the Creative Commons Attribution 4.0 License.
the Creative Commons Attribution 4.0 License.
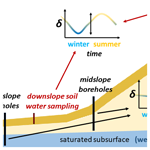
Young and new water fractions in soil and hillslope waters
Marius G. Floriancic
Scott T. Allen
James W. Kirchner
The transport processes and corresponding timescales of water's infiltration into and percolation through the shallow subsurface are poorly understood. Here, we characterize the transport of recent precipitation through a forested hillslope using a continuous 3-year record of O and H stable isotopes in precipitation, streamflow, and soil waters from various depths. We found that the fractions of recent precipitation decreased with depth, both in waters extracted using suction-cup lysimeters and in waters extracted from bulk soil samples using cryogenic distillation. Fractions of recent precipitation found in soils and streamflow were much larger with wet antecedent conditions, showing that wet landscapes can transmit recent precipitation quicker than dry landscapes. Approximately 18 % of streamflow was younger than 2–3 months, 11 % was younger than 3 weeks, and 7 % was younger than 1 week; these new water fractions were similar to those seen in 20 to 80 cm deep soils. Mobile soil waters below 2 m depth contained much less recent precipitation (1.2 ± 0.4 % younger than 2 weeks) than streamflow did (12.3 ± 2.1%), indicating that they are not the dominant source of streamflow. Instead, streamflow must be generated from a mixture of deep subsurface waters, with very little isotopic seasonality and short-term variability, and shallow soil waters, with more pronounced isotopic seasonality and short-term variability. This study illustrates how flow, storage, and mixing processes linking precipitation to streamflow and evapotranspiration can be constrained by measuring isotopic variability across different hillslope positions, subsurface depths, and timescales.
- Article
(3753 KB) - Full-text XML
-
Supplement
(1936 KB) - BibTeX
- EndNote
A total of 40 % of the global ice-free landmasses (Waring and Running, 2007) are covered by forests. Thus, the modulation of input precipitation in forests is of great importance to the global freshwater cycle. While it is generally known that forest soils play an important role in the hydrological cycle by controlling infiltration and percolation to deeper storage (Sprenger et al., 2016), we have limited understanding of water movement in forested hillslopes from shallow subsurface storages to deeper storages or streamflow.
What we do know about subsurface water transport in forested hillslopes has been largely derived from stable isotopes and other tracers. For example, streamflow responds quickly to rainfall inputs even though it may be mostly composed of old waters released from subsurface storage (i.e., the so-called “old-water paradox”; Kirchner, 2003; Neal and Rosier, 1990). More recently, hydrologists have recognized that water stored in the subsurface is often much older than the water draining from those same subsurface storages (Berghuijs and Kirchner, 2017; Kirchner et al., 2023). Whereas most groundwater storages are dominated by waters with ages of 10 years or more (Jasechko et al., 2017), 25 % of global streamflow is younger than 2 to 3 months (Jasechko et al., 2016). While these apparent paradoxes are explainable (Berghuijs and Kirchner, 2017), we have few insights regarding where in the subsurface these age contrasts arise.
Water movement within shallower subsurface storages is often conceptualized as piston flow (recent infiltration partly displacing and mixing with stored water; e.g., Hewlett and Hibbert, 1967) or as preferential flow (bypassing the stored soil waters in the top layers; e.g., Beven and Germann, 1982). Piston flow would always result in more recent precipitation being closer to the surface, and preferential flow would result in recent precipitation also reaching deeper depths, potentially without substantially mixing with waters stored in the intervening layers (i.e., bypass flow, a special case of preferential flow). Bypass flow is often argued to be responsible for ecohydrological separation (Brooks et al., 2010), but it remains unclear how frequently bypass flow occurs. Thus, it also remains unclear how much waters flowing via macropores interact with waters within the soil matrix, especially under different soil wetness conditions; numerous previous studies found contrasting results regarding the degree of interaction between waters flowing through soils and the waters stored in them (e.g., Geris et al., 2015; Goldsmith et al., 2012; Hervé-Fernández et al., 2016). However, seasonal and event isotope signals often become more damped with depth, indicating that the infiltrating water becomes well mixed as it percolates to deeper depths (e.g., Sprenger et al., 2019a, b; Barbecot et al., 2018). While such phenomena have typically been investigated through sampling vertical profiles, it remains unclear how much these profiles are affected by lateral-flow processes, which could also lead to damping of isotopic signals. The question that needs to be answered is as follows: how do subsurface transport processes yield the old, well-mixed waters often seen in streamflow?
To explore this question, we examine how precipitation events infiltrate into the subsurface by analyzing time series of stable water isotopes at various hillslope positions and depths. Soils typically carry the isotopic signature of many previous precipitation events. Due to seasonal isotopic signals in precipitation, with isotopically heavier precipitation in summer and lighter precipitation in winter, we can track precipitation from different seasons in groundwaters (Jasechko, 2019; Jasechko et al., 2014), streamflow (Allen et al., 2019), and soils (Sprenger et al., 2019a). We can use the seasonal fluctuations in precipitation to assess the relative proportions of younger and older water in streamflow. Specifically, the fraction of young water, defined as the fraction of streamflow that is younger than approximately 2–3 months, can be inferred from the amplitude of seasonal tracer cycles in precipitation and streamflow (Kirchner, 2016a, b). Alternatively, the fractions of new water, defined as the fraction of water that is new since the last sampling, can be inferred from ensemble hydrograph separation (Kirchner, 2019; Knapp et al., 2019; Kirchner and Knapp, 2020) of tracer time series. Both methods have been widely applied to streamflow time series (e.g., Ceperley et al., 2020; Jasechko et al., 2016; Gentile et al., 2023; von Freyberg et al., 2017, 2018; Knapp et al., 2019; Floriancic et al., 2024b), but few studies have applied them to time series of soil waters (Gallart et al., 2020; Burt et al., 2023). Both of these stable-isotope tools can reveal how precipitation mixes with older storages as it travels down the soil profile and toward streams.
Here, we use a 3-year continuous dataset of precipitation, mobile and bulk soil water, deep mobile water from boreholes, and streamflow to identify how the partitioning of young and new waters changes across different depths of a forested hillslope. We address the following research questions:
-
How much of the streamflow and soil waters at different depths consist of young water (i.e., water that is younger than approximately 2–3 months) and new water (i.e., younger than 1 d to younger than 3 weeks, depending on the sampling interval)?
-
To what extent do we find higher fractions of new water with wetter antecedent conditions?
-
Are the isotopic signals in subsurface waters variable along the hillslope and what do they reveal about streamflow generation?
2.1 Assessment of young water fractions (Fyw) and new water fractions (Fnew)
In seasonal climates, the ratio of stable water isotopes (O and H) in precipitation differs between summer and winter and also varies among individual precipitation events. Typically, precipitation in continental interiors is isotopically heavier in summer than in winter, resulting in a seasonal cycle of precipitation isotopes. Kirchner (2016a, b) developed a method to calculate the so-called young water fraction Fyw, the fraction of streamflow that is younger than 2–3 months, from the ratio of the amplitudes of the seasonal isotopic cycles in streamflow and precipitation. These amplitudes (and the phase shift between the seasonal cycles) can be inferred from fitting sinusoids with a pre-set frequency of 365 d to the isotope time series using an iteratively re-weighted least-squares (IRLS) approach (see the R script provided in the Supplement of von Freyberg et al., 2018). We calculated the seasonal cycle amplitudes for the precipitation, mobile-soil-water, bulk-soil-water, and streamflow time series to estimate the young water fractions of water in soils and the stream.
In addition to young water fractions Fyw, we also calculated new water fractions Fnew via ensemble hydrograph separation as described in Kirchner (2019). The major difference between Fnew and Fyw is that, whereas Fyw estimates the fraction of streamflow or soil water that is younger than 2–3 months, Fnew estimates the average fraction of water that originates from precipitation between sequential pairs of sampling times (e.g., 3–4 d to 3 weeks for mobile soil waters, 2 to 3 weeks for bulk soil waters and deep mobile soil waters, and 1 d to 3 weeks for streamflow). The method is based on correlations between the fluctuating isotopic signals in precipitation, soil waters, and streamflow. It reveals the average contribution from an endmember (precipitation) to a mixture (soil water or streamflow) through correlations across multiple time steps. This also makes it insensitive to unknown or unmeasured endmembers. While traditional hydrograph separation assesses how fractions of new and old water change over time (e.g., during an individual storm event) for each time step, the ensemble hydrograph separation method (Kirchner, 2019) can estimate the average fractions of new and old water at different antecedent moistures and seasons. Scripts to perform this analysis in R and MATLAB are available in the Supplement of Kirchner and Knapp (2020).
2.2 Sampling and data collection
All analyses are based on data collected in a forested hillslope-to-creek transect in a small 0.3 km2 catchment (WaldLab Forest Experimental Site). This transect is dominated by spruce and beech trees and is part of the larger “Waldlabor Zürich” research and education initiative in Switzerland. This site, just north of Zurich, Switzerland, has a mean annual temperature of 9.3 °C and mean annual precipitation of 1134 mm (2010–2022). The soil is a Luvisol of approximately 100 cm depth on top of ∼ 6 m of moraine material from the Last Glacial Maximum. The dominant soil structure is silty sand, with clay fractions below 10 %.
Since March 2020, we have measured major climate parameters outside the forest with a compact all-in-one weather station (ATMOS 41 – Meter AG) at 10 min resolution. Precipitation isotope samples were collected on any day when a precipitation event larger 3 mm occurred using glass bottles with funnels and syringes to prevent evaporation and vapor diffusion (as described in von Freyberg et al., 2020). Discharge was measured at a V-notch weir at the outlet of our experimental catchment with a pressure sensor (KELLER AG – DCX-II) at 15 min resolution. Daily streamflow samples at the outlet of the catchment were obtained with an ISCO 6712 autosampler (Teledyne Inc.) equipped with evaporation protection as described in von Freyberg et al. (2020) using a daily mixture of four 100 mL samples of stream water taken every 6 h (midnight, 06:00, 12:00, and 18:00 CET).
Soil water was sampled by multiple methods. Suction-cup lysimeters were used to sample what is commonly referred to as “mobile soil water” (the fraction of soil water that is held cohesively and that can move freely), and bulk samples of soil were collected for water to be extracted by cryogenic distillation (assumed to comprise soil water in all capillary spaces, including the mobile soil water sampled by suction-cup lysimeters). We sampled mobile soil water (SWmobile) at 10, 20, 40, and 80 cm depths at two plots (Fig. 1) with suction lysimeters (SoilMoisture Equipment Corp., Slim Tube Soil Water Sampler). We applied a suction of 0.6 bar on Mondays and Thursdays and emptied the samplers twice a week on the following Thursdays and Mondays. In addition, we sampled approximately 15 mL of bulk soil (SWbulk) at the same two plots (Fig. 1) at 10, 20, 40, and 80 cm depths, matching the lengths of the suction cups of the mobile-soil-water samplers with a 2 cm wide auger every 3 weeks, and we extracted the bulk soil water cryogenically. Monitoring of mobile and bulk soil water at the shallower depths (10–40 cm) began in April 2020, and monitoring at 80 cm began in June 2021; thus the 80 cm records are significantly shorter. Additionally, monitoring of a third “downslope” plot (not shown in Fig. 1) began in April 2022, specifically to investigate lateral flows after the overall study began.
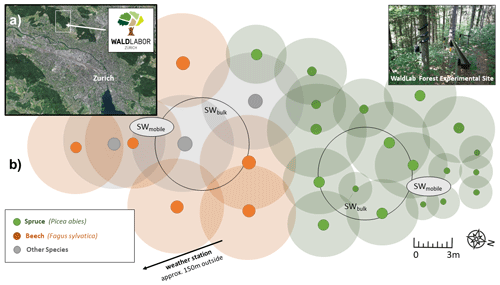
Figure 1Location of the “Waldlabor” in Zurich, Switzerland (a; source: Swisstopo) and a schematic diagram of the approximately 30 × 10 m study plot within the WaldLab Forest Experimental Site (b), indicating the locations of trees (spruce, beech, and other species shown in green, orange, and gray), as well as the locations of mobile (SWmobile) and bulk (SWbulk) soil water sampling. Precipitation for isotope analysis was sampled outside the forest perimeter at the weather station at approximately 150 m distance from the site. The gauge of the Holderbach creek is located approximately 90 m from the experimental site at the bottom of the hillslope. A more detailed map of the WaldLab Forest Experimental Site can be found in Fig. S1 in the Supplement.
Beyond those soil water collections, we also collected deep mobile waters from soil and the underlying moraine in 11 boreholes, screened between approximately 1 and 6 m depth, every 2 weeks. These boreholes were drilled in November 2020, and soil and sediment samples (for cryogenic water extraction) at different depths were collected into exetainers (Labco Ltd., 12 mL Exetainer) and stored at −18 °C for cryogenic extraction. Any water that collected in the bottom of these boreholes was sampled every 2 weeks, representing a seepage flux that is comparable to what can be collected by zero-tension lysimeters.
Cryogenic vacuum distillation was performed at the Institute of Agricultural Sciences Stable Isotope Lab at ETH Zurich (Grassland Science Group). The samples were evaporated in a water bath at a temperature of 80 °C for 3 h with a suction of 10−2 MPa, and the resulting vapor was trapped in u-shaped tubes immersed in liquid nitrogen (Sun et al., 2022). We did not assess the extraction efficiency explicitly in this study; however, the same system was recently tested in another study (Bernhard et al., 2024), showing that extraction efficiencies exceeded 98 % for 89 % of the samples and were between 96 % and 98 % for the remaining 11 % of the samples. Extracted samples and all other samples (precipitation, mobile soil water, deep mobile borehole water, and stream water) were stored in 1.5 mL glass vials (BGB Analytik) refrigerated at 2 °C until analysis. The isotopic composition was analyzed with a triple-isotope water analyzer (Los Gatos – TIWA-45-EP), with a precision of < 1 ‰ for 2H and < 0.2 ‰ for 18O, as determined by long-term replicate sampling of standards. All isotope data are reported in per-mille (‰) notation relative to V-SMOW (Vienna Standard Mean Ocean Water) of δ2H; the respective δ18O plots can be found in the Supplement.
Because the different types of samples were collected at different time intervals (i.e., after each event for precipitation, daily for streamflow, twice a week for mobile soil waters, every 2 weeks for deep mobile borehole waters, and every 3 weeks for bulk soil waters), analyses involving comparisons of those samples to precipitation always used volume-weighted means of precipitation isotope values that were aggregated to reflect the same sampling intervals.
3.1 Seasonal signals in precipitation and streamflow
The seasonal isotopic variation in streamflow was much smaller than that in precipitation (see amplitudes of the sinusoidal cycles in Table S1 in the Supplement), implying that the isotopic signal in precipitation was damped by storage and mixing on its way to becoming streamflow (shown for δ2H in Fig. 2 and for δ18O in Fig. S1). While precipitation isotopes did contain the expected typical seasonal cycle of lighter isotopic signatures during the winter months and heavier isotopic signatures during the summer months, this seasonal cycle was much less pronounced in the streamflow isotope values. The median δ18O isotope values were −9.2 ‰ and −9.4 ‰ for precipitation and streamflow, respectively. Thus, overall streamflow was isotopically slightly lighter than precipitation, indicating that streamflow contained relatively more winter precipitation than summer precipitation.
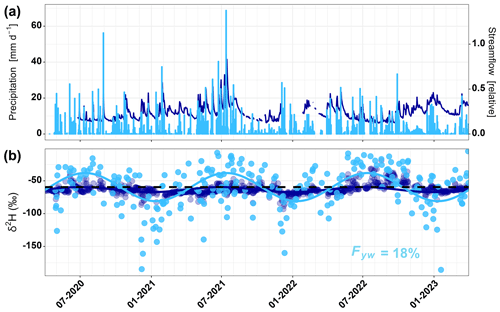
Figure 2Time series of precipitation (light blue) and streamflow (dark blue) (a) and their δ2H isotopic compositions (b) from April 2020 until March 2023. Sinusoidal cycles were fitted to the isotope data using iteratively re-weighted least-squares regression (in light blue for precipitation isotopes and in dark blue for streamflow isotopes). The dashed black line indicates the volume-weighed mean isotopic composition of precipitation; streamflow samples lying above and below this line indicate dominance of summer and winter precipitation, respectively. The seasonal cycles of the stream water isotopes are damped relative to the precipitation isotopic cycles due to storage and mixing in the subsurface. The corresponding time series and sinusoidal fits for δ18O can be found in Fig. S1.
From the ratio of seasonal amplitudes in streamflow and precipitation isotopes (show in Table S1), we estimated the fraction of young water (i.e., water that is younger than approximately 2–3 months, Fyw) in streamflow to be 18 %. Thus, most of the streamflow at our site originated from water that is stored in the subsurface for longer than 2–3 months. This is not only true for our site but is also in line with findings from many other rivers across the globe (Jasechko et al., 2016; von Freyberg et al., 2018; Floriancic et al., 2024b). However, streamflow young water fractions by themselves cannot indicate where in the surface-to-stream transit pathway this damping of seasonal cycles occurs.
3.2 Seasonal signals and young water fractions across different depths in the subsurface
With increasing depth, observed sinusoidal cycles of soil water isotopes became increasingly damped relative to the precipitation input signal (Fig. 3). Values of Fyw in mobile soil water – i.e., water sampled by suction-cup lysimeters – exhibited little variation in the top 40 cm (63 % at 10 cm depth to 68 % at 20 cm depth and 63 % at 40 cm depth) but decreased to 26 % at 80 cm depth. As with the isotope ratios seen in precipitation across seasons, soil water at all depths showed heavier isotopes in summer and lighter isotopes in winter. The lack of a distinctive phase shift between precipitation and soil water at shallower depths (i.e., 10 to 40 cm) presents an argument against the dominant role of piston flow and supports the interpretation that recent (∼ 2–3-month-old) precipitation is seen in these soils, although in fractions that indicate significant dilution by older water (even, surprisingly, at shallow depths). Soil waters at 80 cm were heavily damped and were typically lighter than average precipitation, indicating that recharge to these depths was overrepresented by winter precipitation.
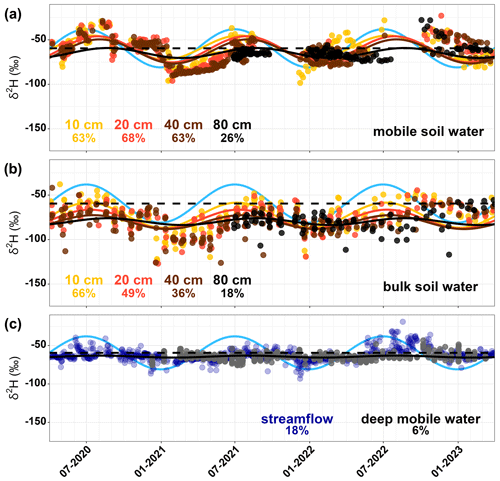
Figure 3Time series of the δ2H isotopic composition from April 2020 until March 2023 in mobile (a) and bulk soil waters (b) of 10, 20, 40, and 80 cm depth and in deep mobile waters (c) collected in boreholes of 2 to 6 m depth. Sinusoidal cycles were fitted to the isotope data using iteratively re-weighted least-squares regression. The light-blue line shows the sinusoidal cycle of precipitation. The percentages indicate the fractions of water younger than 2–3 months in the respective water pools. The dashed black line indicates the mean isotopic composition of precipitation; all samples above are dominated by summer precipitation, and all samples below are dominated by winter precipitation. The seasonal cycles of soil waters exhibit increasing damping with depth. The percentages indicate the fraction of young water for the different soil depths and streamflows. The time series and sinusoidal fits of the δ18O isotope signatures can be found in Fig. S2, and the fitting parameters can be found in Table S2.
Bulk soil Fyw values (assumed to comprise soil water in all capillary spaces, including the mobile soil water sampled by suction-cup lysimeters) were somewhat smaller than those of mobile soil water (Fig. 3). They decreased from 66 % at 10 cm depth to 49 % at 20 cm depth, 36 % at 40 cm depth, and 18 % at 80 cm depth, indicating greater dilution by old water with increasing depth (and a greater presence of old water in bulk soil than in mobile waters at all depths > 10 cm). During all seasons, bulk soil waters were typically lighter than average precipitation, showing that winter precipitation predominates in these pore spaces, even in mid-summer. Suction-cup lysimeters disproportionately sample larger pore spaces that more readily fill and drain (Weihermüller et al., 2005). Thus, while mobile waters in larger pores (sampled from suction-cup lysimeters) are filled by more recent precipitation, smaller pores at 20 to 80 cm depth are typically bypassed by recent precipitation and filled by waters predominantly originating from the winter season.
The seepage water collected from the boreholes at 2 and 6 m depth showed the least young water, with Fyw= 6 % (Fig. 3c). This is one-third of the Fyw observed in streamflow (∼ 18 %), implying that streamflow cannot be composed entirely of water from the deeper subsurface but must also contain shallower components with larger seasonal isotopic cycles.
3.3 Recent precipitation in soil waters inferred by new water fractions
New water fractions (Fnew) also decreased with increasing depth in the subsurface, with Fnew of the borehole deep-mobile-water seepage being much smaller than that of streamflow (Fig. 4, Table 1). Mobile soil waters were sampled twice a week (albeit with significant gaps in the data during the autumn 2021 and 2022 dry periods), and Fnew values calculated from these data reflect the fraction of soil water that is new on timescales of 3 to 4 d up to 3 weeks. This approach quantifies a much newer fraction of water than can be inferred from young water fractions (2–3 months).
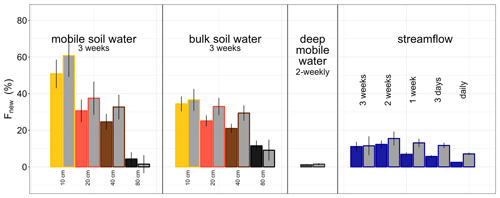
Figure 4New water fractions (Fnew) in mobile and bulk soil waters (younger than 3 weeks) at 10, 20, 40, and 80 cm depth; deep mobile soil water sampled from boreholes (younger than 2 weeks) and streamflow (for 3-week, 2-week, weekly, 3 d, and daily time step aggregations) for all sampling dates (in colors) and the 50 % wettest sampling dates (in shades). Fnew values are typically smaller at greater depths and larger following higher precipitation.
Table 1Fractions of waters younger than 2–3 months (Fyw) and Fnew for different time step aggregations for all sampling dates (in bold) and the wettest 50 % of sampling dates (in italics), including the respective standard errors.
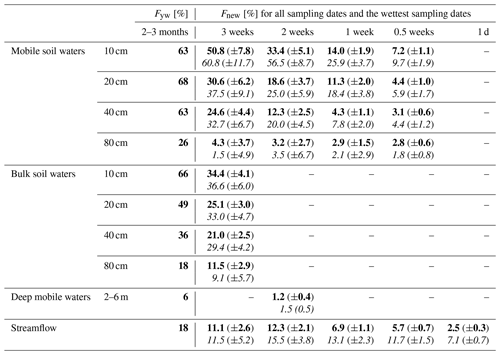
The fraction of new water since the last sampling (i.e.,younger than 3 to 4 d; Fnew) in mobile soil water (water extracted at a tension of < 0.6 bar) decreased from 7 % at 10 cm depth to 4 % at 20 cm depth, 3% at 40 cm depth, and 3 % at 80 cm depth. Calculating Fnew for the 50 % wettest sampling dates (based on total precipitation in the 3–4 d prior to sampling) reveals that mobile soil water contained more new water following wet antecedent conditions, i.e., 10 % at 10 cm depth, 6 % at 20 cm depth, 4 % at 40 cm depth, and 2 % at 80 cm (Table 1). We also calculated the fraction of water that was younger than 3 weeks in mobile soil water, which decreased from 51 % at 10 cm depth to 31 % at 20 cm depth, 25 % at 40 cm depth, and 4 % at 80 cm depth for all sampling dates and from 61 % at 10 cm depth to 38 % at 20 cm depth, 33 % at 40 cm depth, and 2 % at 80 cm depth for the wettest 50 % of sampling dates (Fig. 4). Thus, while approximately two-thirds of the mobile soil water at 10 to 40 cm depths was typically younger than 2 to 3 months (as indicated by Fyw – Fig. 3), relatively little of this water originated from the most recent precipitation (i.e., less than 3–4 d ago).
The Fnew in bulk soil waters (assumed to comprise soil water in all capillary spaces, including the mobile soil water sampled by suction-cup lysimeters), reflecting the fraction of water younger than 3 weeks (the sampling frequency of bulk soils), decreased from 34 % at 10 cm depth to 25 % at 20 cm depth, 21 % at 40 cm depth, and 12 % at 80 cm depth; for the 50 % wettest sampling dates, the corresponding 3-week Fnew values are 37 % at 10 cm depth, 33 % at 20 cm depth, 29 % at 40 cm depth, and 9 % at 80 cm depth (gray bars in Fig. 4). Thus, wet antecedent conditions increased the (3-week) fraction of new precipitation found in bulk soil waters.
The Fnew in deep mobile water at 2 to 6 m depths (reflecting the fraction of water younger than 2 weeks because this is the sampling interval in the deep boreholes) was very small (1.2 % and, for the 50 % wettest sampling dates, 1.5 %). Fnew in discharge (sampled every day) was calculated for 3-weekly, 2-weekly, weekly, 3 d, and daily aggregation intervals. Across all sampling dates, 11.1 % ± 2.6 of streamflow was younger than 3 weeks, 12.3 % ± 2.1 of streamflow was younger than 2 weeks, 6.9 % ± 1.1 was younger than 1 week, 5.7 % ± 0.7 was younger than 3 d, and 2.5 % ± 0.3 was younger than 1 d. Although it may seem surprising that the 3-week new water fractions, which include the 2-week new water fractions, might be smaller than the 2-week fractions, their uncertainties (shown in Table 1) imply that these values are indistinguishable. For the 50 % wettest sampling dates, 11.5 % ± 5.2 of streamflow was younger than 3 weeks, 15.5 % ± 3.8 of streamflow was younger than 2 weeks, 13.1 % ± 2.3 was younger than 1 week, 11.7 % ± 1.5 was younger than 3 d, and 7.1 % ± 0.7 was younger than 1 d (Table 1).
Building upon the comparison of wetter periods versus all data, we calculated the fraction of new water for different ranges of 3-week precipitation amounts. Figure 5 shows that Fnew in mobile and bulk soil waters, as well as in streamflow, increased with more precipitation in the month preceding the sampling. Fnew in mobile soil waters increased from 28 % to 68 % at 10 cm depth, from 18 % to 53 % at 20 cm depth, and from 19 % to 42 % at 40 cm depth. Fnew in bulk soil waters increased from 31 % to 36 % at 10 cm depth, from 23 % to 38 % at 20 cm depth, and from 10 % to 40 % at 40 cm dept and from 11 % to 15 % in streamflow. Unfortunately, we only started sampling bulk soil waters at an 80 cm depth 14 months later, resulting in a much shorter time series; thus, comparisons of Fnew for different precipitation intensities (as shown in Fig. 5 for the other sampling depths) were not reliable. These results reiterate the importance of catchment wetness for water transport and percolation.
3.4 Further interpretation of Fnew and Fyw in soils and streamflow
The data suggest that soil new water fractions decrease with depth and increase with antecedent wetness and that new water flowing to streams may largely bypass the deepest soil waters. Bypass flow is inferred to be dominant whenever pores are already filled with water, independent of the season. However, pores were observed to empty throughout the summer seasons, yielding the driest soils at the end of the growing season (in autumn). Overall, little summer precipitation makes it to the soil (due to interception and evaporation; see Sect. 3.4 and Floriancic et al., 2023). Therefore, deeper pores are predominately filled by the first available water to reach those depths after pores were emptied, which, in our case, is mostly winter precipitation (see also Floriancic et al., 2024a).
Approximately 18 % of streamflow was younger than 2–3 months (as estimated from Fyw – Fig. 2), 11 % was younger than 3 weeks, and 7 % was younger than 1 week (both estimated from Fnew – Fig. 4). Streams with similarly small fractions of young and new waters have been described elsewhere in the Alps (Floriancic et al., 2024b), in an Andean floodplain (Burt et al., 2023), and at Plynlimon in Wales (Knapp et al., 2019); such values are also common globally (Jasechko et al., 2016). The effect of antecedent wetness on the shorter transit times that we observed has also been quantified and observed in some of the abovementioned studies (Floriancic et al., 2024b; Knapp et al., 2019). While it may be intuitive that wet conditions allow for subsurface flow paths to connect pores, activate flow paths, and transmit recent precipitation more quickly to streams, our measurements show that the same occurs vertically in soils (Figs. 3, 4, 5). Those patterns, and even the Fnew values in soils of 40 to 80 cm depth, were relatively consistent with those in streamflow but not with the patterns and values observed in the deeper subsurface (i.e., deep mobile soil waters sampled from boreholes between 2 and 6 m depth). This indicates that stream water was predominantly not derived from mobile soil waters below 2 m depth. Instead, waters stored in our hillslope below 2 m depth were typically much older than waters draining from our hillslope (sensu age contrasts described in Berghuijs and Kirchner, 2017). That is to say that, while the isotopic signatures in soils between 10 and 80 cm suggest either piston flow or well-mixed transport, flows from these soil layers to the stream may largely bypass deeper storages.
There were large isotopic differences between the mobile fraction of soil water (sampled by suction-cup lysimeters) and the entirety of soil water (represented by bulk-soil-water signatures – see Fig. 3 and Sect. 3.2.). We found that bulk soil waters typically contained less young and new waters than mobile soil waters (Table 1). This is not surprising as one would expect that mobile water is more easily replaced by recent precipitation as it is less tightly bound in typically larger pores (compared to bulk soil waters that comprise soil water in all capillary spaces, including the mobile soil water sampled by suction-cup lysimeters). While, typically, around two-thirds of mobile soil waters at 10 to 40 cm depth were younger than 2–3 months (Fig. 3a), bulk soil waters at 20 to 80 cm depth were typically bypassed by recent precipitation and filled by waters predominantly originating from the winter season (Fig. 3b). At 3 to 4 d to 3-week timescales, however (i.e., Fnew in mobile soil waters – Fig. 4, Table 1), we found that soil new water fractions decreased with depth, indicating that percolation from shallower to deeper layers typically requires more than 3 weeks.
Our results also suggest that soil water signatures and the fractions of young and new soil waters are significantly altered by evaporation of intercepted precipitation and tree water uptake from specific pools. We hypothesize that forest trees, which preferentially access water in smaller pores (see discussion in Sprenger and Allen, 2020), cannot be responsible for emptying the bulk-soil-water stores because bulk soils contain less than 50 % young water (less than 2–3 months old) at all depths below 10 cm. Furthermore, the fact that bulk soil waters are systematically older than mobile soil waters at all depths below 10 cm implies that mobile soil waters must be largely bypassing the bulk-soil-water stores as they percolate through the profile. Although bulk soil waters also contain mobile soil waters, the relative fraction of bulk and mobile soil waters likely depends on soil wetness. As soils dry, the isotopic signal in bulk soil waters is presumably increasingly dominated by the waters stored in small pores that are not part of the pool of mobile soil water. From previous studies, we found that tree water uptake at our site predominantly occurs around 40 cm depth (Floriancic et al., 2024a; Martinetti et al., 2024), although the small fractions of young (< 2–3 months) and new (< 3 weeks) bulk soil water in this layer (Fyw= 36 % and Fnew= 21 %, respectively) indicate that any root water uptake is not primarily refilled by recent precipitation. By contrast, at 10 cm depth, the fractions of young water are large (> 60 %) and consistent between mobile and bulk soil water, indicating that waters at 10 cm removed by evaporation, root water uptake, and percolation to deeper layers are substantially replenished by recent precipitation, with less bypassing than observed in deeper layers. Exact inferences about transport processes from these types of data are complicated by the localized uptake of water at different depths, which is often ignored in hillslope- or catchment-scale transport models.
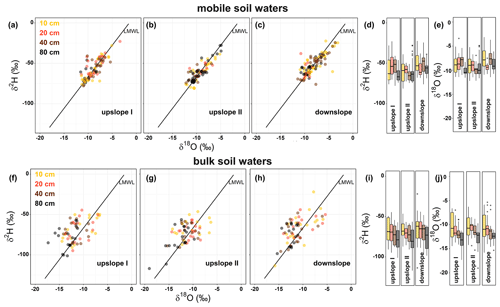
Figure 6Dual-isotope plots of mobile soil waters (a–e) and bulk soil waters (f–j) for the two sites on top of the hillslope (a, b, f, g) and for the downslope site (c, h), including their respective boxplots (d, e, i, j). Mobile soil waters were different between the upslope sites and the downslope location, whereas bulk soil waters were quite similar in all three sites. Local meteoric water lines (LMWLs) are calculated by reduced-major-axis regression (described in Harper, 2016) because uncertainties in both δ2H and δ18O are equally important, whereas classic linear-regression fitting assumes that the x axis data have no error or uncertainty.
A limitation in the inferences made from comparing isotopes in bulk and mobile soil waters is that they are influenced by different uncertainties. While mobile soil waters were always sampled at the same location throughout the observation period and thus reflect only the temporal variability of soil water isotopic signatures, bulk soil waters were sampled destructively at different locations in ∼ 8 m2 plots, thus reflecting both temporal and spatial variations in soil water isotopes. Another difference is that bulk soil water was extracted via cryogenic distillation, which introduces additional uncertainties (Orlowski et al., 2016; Chen et al., 2020). However, analyses such as the ones used in this study, which leverage variations rather than absolute values, should not be sensitive to extraction artifacts if those artifacts bias all samples similarly (Allen and Kirchner, 2022).
3.5 Influences of vertical versus lateral flows along the hillslope
It is still unknown when or where lateral-flow processes affect soil water, which is typically conceptualized and measured from a vertically oriented perspective. To expand beyond this vertical perspective, we compared mobile and bulk soil waters from the two different sites at the top of the hillslope (the data shown in the previous figures) with those at a downslope stream-side site (sampled for approximately 1 year from 4 April 2022 onwards). We found that the isotopic signatures in mobile soil waters were similar between the two upslope sites but significantly different compared to the downslope site (t test, p value < 0.05 – Fig. 6). However, the bulk-soil-water signatures were not significantly different between the three sites (t test, p value > 0.05 – Fig. 6).
We also compared the isotopic signatures in all 11 boreholes, of which 5 are located on top of the hillslope (upslope); 4 are in the middle of the hillslope (mid-slope); and 2 are in the saturated zone, 1.5 and 5 m from the creek (downslope). We found that seasonal isotopic variability was small for all three hillslope positions and much smaller than the seasonal variability in streamflow (Fig. 7).
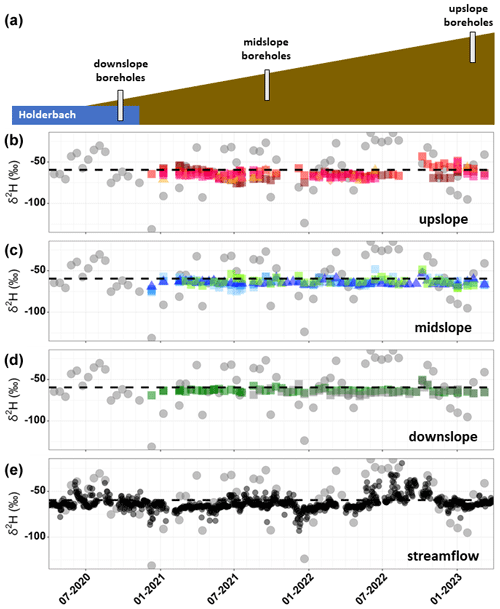
Figure 7Schematic diagram of the hillslope and borehole locations (a). Differences in the isotopic δ2H signatures of upslope and mid-slope deep mobile waters (b, c), downslope saturated groundwater (d), streamflow in the Holderbach creek (e), and precipitation (in gray in the background of the panels). The different colors indicate samples from different boreholes (five boreholes upslope, four boreholes mid-slope, and two boreholes downslope). The time series of the δ18O isotope signatures can be found in Fig. S3.
Water samples extracted from the soils and sediments that were excavated when digging the boreholes were examined together with the overlying shallower bulk soil waters to extend the depth profile of the stored subsurface waters. In general, all bulk soil waters were isotopically lighter than average precipitation (the dashed line in Fig. 8), indicating that they are dominated by lighter winter precipitation. With increasing depth, winter precipitation became even more dominant in the bulk soil and sediment waters, indicating that less and less summer precipitation reaches deep layers of the subsurface at this site (Fig. 8). Although our site typically receives more precipitation during the summer half of the year (i.e., around 60 % of annual precipitation), a much larger fraction of summer precipitation is lost through evaporation from the canopies, the forest floor litter layer, and the upper soil layer (e.g., Gerrits and Savenije, 2011; Floriancic et al., 2023). As a rough calculation, considering the interception loss of 20 % of precipitation from the forest canopy, another 18 % loss of precipitation from litter interception loss (see Floriancic et al., 2023), roughly 18 % of precipitation quickly reaching streams (Fig. 2), and an unknown amount being withdrawn from shallow soils by trees, we suspect that less than one-third of summer precipitation could end up recharging deeper storage. However, in winter, when these interception losses and evaporative demands are smaller, more winter precipitation is likely to be available to reach those depths, explaining the progressive decrease in isotope ratios with depth. An exception to this pattern is seen in Fig. 7, where the heavy-isotope signature of summer precipitation momentarily appears in the borehole records in October 2022, showing that these deep soil waters can respond to less-damped influxes, albeit with much more lag than seen in streamflow.
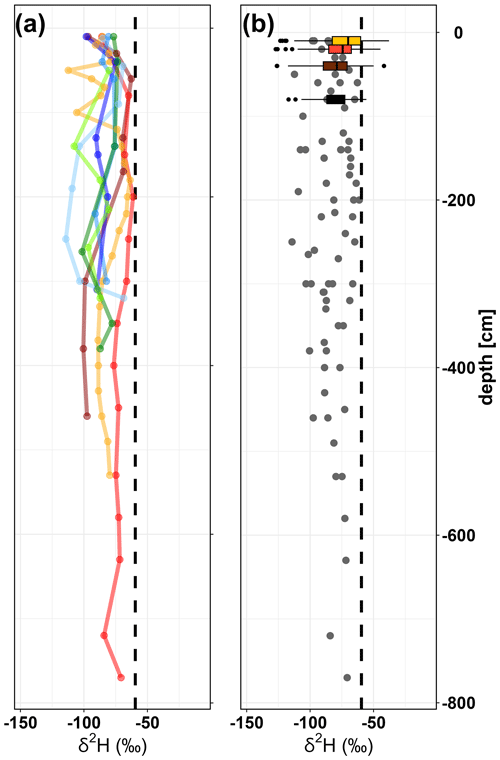
Figure 8Isotopic δ2H signals in bulk soil waters during borehole drilling on 22 November 2020 down to ∼ 7 m depth (a), also plotted in gray with boxplots of bulk-soil-water δ2H isotopic signatures for all regular bulk-soil-water samples across the 3-year observation period for 10 cm in yellow, 20 cm in red, 40 cm in brown, and 80 cm in black (b). The dashed line indicates the mean precipitation δ2H isotopic signature. Isotopic signatures in bulk-soil-water samples are typically lighter than the mean precipitation isotopic signatures, indicating a dominance of winter precipitation in bulk soil waters. The corresponding plots for δ18O can be found in Fig. S4.
3.6 Conceptualization of lateral and vertical hillslope water fluxes
Through interpreting Figs. 6 to 8 together, we identify two interesting trends. Bulk-soil-water signatures and variabilities were similar among the different sites, both in terms of value and pattern of progressive damping, indicating that similar vertical infiltration processes occur from 10 to 80 cm depth at each site. The mobile soil waters show some differences among sites, especially at the surface, shrinking at 40 to 80 cm and then disappearing at deeper depths (as seen in the borehole mobile waters). Thus, similar vertical-transport processes predominate in the top 80 cm of both the upslope and downslope positions, with minor differences likely being attributable to site-specific soil traits. However, somewhere below 80 cm, there is a transition to deeper soil waters being very well mixed (Fig. 7). From the data, we cannot tell whether this results from vertical mixing or homogenization due to lateral transport. However, any lateral transport or return flow from these deep, well-mixed pools has no observable effect on the soils at 10 to 80 cm depth, even those located at topographically lower downslope sites.
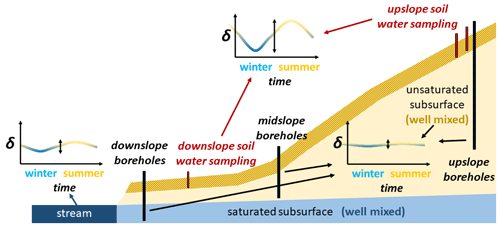
Figure 9Conceptualization of lateral and vertical hillslope water fluxes at the WaldLab Forest Experimental Site. The seasonal signal in soil water isotopes becomes weaker with depth. Below approximately 80 cm depth, soil waters are well mixed with almost no seasonality. The seasonal isotopic cycle is larger in streamflow than in mobile waters sampled from boreholes between 2 and 6 m depth, including boreholes that sample the saturated subsurface close to the stream. This suggests that streamflow at our site is a mixture of waters from the shallow (20–80 cm) subsurface, which exhibit a pronounced seasonal isotopic cycle, and from deeper layers, which exhibit almost no seasonal isotopic cycle.
Moreover, streamflow is much more isotopically variable than the deep-mobile-water samples, indicating that it is not primarily generated by displacement of these deep mobile waters into the stream channel (Hewlett and Hibbert, 1967; McDonnell, 1990). Even the boreholes in the saturated zone close to the creek (Fig. 7d) do not show the same seasonal variation that is observed in streamflow. Likewise, contributions from stream water do not control riparian groundwater signatures because the near-stream boreholes have vertical profiles similar to the upslope ones. The limited connection between near-stream groundwater and stream water is further indicated by the mismatch between shallower (10 to 80 cm) and deeper mobile waters (2 to 6 m) during wet periods, as would be expected if riparian-area groundwater levels rose to generate streamflow. Instead, the seasonal isotopic cycle in streamflow indicates that streamflow is formed from a mixture of deep subsurface waters, which exhibit nearly no seasonal isotopic cycle, and shallow (20–80 cm) soil waters, which exhibit a much more pronounced seasonal cycle (Fig. 9). These shallow soil waters must primarily originate close to the stream because we see no isotopic evidence for lateral dispersion or mixing of shallow soil waters across our hillslope sampling sites.
Interpreting mixtures of recent precipitation and older waters in the subsurface is key to understanding how, and how quickly, water is transported to its eventual fates as evapotranspiration and streamflow. By determining fractions of older waters versus recent precipitation in mobile soil water, bulk soil water, borehole mobile waters, and streamflow from continuous 3-year records of isotope data across our forested hillslope transect, we have generated a novel suite of insights into hillslope water movement.
Our isotopic analyses demonstrate that fractions of young and new waters decreased with depth below the surface but not monotonically. Roughly two-thirds of mobile soil waters at 10 to 40 cm depths were younger than 2 to 3 months, but less than 50 % of bulk soil waters were similarly young at depths of 20 to 80 cm. Thus, the most recent precipitation bypassed the smaller pores (represented by the bulk-soil-water samples) in the shallow layers. This isotopic evidence challenges general conceptualizations of new precipitation inputs wetting dry soils or displacing previously stored waters from those soils. At our site, this was only evident for the top 10 cm, which was also, unsurprisingly, strongly affected by evaporation. Stream water was composed of 18 % precipitation younger than 2–3 months, 11 % precipitation younger than 3 weeks, and 7 % precipitation younger than 1 week. These fractions of recent precipitation greatly exceeded those in deep subsurface waters at 2 to 6 m depth, even in boreholes situated immediately adjacent to the stream. The seasonal isotopic cycle in streamflow can only be explained as a mixture of deep subsurface waters, with very little isotopic seasonality, and shallow soil waters, with more pronounced isotopic seasonality. The bulk soil waters and deeper mobile soil waters were dominated by light isotopic signatures reflecting winter precipitation across both upslope and near-stream positions. Typically, fractions of recent precipitation in stream water and soil water were higher under wet antecedent conditions, indicating accelerated transport through the hillslope hydrologic system.
These observations illustrate how measurements of isotopic variability across different subsurface depths, hillslope positions, and timescales can help to constrain potential flow processes delivering precipitation to deep soils and streams.
The data that support the findings of this study are available from the corresponding author upon reasonable request.
The supplement related to this article is available online at: https://doi.org/10.5194/hess-28-4295-2024-supplement.
MGF designed and carried out the experiments and field sampling, processed the data, and prepared the paper with contributions from all the co-authors.
The contact author has declared that none of the authors has any competing interests.
Publisher's note: Copernicus Publications remains neutral with regard to jurisdictional claims made in the text, published maps, institutional affiliations, or any other geographical representation in this paper. While Copernicus Publications makes every effort to include appropriate place names, the final responsibility lies with the authors.
We thank Waldlabor Zürich (Martin Brüllhardt – https://www.waldlabor.ch, last access: 16 September 2024) and the forest owner Jakob Heusser for their cooperation and support. Further information on the WaldLab Forest Experimental Site can be found here: https://hyd.ifu.ethz.ch/ecohydlab/waldlab.html (last access: 16 September 2024). We acknowledge support during the data collection and laboratory work from the technical staff and student helpers working at the WaldLab Forest Experimental Site (Lena Straumann, Anna Lena Könz, Maria Grundmann, Lara Virsik, Elisa Hage, Stefano Martinetti, Fabian Strittmatter, Adrian Kreiner, Mark Bühler, Gian-Luca Cavelti, Lucien Biolley, and Martin Huber). We thank Tracy Napitupulu and Björn Studer for the isotope analyses and Annika Ackermann, Roland A. Werner, and Nina Buchmann for sharing the cryogenic extraction laboratory.
This research has been supported by the Waldlabor Zurich (grant no. 4.20.P01).
This paper was edited by Markus Weiler and reviewed by two anonymous referees.
Allen, S. T. and Kirchner, J. W.: Potential effects of cryogenic extraction biases on plant water source partitioning inferred from xylem-water isotope ratios, Hydrol. Process., 36, e14483, https://doi.org/10.1002/hyp.14483, 2022.
Allen, S. T., von Freyberg, J., Weiler, M., Goldsmith, G. R., and Kirchner, J. W.: The Seasonal Origins of Streamwater in Switzerland, Geophys. Res. Lett., 46, 10425–10434, https://doi.org/10.1029/2019GL084552, 2019.
Barbecot, F., Guillon, S., Pili, E., Larocque, M., Gibert-Brunet, E., Hélie, J.-F., Noret, A., Plain, C., Schneider, V., Mattei, A., and Meyzonnat, G.: Using Water Stable Isotopes in the Unsaturated Zone to Quantify Recharge in Two Contrasted Infiltration Regimes, Vadose Zone J., 17, 1–13, https://doi.org/10.2136/vzj2017.09.0170, 2018.
Berghuijs, W. R. and Kirchner, J. W.: The relationship between contrasting ages of groundwater and streamflow: Connecting Storage and Streamflow Ages, Geophys. Res. Lett., 44, 8925–8935, https://doi.org/10.1002/2017GL074962, 2017.
Bernhard, F., Floriancic, M. G., Treydte, K., Gessler, A., Kirchner, J. W., and Meusburger, K.: Tree- and stand-scale variability of xylem water stable isotope signatures in mature beech, oak and spruce, Ecohydrology, 17, e2614, https://doi.org/10.1002/eco.2614, 2024.
Beven, K. and Germann, P.: Macropores and water flow in soils, Water Resour. Res., 18, 1311–1325, https://doi.org/10.1029/WR018i005p01311, 1982.
Brooks, R. J., Barnard, H. R., Coulombe, R., and McDonnell, J. J.: Ecohydrologic separation of water between trees and streams in a Mediterranean climate, Nat. Geosci., 3, 100–104, https://doi.org/10.1038/ngeo722, 2010.
Burt, E. I., Coayla Rimachi, D. H., Ccahuana Quispe, A. J., Atwood, A., and West, A. J.: Isotope-derived young water fractions in streamflow across the tropical Andes mountains and Amazon floodplain, Hydrol. Earth Syst. Sci., 27, 2883–2898, https://doi.org/10.5194/hess-27-2883-2023, 2023.
Ceperley, N., Zuecco, G., Beria, H., Carturan, L., Michelon, A., Penna, D., Larsen, J., and Schaefli, B.: Seasonal snow cover decreases young water fractions in high Alpine catchments, Hydrol. Process., 34, 4794–4813, https://doi.org/10.1002/hyp.13937, 2020.
Chen, Y., Helliker, B. R., Tang, X., Li, F., Zhou, Y., and Song, X.: Stem water cryogenic extraction biases estimation in deuterium isotope composition of plant source water, P. Natl. Acad. Sci. USA, 117, 33345–33350, https://doi.org/10.1073/pnas.2014422117, 2020.
Floriancic, M. G., Allen, S. T., Meier, R., Truniger, L., Kirchner, J. W., and Molnar, P.: Potential for significant precipitation cycling by forest-floor litter and deadwood, Ecohydrology, 16, e2493, https://doi.org/10.1002/eco.2493, 2023.
Floriancic, M. G., Allen, S. T., and Kirchner, J. W.: Isotopic evidence for seasonal water sources in tree xylem and forest soils, Ecohydrology, 17, e2641, https://doi.org/10.1002/eco.2641, 2024a.
Floriancic, M. G., Stockinger, M. P., Kirchner, J. W., and Stumpp, C.: Monthly new water fractions and their relationships with climate and catchment properties across Alpine rivers, Hydrol. Earth Syst. Sci., 28, 3675–3694, https://doi.org/10.5194/hess-28-3675-2024, 2024b.
Gallart, F., Valiente, M., Llorens, P., Cayuela, C., Sprenger, M., and Latron, J.: Investigating young water fractions in a small Mediterranean mountain catchment: Both precipitation forcing and sampling frequency matter, Hydrol. Process., 34, 3618–3634, https://doi.org/10.1002/hyp.13806, 2020.
Gentile, A., Canone, D., Ceperley, N., Gisolo, D., Previati, M., Zuecco, G., Schaefli, B., and Ferraris, S.: Towards a conceptualization of the hydrological processes behind changes of young water fraction with elevation: a focus on mountainous alpine catchments, Hydrol. Earth Syst. Sci., 27, 2301–2323, https://doi.org/10.5194/hess-27-2301-2023, 2023.
Geris, J., Tetzlaff, D., McDonnell, J., Anderson, J., Paton, G., and Soulsby, C.: Ecohydrological separation in wet, low energy northern environments? A preliminary assessment using different soil water extraction techniques, Hydrol. Process., 29, 5139–5152, https://doi.org/10.1002/hyp.10603, 2015.
Gerrits, A. M. J. and Savenije, H. H. G.: Forest Floor Interception, in: Forest Hydrology and Biogeochemistry: Synthesis of Past Research and Future Directions, edited by: Levia, D. F., Carlyle-Moses, D., and Tanaka, T., Springer Netherlands, Dordrecht, 445–454, https://doi.org/10.1007/978-94-007-1363-5_22, 2011.
Goldsmith, G. R., Muñoz-Villers, L. E., Holwerda, F., McDonnell, J. J., Asbjornsen, H., and Dawson, T. E.: Stable isotopes reveal linkages among ecohydrological processes in a seasonally dry tropical montane cloud forest, Ecohydrology, 5, 779–790, https://doi.org/10.1002/eco.268, 2012.
Harper, W. V.: Reduced Major Axis Regression, in: Wiley StatsRef: Statistics Reference Online, edited by: Balakrishnan, N., Colton, T., Everitt, B., Piegorsch, W., Ruggeri, F., and Teugels, J. L., Wiley, 6 pp., https://doi.org/10.1002/9781118445112.stat07912, 2016.
Hervé-Fernández, P., Oyarzún, C., Brumbt, C., Huygens, D., Bodé, S., Verhoest, N. E. C., and Boeckx, P.: Assessing the `two water worlds' hypothesis and water sources for native and exotic evergreen species in south-central Chile, Hydrol. Process., 30, 4227–4241, https://doi.org/10.1002/hyp.10984, 2016.
Hewlett, J. D. and Hibbert, A. R.: Factors Affecting the Response of Small Watersheds to Precipitation in Humid Areas, in: Proceedings of the International Symposium on Forest Hydrology, Pergamon, edited by: Sopper, W. E. and Lull, H. W., Pennsylvania State University, New York, 275–290, 496013670, 1967.
Jasechko, S.: Global Isotope Hydrogeology – Review, Rev. Geophys., 57, 835–965, https://doi.org/10.1029/2018RG000627, 2019.
Jasechko, S., Birks, S. J., Gleeson, T., Wada, Y., Fawcett, P. J., Sharp, Z. D., McDonnell, J. J., and Welker, J. M.: The pronounced seasonality of global groundwater recharge, Water Resour. Res., 50, 8845–8867, https://doi.org/10.1002/2014WR015809, 2014.
Jasechko, S., Kirchner, J. W., Welker, J. M., and McDonnell, J. J.: Substantial proportion of global streamflow less than three months old, Nat. Geosci., 9, 126, https://doi.org/10.1038/ngeo2636, 2016.
Jasechko, S., Perrone, D., Befus, K. M., Bayani Cardenas, M., Ferguson, G., Gleeson, T., Luijendijk, E., McDonnell, J. J., Taylor, R. G., Wada, Y., and Kirchner, J. W.: Global aquifers dominated by fossil groundwaters but wells vulnerable to modern contamination, Nat. Geosci., 10, 425–429, https://doi.org/10.1038/ngeo2943, 2017.
Kirchner, J. W.: A double paradox in catchment hydrology and geochemistry, Hydrol. Process., 17, 871–874, https://doi.org/10.1002/hyp.5108, 2003.
Kirchner, J. W.: Aggregation in environmental systems – Part 1: Seasonal tracer cycles quantify young water fractions, but not mean transit times, in spatially heterogeneous catchments, Hydrol. Earth Syst. Sci., 20, 279–297, https://doi.org/10.5194/hess-20-279-2016, 2016a.
Kirchner, J. W.: Aggregation in environmental systems – Part 2: Catchment mean transit times and young water fractions under hydrologic nonstationarity, Hydrol. Earth Syst. Sci., 20, 299–328, https://doi.org/10.5194/hess-20-299-2016, 2016b.
Kirchner, J. W.: Quantifying new water fractions and transit time distributions using ensemble hydrograph separation: theory and benchmark tests, Hydrol. Earth Syst. Sci., 23, 303–349, https://doi.org/10.5194/hess-23-303-2019, 2019.
Kirchner, J. W. and Knapp, J. L. A.: Technical note: Calculation scripts for ensemble hydrograph separation, Hydrol. Earth Syst. Sci., 24, 5539–5558, https://doi.org/10.5194/hess-24-5539-2020, 2020.
Kirchner, J. W., Benettin, P., and van Meerveld, I.: Instructive Surprises in the Hydrological Functioning of Landscapes, Annu. Rev. Earth Pl. Sc., 51, 277–299, https://doi.org/10.1146/annurev-earth-071822-100356, 2023.
Knapp, J. L. A., Neal, C., Schlumpf, A., Neal, M., and Kirchner, J. W.: New water fractions and transit time distributions at Plynlimon, Wales, estimated from stable water isotopes in precipitation and streamflow, Hydrol. Earth Syst. Sci., 23, 4367–4388, https://doi.org/10.5194/hess-23-4367-2019, 2019.
Martinetti, S., Molnar, P., Carminati, A., and Floriancic, M. G.: Contrasting the soil-plant hydraulics of beech and spruce by linking root water uptake to transpiration dynamics, Tree Physiol., in review, 2024.
McDonnell, J. J.: A Rationale for Old Water Discharge Through Macropores in a Steep, Humid Catchment, Water Resour. Res., 26, 2821–2832, https://doi.org/10.1029/WR026i011p02821, 1990.
Neal, C. and Rosier, P. T. W.: Chemical studies of chloride and stable oxygen isotopes in two conifer afforested and moorland sites in the British uplands, J. Hydrol., 115, 269–283, https://doi.org/10.1016/0022-1694(90)90209-G, 1990.
Orlowski, N., Breuer, L., and McDonnell, J. J.: Critical issues with cryogenic extraction of soil water for stable isotope analysis, Ecohydrology, 9, 1–5, https://doi.org/10.1002/eco.1722, 2016.
Sprenger, M. and Allen, S. T.: What Ecohydrologic Separation Is and Where We Can Go With It, Water Resour. Res., 56, e2020WR027238, https://doi.org/10.1029/2020WR027238, 2020.
Sprenger, M., Leistert, H., Gimbel, K., and Weiler, M.: Illuminating hydrological processes at the soil-vegetation-atmosphere interface with water stable isotopes, Rev. Geophys., 54, 674–704, https://doi.org/10.1002/2015RG000515, 2016.
Sprenger, M., Llorens, P., Cayuela, C., Gallart, F., and Latron, J.: Mechanisms of consistently disjunct soil water pools over (pore) space and time, Hydrol. Earth Syst. Sci., 23, 2751–2762, https://doi.org/10.5194/hess-23-2751-2019, 2019a.
Sprenger, M., Stumpp, C., Weiler, M., Aeschbach, W., Allen, S. T., Benettin, P., Dubbert, M., Hartmann, A., Hrachowitz, M., Kirchner, J. W., McDonnell, J. J., Orlowski, N., Penna, D., Pfahl, S., Rinderer, M., Rodriguez, N., Schmidt, M., and Werner, C.: The Demographics of Water: A Review of Water Ages in the Critical Zone, Rev. Geophys., 57, 800–834, https://doi.org/10.1029/2018RG000633, 2019b.
Sun, Q., Klaus, V. H., Wittwer, R., Liu, Y., van der Heijden, M. G. A., Gilgen, A. K., and Buchmann, N.: Water uptake patterns of pea and barley responded to drought but not to cropping systems, Biogeosciences, 19, 1853–1869, https://doi.org/10.5194/bg-19-1853-2022, 2022.
von Freyberg, J., Studer, B., and Kirchner, J. W.: A lab in the field: high-frequency analysis of water quality and stable isotopes in stream water and precipitation, Hydrol. Earth Syst. Sci., 21, 1721–1739, https://doi.org/10.5194/hess-21-1721-2017, 2017.
von Freyberg, J., Allen, S. T., Seeger, S., Weiler, M., and Kirchner, J. W.: Sensitivity of young water fractions to hydro-climatic forcing and landscape properties across 22 Swiss catchments, Hydrol. Earth Syst. Sci., 22, 3841–3861, https://doi.org/10.5194/hess-22-3841-2018, 2018.
von Freyberg, J., Knapp, J. L. A., Rücker, A., Studer, B., and Kirchner, J. W.: Technical note: Evaluation of a low-cost evaporation protection method for portable water samplers, Hydrol. Earth Syst. Sci., 24, 5821–5834, https://doi.org/10.5194/hess-24-5821-2020, 2020.
Waring, R. H. and Running, S. W.: Forest Ecosystems, in: Analysis at Multiple Scales, 3rd edn., Elsevier, ISBN 9780123706058, 2007.
Weihermüller, L., Kasteel, R., Vanderborght, J., Pütz, T., and Vereecken, H.: Soil Water Extraction with a Suction Cup: Results of Numerical Simulations, Vadose Zone J., 4, 899–907, https://doi.org/10.2136/vzj2004.0156, 2005.