q., Li, C. H., Wang, X., Cai, Y. P., Cui, G. N., and Yang, Z. F.: An improved multi-objective optimization model for supporting reservoir operation of China's South-to-North Water Diversion Project, Sci. Total Environ., 575, 970–981, https://doi.org/10.1016/j.scitotenv.2016.09.165, 2017.
Liu, C. M. and Zheng, H. X.: South-to-north water transfer schemes for China, Int. J. Water Resour. Dev., 18, 453–471, https://doi.org/10.1080/0790062022000006934, 2002.
Liu, D. C., Li, Y., Wang, P. F., Zhong, H. Q., and Wang, P.: Sustainable Agriculture Development in Northwest China Under the Impacts of Global Climate Change, Front. Nutr., 8, 8, https://doi.org/10.3389/fnut.2021.706552, 2021.
Liu, Y., Chong, F. T., Jia, J. J., Cao, S. L., and Wang, J.: Proper Pricing Approach to the Water Supply Cost Sharing: A Case Study of the Eastern Route of the South to North Water Diversion Project in China, Water, 14, 12, https://doi.org/10.3390/w14182842, 2022.
Lopez, J. C.: Interbasin water transfers and the size of regions: An economic geography example, Water Resour. Econ., 21, 40–54, https://doi.org/10.1016/j.wre.2017.10.005, 2018.
Lu, Y., Tian, F. Q., Guo, L. Y., Borzì, I., Patil, R., Wei, J., Liu, D. F., Wei, Y. P., Yu, D. J., and Sivapalan, M.: Socio-hydrologic modeling of the dynamics of cooperation in the transboundary Lancang-Mekong River, Hydrol. Earth Syst. Sci., 25, 1883–1903, https://doi.org/10.5194/hess-25-1883-2021, 2021.
Ma, T., Sun, S., Fu, G. T., Hall, J. W., Ni, Y., He, L. H., Yi, J. W., Zhao, N., Du, Y. Y., Pei, T., Cheng, W. M., Song, C., Fang, C. L., and Zhou, C. H.: Pollution exacerbates China's water scarcity and its regional inequality, Nat. Commun., 11, 9, https://doi.org/10.1038/s41467-020-14532-5, 2020.
Markosov, A. I.: Does Transferring Water Present an Efficient Way of Solving Water Scarcity in China's Northeast?, University of Kansas, 2014.
McDonald, R. I., Weber, K., Padowski, J., Florke, M., Schneider, C., Green, P. A., Gleeson, T., Eckman, S., Lehner, B., Balk, D., Boucher, T., Grill, G., and Montgomery, M.: Water on an urban planet: Urbanization and the reach of urban water infrastructure, Global Environ. Change, 27, 96–105, https://doi.org/10.1016/j.gloenvcha.2014.04.022, 2014.
Medeiros, P. and Sivapalan, M.: From hard-path to soft-path solutions: slow-fast dynamics of human adaptation to droughts in a water scarce environment, Hydrolog. Sci. J., 65, 1803–1814, https://doi.org/10.1080/02626667.2020.1770258, 2020.
Nazemi, A. and Wheater, H. S.: On inclusion of water resource management in Earth system models – Part 2: Representation of water supply and allocation and opportunities for improved modeling, Hydrol. Earth Syst. Sci., 19, 63–90, https://doi.org/10.5194/hess-19-63-2015, 2015.
Ni, X. K., Dong, Z. C., Xie, W., Jia, W. H., Duan, C. G., and Yao, H. Y.: Research on the Multi-Objective Cooperative Competition Mechanism of Jinsha River Downstream Cascade Reservoirs during the Flood Season Based on Optimized NSGA-III, Water, 11, 20, https://doi.org/10.3390/w11040849, 2019.
Peng, Y., Chu, J. G., Peng, A. B., and Zhou, H. C.: Optimization Operation Model Coupled with Improving Water-Transfer Rules and Hedging Rules for Inter-Basin Water Transfer-Supply Systems, Water Resour. Manage., 29, 3787–3806, https://doi.org/10.1007/s11269-015-1029-4, 2015.
Pigram, J. J.: Options for rehabilitation of Australia's Snowy River: An economic perspective, Regul. Rivers-Res. Manage., 16, 363–373, https://doi.org/10.1002/1099-1646(200007/08)16:4<363::Aid-rrr610>3.0.Co;2-i, 2000.
Pohlner, H.: Institutional change and the political economy of water megaprojects: China's south-north water transfer, Global Environ. Change, 38, 205–216, https://doi.org/10.1016/j.gloenvcha.2016.03.015, 2016.
Rossi, G. and Peres, D. J.: Climatic and Other Global Changes as Current Challenges in Improving Water Systems Management: Lessons from the Case of Italy, Water Resources Management, 37, 2387-2402, 10.1007/s11269-023-03424-0, 2023.
Sun, S., Zhou, X., Liu, H. X., Jiang, Y. Z., Zhou, H. C., Zhang, C., and Fu, G. T.: Unraveling the effect of inter-basin water transfer on reducing water scarcity and its inequality in China, Water Res., 194, 10, https://doi.org/10.1016/j.watres.2021.116931, 2021.
Tang, X. Y., He, Y., Qi, P., Chang, Z. H., Jiang, M., and Dai, Z. B.: A New Multi-Objective Optimization Model of Water Resources Considering Fairness and Water Shortage Risk, Water, 13, 18, https://doi.org/10.3390/w13192648, 2021.
Tian, H. G.: Research on Scientific Development Strategy of Water Conservancy Economy Based on Sustainable Development, in: 3rd International Conference on Social Science, Management and Economics (SSME), 13–14 May 2017, Guangzhou, Peoples Republic of China, 476–481, WOS:000426950600086, 2017.
Vogel, R. M., Lall, U., Cai, X. M., Rajagopalan, B., Weiskel, P. K., Hooper, R. P., and Matalas, N. C.: Hydrology: The interdisciplinary science of water, Water Resour. Res., 51, 4409–4430, https://doi.org/10.1002/2015wr017049, 2015.
Wang, L. Z., Fang, L. P., and Hipel, K. W.: Basin-wide cooperative water resources allocation, Eur. J. Oper. Res., 190, 798–817, https://doi.org/10.1016/j.ejor.2007.06.045, 2008.
Wei, Y. P., Wei, J., Li, G., Wu, S. L., Yu, D., Ghoreishi, M., Lu, Y., Souza, F. A. A., Sivapalan, M., and Tian, F. Q.: A socio-hydrological framework for understanding conflict and cooperation with respect to transboundary rivers, Hydrol. Earth Syst. Sci., 26, 2131–2146, https://doi.org/10.5194/hess-26-2131-2022, 2022.
Witcher, T. R.: The Colorado River Aqueduct, Civ. Eng., 87, 46–49, https://doi.org/10.1061/ciegag.0001187, 2017.
Xu, J. P., Tu, Y., and Zeng, Z. Q.: Bilevel Optimization of Regional Water Resources Allocation Problem under Fuzzy Random Environment, J. Water Resour. Pl. Manage., 139, 246–264, https://doi.org/10.1061/(asce)wr.1943-5452.0000248, 2013.
Zhang, C., Wang, G. L., Peng, Y., Tang, G. L., and Liang, G. H.: A Negotiation-Based Multi-Objective, Multi-Party Decision-Making Model for Inter-Basin Water Transfer Scheme Optimization, Water Resour. Manage., 26, 4029–4038, https://doi.org/10.1007/s11269-012-0127-9, 2012.
Zhou, B. W., Liu, B. Y., Yang, D. S., Cao, J., and Littler, T.: Multi-objective optimal operation of coastal hydro-electrical energy system with seawater reverse osmosis desalination based on constrained NSGA-III, Energ. Convers. Manage., 207, 15, https://doi.org/10.1016/j.enconman.2020.112533, 2020.
Zhu, X. P., Zhang, C., Yin, J. X., Zhou, H. C., and Jiang, Y. Z.: Optimization of Water Diversion Based on Reservoir Operating Rules: Analysis of the Biliu River Reservoir, China, J. Hydrol. Eng., 19, 411–421, https://doi.org/10.1061/(asce)he.1943-5584.0000805, 2014.
Zhuan, X. T., Li, W., and Yang, F.: Optimal operation scheduling of a pumping station in east route of South-to-North water diversion project, Energ. Proced., 105, 3031–3037, https://doi.org/10.1016/j.egypro.2017.03.623, 2016.