the Creative Commons Attribution 4.0 License.
the Creative Commons Attribution 4.0 License.
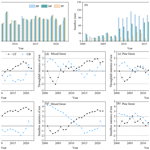
Rainfall redistribution in subtropical Chinese forests changes over 22 years
Wanjun Zhang
Thomas Scholten
Steffen Seitz
Qianmei Zhang
Guowei Chu
Linhua Wang
Xin Xiong
Juxiu Liu
Rainfall redistribution through the vegetation canopy plays a key role in the hydrological cycle. Although there have been studies on the heterogeneous patterns of rainfall redistribution in some ecosystems, the understanding of this process in different stages of forest succession remains insufficient. Therefore, this study investigated the change tendency in rainfall redistribution and rainwater chemistry in a subtropical forest succession in South China, based on 22 years (2001–2022) of rainfall monitoring (740 valid events).
Results showed that, at the event scale, both the throughfall ratio and the stemflow ratio in pine forest (PF) were higher than in mixed forest (MF) and broadleaf forest (BF). At the interannual scale, throughfall and stemflow of forests experienced an initial decrease followed by a subsequent increase over the entire measurement period (except stemflow of the pine forest), which reflects the trend in open rainfall. The variability in throughfall showed an increase from MF to PF to BF, and the variability in stemflow likewise showed an increase from MF to PF to BF. Changes in throughfall and stemflow in the BF are thus higher than those in the MF and PF over time. Furthermore, important differences in rainwater chemistry fluxes among the three forest types were found, changing in varying order over time. On average, total nitrogen (TN) and total phosphorus (TP) fluxes of throughfall increased from BF to MF to PF, while the potassium (K+) flux of throughfall showed a decrease from BF to MF to PF. Stemflow chemical fluxes varied less among forest types and over time, although tree species most importantly affected varying stemflow chemistry.
These results show important changes in patterns of rainfall redistribution over time and that characteristic variations are driven by rainfall and forest factors. Therefore, this study provides insight into long-term rainfall redistribution processes by linking changes in rainfall spectra with a typical subtropical forest succession sequence.
- Article
(1587 KB) - Full-text XML
-
Supplement
(650 KB) - BibTeX
- EndNote
In recent years, there has been ongoing concern about the potential impacts of climate change on forest ecosystems, particularly regarding rainfall input to water resources (Reynaert et al., 2021; Grossiord et al., 2017; Bruijnzeel et al., 2011; Leuzinger and Körner, 2010). Numerous studies have documented rainfall regimes and their effect on the water cycle in different regions of the world, including spatial and temporal changes in the amount, intensity, and frequency (Brasil et al., 2018; Ponette-González et al., 2010). Meanwhile, these variables in rainfall refer to the redistribution of rainfall into canopy interception, throughfall, and stemflow, which are important components of hydrological processes in terrestrial ecosystems (Germer et al., 2010; Levia and Frost, 2006; Loustau et al., 1992). Rainfall redistribution patterns can impact biogeochemical cycles down to soil moisture distribution, which in turn affects the activity of soil microorganisms that decompose organic matter (Tonello et al., 2021a; Junior et al., 2017; Van Stan and Pypker, 2015). For example, Sun et al. (2023) showed that throughfall reduction significantly affects the soil carbon cycle in a subtropical forest. Therefore, understanding the roles of rainfall redistribution in woodlands is essential.
Rainfall redistribution, as the partitioning into interception loss, throughfall, and stemflow, is an important hydrological process that regulates water and nutrient cycling in forest ecosystems. Interception loss refers to the part of the event rainfall intercepted by the canopy, accounting for about 10 %–30 % of gross rainfall depending on the studied forest canopy, for example shrub (Zhang et al., 2015), mixed broadleaf (Yan et al., 2003), or pine (Loustau et al., 1992). The remaining rainwater reaches the ground as either throughfall or stemflow. Throughfall is a critical component of rainfall redistribution, and it contributes on average to approximately 60 %–90 % of the gross rainfall on the forest floor in forests, shrubland, or cropland. (Zhang et al., 2023, 2021; Brauman et al., 2010; Marin et al., 2000). Raindrops coalesce or splash on canopy leaf surfaces, generating spatially different throughfall volume and raindrop kinetic energies which can be higher or lower than that of open rainfall (Levia et al., 2019; Goebes et al., 2015). Stemflow, the water flowing downwards along the plant stem or trunk, often accounts for only a small proportion (0 %–12 %) of rainfall (Niu et al., 2023; Yue et al., 2021; Llorens and Domingo, 2007). Nevertheless, stemflow inputs can be important as hot spots for near-trunk soils, inducing water and nutrient enrichment and deep infiltration, but also erosion (Zhao et al., 2023; Llorens et al., 2022). It can funnel more water than open rainfall over an equivalent area and contributes to 10 % of the annual soil water input (Levia and Germer, 2015; Chang and Matzner, 2000). Throughfall and stemflow restrict water input to the soil layer, thereby affecting soil moisture conditions, runoff generation, and water and nutrient cycling (Lian et al., 2022; Lacombe et al., 2018; Klos et al., 2014).
The proportions of rainfall redistribution are generally driven by meteorological conditions (e.g. rainfall amount, intensity, and duration) and vegetation cover (e.g. canopy structure and tree characteristics) (Tonello et al., 2021a; Sun et al., 2018; Mużyło et al., 2012; Nanko et al., 2006). For meteorological conditions, numerous studies have documented that the throughfall volume and stemflow volume increase with increasing gross rainfall amount and intensity (Ji et al., 2022; André et al., 2011). The ratios of throughfall and stemflow were both characterized as logarithmically increasing with rainfall, tending to be quasi-constant for heavy-rainfall events (Zhang et al., 2021; Liu et al., 2019). This is also synchronously related to the gradual saturation of the canopy, which limits the ratios of rainwater partitioning (Carlyle-Moses et al., 2004). Furthermore, spatial differences in water volume exist from place to place. The spatial variability (expressed as a coefficient of variation) in the throughfall volume is generally higher for low-rainfall events (<10 mm) than for heavy-rainfall events (Germer et al., 2006; Price et al., 1997).
Rainfall redistribution among different plant species can vary significantly due to differences in the structure and characteristics of their canopies. Specifically, key factors determining the redistribution of rainfall, such as the leaf area index (LAI), leaf shapes, and orientations, can affect the amount of intercepted loss and throughfall (Zhang et al., 2021; Goebes et al., 2015; Keim et al., 2006). The diameter at breast height (DBH), bark type, and orientation of trunks/stems and branches influence the amount of stemflow (Levia and Germer, 2015; Livesley et al., 2014; Germer et al., 2010). For each of the rainfall partitioning fluxes, their responses to the influential predictors often show high variation. A modelling study of rainfall partitioning in China explained that throughfall was best represented by mean tilt angle (MTA), followed by DBH. Subsequently, DBH was the dominant predictor for stemflow, followed by LAI and bark texture (Zhang et al., 2023). Due to these factors, rainfall redistribution presented different degrees of spatial variability. This variability (expressed as a coefficient of variation) decreased with increasing rainfall amount and intensity, consequently tending to be quasi-constant (Germer et al., 2006). Moreover, at an interannual scale, the ratios of rainfall redistribution are driven by annual canopy structures. The study of Niu et al. (2023) documented that the annual throughfall ratio gradually increased, while the annual stemflow ratio and interception loss ratio decreased with increasing thinning intensity in shrub plantations. Meanwhile, annual changes in rainfall events (amount and intensity) reinforced the time instability of throughfall spatial variability (Rodrigues et al., 2022). Overall, the rainfall–canopy interactions play a key role in rainfall redistribution processes and further affect the water cycle in many ecosystems.
The vegetation canopy is the functional interface between an ecosystem and atmospheric wet deposition (Van Stan and Pypker, 2015). Leaves and trunks/stems, acting as a filter, alter rainwater chemical concentrations via leaching and deposition processes. As a result, throughfall and stemflow exhibit high chemical concentrations compared with open rainfall (Jiang et al., 2021; Zimmermann et al., 2007). For instance, in a Chinese pine plantation, the volume-weighted mean concentrations of and in throughfall were significantly higher than those in open rainfall (Wang et al., 2023). Stemflow ion fluxes (e.g. K+) from deciduous tree species were higher than those for evergreen tree species because of the differences in bark morphology and branch architecture (Su et al., 2019). Moreover, it is also common that throughfall and stemflow chemistry shows fluctuating seasonality with the shifts in rainfall regime and leaf growth (Turpault et al., 2021; Siegert and Levia, 2014; Staelens et al., 2007). Plants mainly require N, P, K, Ca, and Mg. In general, N, K, and Ca are the most important inputs to a forest ecosystem, whereas P is the least. Phosphorus (P) is considered to be a limiting nutrient element in tropical and subtropical forests. The long-term productivity of vegetation depends on the input of atmospheric P. Furthermore, an increasing trend in atmospheric nitrogen (N) deposition with more frequent seasonal droughts in subtropical areas of China has been reported (Zhou et al., 2011), and this may inhibit the growth of plants and affect the productivity and functioning of forest ecosystems (Wu et al., 2023; Borghetti et al., 2017). Therefore, the significance of atmospheric precipitation to an ecosystem can also be confirmed for element cycling, and canopy leaching plays an important role in the chemical cycle of forest ecosystems.
Although there have been studies on the spatiotemporal variability in rainfall redistribution, most of these are limited to data from short-term monitoring over several months to 1–2 years (Liu et al., 2019; Ziegler et al., 2009; Carlyle-Moses et al., 2004; Marin et al., 2000). There are few studies exceeding several years of measurements and simultaneously focusing on forest structural changes and rainwater interception (Grunicke et al., 2020; Shinohara et al., 2015; Jackson, 2000). Long-term field monitoring studies are valuable to gain insight into the temporal dynamics of forest hydrological processes (Rodrigues et al., 2022; Sun et al., 2023; Levia and Frost, 2006). Such studies can also contribute to identifying patterns and trends in rainfall redistribution, which is essential for predicting the long-term effect of water resource change on forest ecosystems.
Therefore, in this study, we focus on the changing characteristics of throughfall and stemflow in a subtropical forest succession sequence (pine forest → mixed forest → monsoon evergreen broadleaf forest), based on long-term monitoring. Specifically, the objectives are to analyse (1) the changes in water volume of throughfall and stemflow and (2) the changes in water chemistry (N, P, and K+) of rainfall, throughfall, and stemflow among the three forests. We hypothesize that (1) both throughfall and stemflow in the broadleaf forest are characterized by high variability compared with mixed forest and pine forest and (2) the chemical fluxes of throughfall and stemflow change over time from broadleaf forest to mixed forest to pine forest. We aim to assess the variability in forest hydrological processes from a long-term perspective to help predict future dynamic trends in water resources in subtropical forest ecosystems.
2.1 Study site
This study was conducted at the Dinghushan Biosphere Reserve (– N, – E), located in Zhaoqing, South China. Dinghushan catchment consists of two streams, both with 12 km length, that flow into the Xi River (the main trunk of the Pearl River). According to the Köppen–Geiger climate classification (Kottek et al., 2006), the study area experiences a tropical monsoon climate (Cwa) with a pronounced wet (April–September) and dry (October–March) season. The average annual temperature is 20.9 °C, and the annual rainfall and evaporation are 1900 and 1115 mm, respectively. Dinghushan Biosphere Reserve is covered with a complete horizontal succession series of three types of subtropical forest, which is highly representative of the region (Zhou et al., 2011). The monsoon evergreen broadleaf forest (BF) is 400 years old and comprises typical tree species including Castanopsis chinensis (Spreng.) Hance, Schima superba Gardner and Champ., and Cryptocarya concinna Hance. The mixed pine–broadleaf forest (MF) is a natural 70- to 80-year-old succession with a coniferous broadleaf ratio of about 4:6. The main broadleaf tree species are Schima superba Gardner and Champ., Castanopsis chinensis (Spreng.) Hance, and the coniferous species Pinus massoniana Lamb. The pine forest (PF), planted before 1960, belongs to the primary succession community; within this community, Pinus massoniana Lamb. forms the only tree layer. The community composition and biodiversity are shown in Table S1 in the Supplement.
2.2 Gross rainfall, throughfall, and stemflow monitoring
Atmospheric rainfall data were collected at Dinghushan Automatic Meteorological Station from 2001 to 2022. Automatic meteorological systems were used to measure atmospheric pressure (DPA501 gas-pressure meter), temperature (HMP45D sensor), relative humidity (HMP45D sensor), and rainfall (SM1-1 pluviometer), and this information was recorded with a CR1000X datalogger (America Campbell). The resolution of the data recording was ±0.2 mm with a time interval of 10 min. The raw data comprised annual rainfall amounts as well as single rainfall events with throughfall and stemflow measurements.
Throughfall and stemflow were collected in all three forest types and synchronously measured. Devices with cross-shaped collectors (1.25 m2) attached to reservoirs (1000 L) at the bottom were used to collect throughfall. Three throughfall devices were randomly installed in each forest field (Fig. S1 in the Supplement).
Half-shell plastic tubes were installed around tree trunks attached to reservoirs (1000 L) at the bottom to collect stemflow. The ratio of volume (mL) to canopy area (cm2) is the stemflow (mm). A total of 24 trees were selected to measure the stemflow volume (Table S2 in the Supplement). In detail, four tree species were selected in the broadleaf forest, including Acmena acuminatissima (Blume) Merr. and L.M. Perry (SF1), Cryptocarya chinensis (Hance) Hemsl. (SF2), Gironniera subaequalis Planch. (SF3), and Schima superba Gardner and Champ. (SF4), with three repetitions, respectively. Three tree species were selected in the mixed forest, including Castanea henryi (Skam) Rehd. and Wils (SF5), Schima superba Gardner and Champ. (SF6), and Pinus massoniana Lamb. (SF7), with three repetitions, respectively. In the pine forest, Pinus massoniana Lamb. (SF8) was selected as the monitoring subject with three repetitions.
2.3 Rainwater chemistry measurement
For the measurement of rainwater chemistry, rainwater samples were taken in 2000, 2010, and 2022. The respective open rainfall, throughfall, and stemflow samples were manually collected for every 1-month period. The samples of open rainfall and throughfall were collected with three repetitions, whereas stemflow was collected with four repetitions in the broadleaf forest, three repetitions in the mixed forest, and three repetitions in the pine forest. In total, 792 rainwater samples (108 open rainfall, 324 throughfall, and 360 stemflow) were collected.
Rainwater samples were defrosted and filtered through 0.45 µm polypropylene membranes. Concentrations of total nitrogen (TN) and total phosphorus (TP) were measured using an ultraviolet spectrophotometer (Lambda 25, PerkinElmer), and ion potassium (K+) was measured using an inductively coupled plasma optical emission spectrometer (Optima 2000, PerkinElmer). The original TN, TP, and K+ data were processed into annual flux and monthly values with the weighted-average method:
where Ci and Vi are the concentrations of ions (mg L−1) and the water sample volume (L) in each rainfall event, respectively.
2.4 Other measurement and statistical analysis
In the forests, plant density and canopy structure have been measured every 5 years since 2000. In total, 25 plots of 20 m×20 m (A1–A25 plots) were built on an area of 1 ha to survey tree density (Fig. S1). Then, 25 plots of 5 m×5 (B1–B25 plots) were randomly set on the A1–A25 plots to survey shrub density. Finally, 25 plots of 1 m×1 m (C1–C25 plots) were randomly set on the B1–B25 plots to survey herb density. The percentage of the surface area covered by plants to the total plot area is termed canopy coverage (%). A total of 25 observation plots (1 m×1 m) were selected in the 1 ha area of each forest type. The LAI (leaf area index) was measured using an LAI-2200 plant canopy analyser with 90° view caps (LI-COR Inc., USA). A total of 10 observation points (distance of about 10 m) were selected in the 1 ha area of each forest type with five replications. The following growth indicators of the selected trees were recorded: tree height (m), diameter at breast height (DBH, cm), and crown area (CA, m2). Tree height was measured using a laser rangefinder. A tape measure was used to measure the diameter of trees at a height of 1.3 m, namely, the DBH. To establish the CA, a laser rangefinder was used to measure the maximum diameter at the edge of the canopy, with multiple measurements at different points to ensure accuracy.
The differences in throughfall and stemflow among different forests were assessed using an analysis of variance (ANOVA), followed by a Tukey test for multiple comparisons between means. A Mann–Kendall (MK) test was used to analyse the variation trend in annual rainfall. All statistical procedures were conducted with α=0.05 threshold for significance using the IBM SPSS Statistics 22.0 software (IBM Inc.).
3.1 Rainfall and temperature characteristics
Based on the 22-year rainfall dataset from the Dinghushan area, annual gross rainfall ranged between 1370.0 and 2361.1 mm (Fig. 1). A total of 78.0 % of gross rainfall appeared in the wet season (April–September). The M–K test showed that rainfall presented a significantly decreasing trend from 2001 to 2007 (UF<0, P<0.05, where UF is the unadjusted forward value); this then shifted to a significantly increasing pattern from 2012 to 2022 (UF>0, P<0.05). Moreover, 2008 and 2011 (the intersection of UF and UB, where UB is the unadjusted backward value) were the mutation time steps of the rainfall trend (P<0.05). Anomalies were revealed in the temporal variability (coefficient of variation, CV, of 16.6 %) in annual rainfall (Fig. 1a). Anomalies varied at −426.4 to 476.8 mm and −258.0 to 471.4 mm in the wet season and dry season, respectively. By comparison, the dry season experienced greater variation, with a CV of 40.4 %, than the wet season, with a CV of 21.7 %. Furthermore, annual rainy days tended to decrease over time from 2012 to 2021 (Fig. 1b). Based on five rainfall classifications, it was shown that rainfall <10 mm accounts for about 68.5 % of total rainy days (2856) among the 22 years, while rainfall >50 mm accounts for about 4.9 % of total rainy days. Moreover, annual temperature changed between 21.8 and 23.3 °C over the 22 years. The M–K test showed a statistically significant increase in temperature for 8 years out of the 22 years (UF>0, P<0.05). Moreover, 2005 and 2013–2014 were the mutation time steps in the temperature trend (P<0.05).
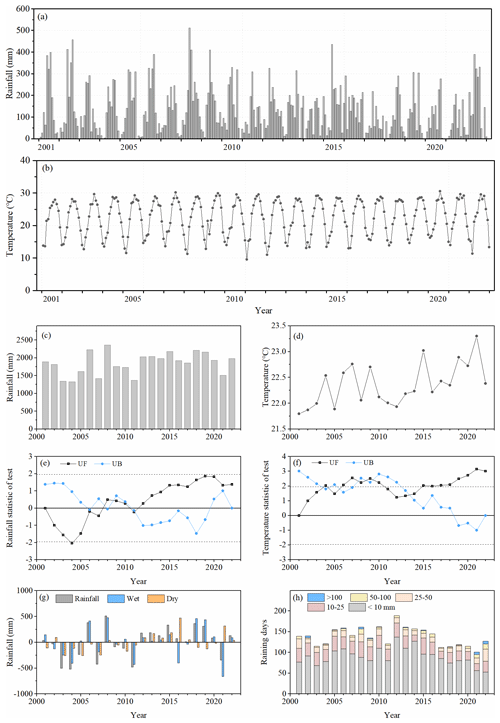
Figure 1Monthly (a) rainfall and (b) temperature in Dinghushan Biosphere Reserve in southern China from 2001 to 2022, annual (c) rainfall and (d) temperature in Dinghushan Biosphere Reserve in southern China from 2001 to 2022, and the (e) rainfall and (f) temperature statistics from the Mann–Kendall test. A UF (unadjusted forward) value > 0 indicates a continuous increasing trend (P<0.05). The intersection point of the UF and UB (unadjusted backward) values is the mutation time point. Within the confidence interval , the variable presents a significantly mutated growth state at this time point (P<0.05). Panel (g) presents the anomaly of annual rainfall from 2001 to 2022. Panel (h) shows the annual rainy days based on the five classifications listed in the legend.
3.2 Variability in throughfall
Rainfall redistribution (throughfall and stemflow) among the three forests experienced differing magnitudes for the 22 years (Fig. 2). Annual throughfall was concentrated between 954.2 and 2192.6 mm. The M–K test showed a significantly decreasing trend in throughfall at first (UF<0, P<0.05); this then shifted to a significant increase (UF>0, P<0.05) from 2001 to 2022, similar to the trend in open rainfall. In contrast, a mutation time step in throughfall occurred in 2008, 2011, and 2021 in the broadleaf forest; in 2008 and 2011 in the mixed forest; and in 2006, 2008, 2011, and 2021 in the pine forest (P<0.05). The throughfall ratio varied significantly at both the event and interannual scales (Fig. 3a–c). The median annual throughfall ratio in the broadleaf forest varied between 60 % and 120 % with a CV of 16.4 % from 2001 to 2022. The median throughfall ratio in the mixed pine–broadleaf forest varied between 80 % and 110 % with a CV of 9.7 %. The median throughfall ratio in the pine forest varied between 59 % and 110 % with a CV of 11.8 %. Therefore, the throughfall ratio was characterized by low variability at an annual timescale (CV<20 %). Moreover, some differences in the throughfall ratio were found among the three forest types based on rainfall classifications (Fig. 3d). For rainfall events <10 mm, the throughfall ratio range in the broadleaf forest was 30 %–70 %, whereas it was 15 %–85 % in the other two forest types. The mean value of the throughfall ratio was small in the broadleaf forest (53.9 %), although no significant differences among the three forests (P>0.05) were detected. For rainfall events <50 mm, no significant difference in the throughfall ratio among the three forest types was found (P>0.05). However, the median values of the throughfall ratio in the pine forest (90.0 %) and the mixed forest (89.4 %) were both significantly larger than that in the broadleaf forest (83.7 %) for rainfall events >50 mm.
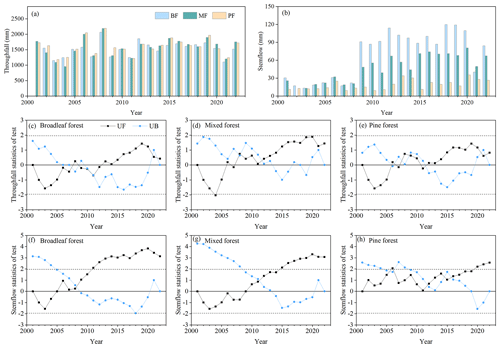
Figure 2Annual (a) throughfall and (b) stemflow in the respective broadleaf forest (BF), mixed pine–broadleaf forest (MF), and pine forest (PF) from 2001 to 2022 and (c–h) the respective rainfall and stemflow statistics from the Mann–Kendall test for each forest type. A UF (unadjusted forward) value > 0 indicates a continuous increasing trend (P<0.05). The intersection point of the UF and UB (unadjusted backward) values is the mutation time point. Within the confidence interval , the variable presents a significantly mutated growth state at this time point (P<0.05).
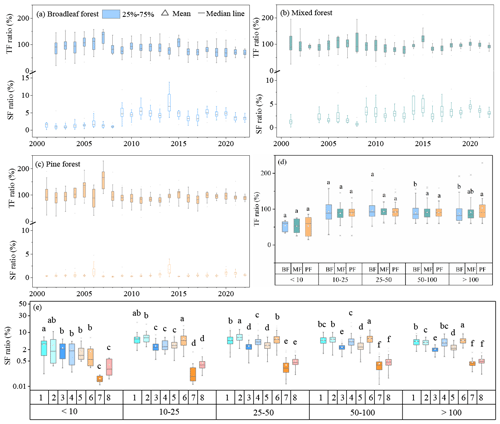
Figure 3Box plots of the throughfall (TF) ratio and stemflow (SF) ratio in (a) broadleaf forest, (b) mixed pine–broadleaf forest, and (c) pine forest from 2001 to 2022. Box plots of (d) the TF ratio in the three forests and (e) the SF ratio for eight plant species based on the rainfall classifications. Broadleaf forest comprised Acmena acuminatissima (Blume) Merr. and L.M. Perry (SF1), Cryptocarya chinensis (Hance) Hemsl. (SF2), Gironniera subaequalis Planch. (SF3), and Schima superba Gardner and Champ. (SF4). Mixed forest comprised Castanea henryi (Skam) Rehd. and Wils (SF5), Schima superba Gardner and Champ. (SF6), and Pinus massoniana Lamb. (SF7). Pine forest comprised Pinus massoniana Lamb. (SF8). Different letters indicate a significant difference at P<0.05.
The CV values of throughfall based on all of the rainfall event classifications are presented in Fig. 4a. Results show that the median CV of throughfall in the pine forest (15.2 %) is lower than that for the broadleaf forest (21.7 %) and for the mixed forest (26.3 %) for rainfall events <10 mm. For rainfall events >10 mm, small differences in the median CV among the three forest types are shown. Meanwhile, CV values decrease with increasing rainfall amounts, eventually falling to 3.5 %–4.3 %. Moreover, interannual-scale throughfall CV values are given in Fig. 5a, b, and c. Annual CV values among different forest types show different fluctuations over time. The annual and the wet- and dry-season median CV values presented different orders in different years. Based on a linear fit, significant negative correlations were found in the median of CVTF in the mixed forest over time (r=0.63, P<0.01). In addition, the fitted result of the 740 rainfall events over the 22 years showed that CV values of throughfall significantly decreased with increasing gross rainfall (Fig. S2 in the Supplement).
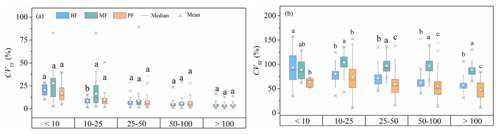
Figure 4Box plots of the coefficient of variation (CV, %) in (a) throughfall (TF) and (b) stemflow (SF) in broadleaf forest (BF), mixed pine–broadleaf forest (MF), and pine forest (PF) based on the rainfall classifications. Different letters indicate a significant difference at P<0.05.
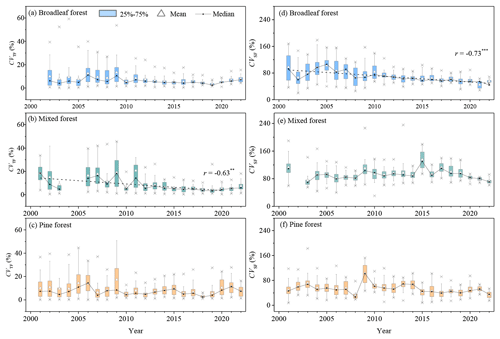
Figure 5Box plots of the coefficient of variation (CV, %) in (a–c) throughfall (TF) and (d–f) stemflow (SF) in the three forests from 2001 to 2022. Medians of the annual CV were fitted. r represents the Pearson coefficient of correlation, and ∗, , and denote significance at P<0.05, P<0.01, and P<0.001, respectively.
3.3 Variability in stemflow
Annual stemflow was concentrated between 9.0 and 119.7 mm over the 22 years (Fig. 2). More stemflow was collected in the broadleaf forest and mixed forest than in the pine forest. The M–K test showed that stemflow of both the broadleaf forest and mixed forest was decreasing at first (UF<0, P<0.05); this then shifted to an increase (UF>0, P<0.05), in contrast to the continuously increasing trend in the pine forest (UF>0). The mutation time steps of stemflow occurred in 2008 in the broadleaf forest; in 2011 in the mixed forest; and in 2006, 2012, and 2015 in the pine forest (P<0.05). The stemflow ratio changed significantly at both the event and interannual scales (Fig. 3a–c). For the 22 years, the median annual stemflow ratio in the broadleaf forest varied between 1.3 % and 5.4 % with a CV of 56.2 %. The stemflow ratio of mixed forest varied between 1.5 % and 4.4 % with a CV of 38.6 %. In the pine forest, it varied between 0.3 % and 1 % with a CV of 50.9 %. This indicated that the stemflow ratio was characterized by an extremely high variability over time. Similar to the seasonal throughfall ratio, the median annual and wet- and dry-season stemflow ratios presented different orders in different years. Furthermore, the stemflow ratio was significantly different among various tree species and rainfall classifications (Fig. 3e). By comparison, the stemflow ratios of the SF1 and SF2 trees in the broadleaf forest were both higher in all of the tree species for the rainfall events <50 mm. However, for strong events (>50 mm), the stemflow ratio of the SF5 tree in the mixed forest was highest for all tree species, followed by the trees in the broadleaf forest. For all the rainfall events, the stemflow ratios of SF7 in the mixed forest and SF8 in the pine forest were both lower than that for other tree species.
The CV values of stemflow based on rainfall event classifications are shown in Fig. 4b. By comparison, stemflow varied more than throughfall across all rainfall events, with CVSF values of 25 %–130 %. The median CV of stemflow in the pine forest was always lower (45 %–68 %) than that for the other two forest types (56 %–120 %). The CV values of stemflow on an interannual scale changed over time among different forest types (Fig. 5d–f). The annual and the wet- and dry-season medians of CVSF presented different orders in different years. By comparison, CVSF was always greater than CVTF, and the interannual fluctuation in CVSF was also stronger than that in CVTF. Based on a linear fit, significant negative correlations were found in the median of CVSF in the broadleaf forest over time (r=0.73). In addition, the fitted result of the 740 rainfall events over the 22 years showed that CV values of stemflow significantly decreased with increasing gross rainfall (Fig. S2).
3.4 Rainwater chemistry
Rainwater (open rainfall, throughfall, and stemflow) chemical properties (TN, TP, and K+ concentration) were measured in the three respective forest types. All of the TN, TP, and K+ values are presented in the following order: stemflow > throughfall > open rainfall (Fig. 6a–c). However, changes in TN, TP, and K+ were different for the three forest types in 2000, 2010, and 2022. For instance, in 2000 and 2010, TN values of throughfall and stemflow both decreased from pine forest to mixed forest to broadleaf forest, whereas no such result could be confirmed in 2022. Similarly, TP values of throughfall in the broadleaf forest were 1.3 times higher than in the pine forest in 2022, while TP values in the pine forest were 6.8 times higher than in the broadleaf forest in 2000. The K+ values of stemflow in 2010 (6.76 mg L−1) and 2022 (6.22 mg L−1) were higher for the broadleaf forest than for the pine forest (3.76 and 2.46 mg L−1, respectively), which was in contrast to our findings for 2022.
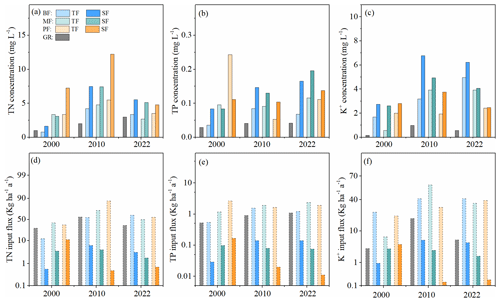
Figure 6Concentrations and fluxes of TN, TP, and K+ for gross rainfall (GR), throughfall (TF), and stemflow (SF) in the broadleaf forest (BF), mixed forest (MF), and pine forest (PF) in 2000, 2010, and 2022, respectively.
The TN, TP, and K+ fluxes of stemflow were <10, 0.2, 6 , respectively, and these values were all lower than those of throughfall and open rainfall (Fig. 7d–f). In 2000, 2010, and 2022, TN fluxes (39.4–87.4 ) were 1.2–1.8 times higher than those of open rainfall and 3.3–28.0 times higher than those of stemflow; TP fluxes (1.1–2.7 ) were 1.0–2.3 times higher those that of open rainfall and 8.7–31.4 times higher than those of stemflow; and K+ fluxes (21.5–59.2 ) were 2.2–8.1 times higher than those of open rainfall and 2.2–26.8 times greater than those of stemflow. In addition, TN, TP, and K+ fluxes of stemflow increased with succession from primary to climax, namely, pine forest < mixed forest < broadleaf forest. In contrast, differences in chemical fluxes of throughfall were not found among different forests or among different periods.
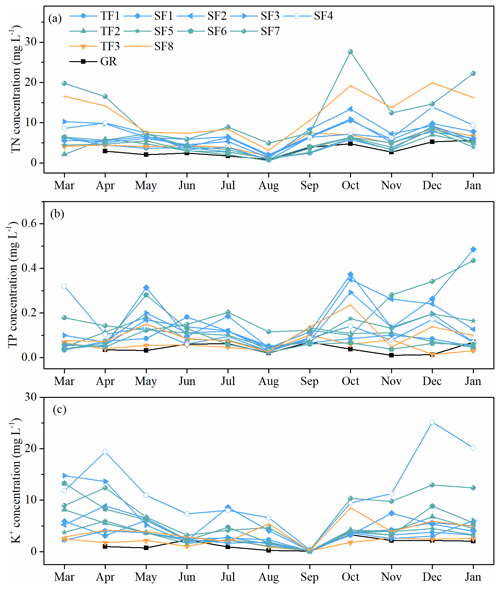
Figure 7Monthly concentrations of (a) TN, (b) TP, and (c) K+ in throughfall (TF1) and stemflow (SF1, SF2, SF3, and SF4) in the broadleaf forest, throughfall (TF2) and stemflow (SF5, SF6, and SF7) in the mixed forest, and throughfall (TF3) and stemflow (SF8) in the pine forest.
Moreover, monthly chemical concentrations in rainfall, throughfall, and stemflow showed distinct changes (Fig. 7). Monthly TN, TP, and K+ concentrations in rainfall were always lower than those of stemflow for all trees. Monthly TN, TP, and K+ in stemflow in the dry season were generally higher than in the wet season. High monthly TN concentrations of stemflow were found for SF6 in the mixed forest and for SF8 in the pine forest, especially in the dry season, with maximum TN concentrations of 27.59 mg L−1 for SF6 and 19.94 mg L−1 for SF8. In contrast, a high monthly K+ concentration was found in stemflow for SF4 in the broadleaf forest, with a maximum K+ concentration of 25.17 mg L−1 in the dry season.
4.1 Open rainfall partitioned to throughfall and stemflow
Studies in forests have confirmed that the throughfall volume increased with increasing gross rainfall at the event scale (Table 1), accounting for 60 %–80 % of gross rainfall (Ji et al., 2023; André et al., 2011; Carlyle-Moses, 2004). The throughfall ratio changed over time and showed different fluctuations among different forests (Fig. 3). During light-rainfall events with rainfall amounts <10 mm, a low proportion of raindrops would reach the ground as throughfall, as the tree canopy intercepts almost all of the incoming raindrops. Specifically, high canopy coverage in broadleaf forests can reinforce raindrop interception (Brasil et al., 2018; Ponette-González et al., 2010), consequently generating a lower throughfall ratio than those in the mixed forest and pine forest (Fig. 3). During moderate-rainfall events (10–50 mm), given that the interception effect of the wet tree canopy was weakened (Shinohara et al., 2015), the throughfall ratio was in a high and steady state. As the gross rainfall increased further (>50 mm), significant differences in the throughfall ratio were found among the three forests. The throughfall ratio was significantly lower in the broadleaf forest than those in the other two forests. Likewise, such differences due to the rainfall event class also appeared in other forest studies with stands formed by beech, pine monocultures, and mixed pine–beech (Blume et al., 2022). Influenced by forest stand characteristics, throughfall therefore indicated different forest water budgets.
Stemflow of forests was variably controlled by tree species, on average accounting for about <10 % of gross rainfall and occasionally <1 % (Sun et al., 2018; André et al., 2008; Crockford and Richardson, 1990). At our study site, the lowest stemflow (<1 %) was collected in the pine forest, although it weakly increased with rainfall classification (Fig. 3). The stemflow ratio in the broadleaf forest was maintained at 5 %–10 %, seemingly without an effect of rainfall amount. In detail, the stemflow ratio in the pine forest (SF8) was significantly lower than that in the broadleaf forest (SF1–4). In the mixed forest, broadleaf trees (SF5 and SF6) have larger stemflow than pine trees (SF7). However, for some rainfall events, a particularly low proportion of stemflow was caught in the broadleaf forest and an extraordinarily high proportion was caught in the pine forest. This implies that rainfall conditions (e.g. intensity and duration) and tree species with specific tree traits (e.g. branch angle) play a key role, consistent with reported studies, for example, the factor of tree size in evergreen forest (Chen et al., 2019; Bruijnzeel et al., 2011) or trunk lean and bark morphology in pine forest (Pinos et al., 2021; Crockford and Richardson, 1990). Moreover, an ANOVA showed significant differences in the stemflow ratio among tree species and rainfall classifications (P<0.001; Table 2). This indicates that rainfall and tree species simultaneously affect stemflow. The branch inclination angle, canopy cover, tree height, and DBH of tree species proved to be key factors affecting stemflow yield (Table 1; Levia et al., 2015).
Table 1Correlations of throughfall and stemflow with rainfall and forest factors.

The abbreviations used in the table are as follows: DBH – diameter at breast height; CA – crown area; and LAI – leaf area index. The significance levels presented are ∗ P<0.05, P<0.01, and P<0.001.
Table 2Analysis of variance (ANOVA) for throughfall and stemflow affected by rainfall classifications and tree species.

α=0.05.
Throughfall and stemflow were generally enriched with respect to their chemical concentration compared with open rainfall due to leachable canopy/stem ion pools (Jiang et al., 2021; Van Stan et al., 2017; Zimmermann et al., 2007). In our study, the concentration of K+ in stemflow was 16 times higher than that in open rainfall, while the K+ concentration in throughfall reached up to 11 times higher than that in open rainfall (Fig. 6). Similar results were also found in forestry plantations (Acacia mangium and Dimocarpus longan) in South China (Shen et al., 2013) and in oriental beech (Fagus orientalis Lipsky) trees in Northern Iran (Moslehi et al., 2019), indicating strong K+ leaching from the canopy. Even so, throughfall was generally characterized by high fluxes compared with open rainfall and stemflow; thus, it is the largest contributor to wet deposition. Meanwhile, the TN flux of throughfall was greatest in the pine forest in 2010, the TP flux of throughfall was greatest in the broadleaf forest in 2000, and the K+ flux of throughfall was greatest in the mixed forest in 2010. It should be noted that the differences in rainwater chemistry shifted over time among the three forests. Accordingly, throughfall and stemflow via the canopy and stem to the soil are significant contributors, and their long-term effect on ecosystems needs more scientific attention (Fan et al., 2021). Overall, atmospheric wet deposition provides important nutrient amounts for ecosystems, but it also imposes a considerable burden on the ecosystems in general. For instance, N enrichment and P limitation have proven to have different effects on soil carbon sequestration, microbial community composition, and forest productivity, especially in tropical and subtropical forest ecosystems with highly weathered soils (Zheng et al., 2022; Li et al., 2016; Huang et al., 2012). Moreover, throughfall and stemflow were mainly characterized by low chemical concentrations in the wet season and high concentrations in the dry season. The primary reasons for seasonal rainwater chemistry may be (i) the seasonal moisture source associated with frontal weather systems and (ii) the depleting effect of chemistry concentration with increasing rainfall amount (Dunkerley, 2014; Germer et al., 2007). The present study in subtropical forests and previous studies in tropical forests and European temperate forests all exhibited variable rainwater chemistry in throughfall and stemflow, both spatially and temporally (Zimmermann et al., 2007; Staelens et al., 2006; Seiler and Matzner, 1995). The chemical concentration of rainfall redistribution was also profoundly affected by the canopy and stem parameters of tree species (Tonello et al., 2021b; Chen et al., 2019). In our study, some differences in TN, TP, and K+ were also found among SF1–SF8 due to tree-species-specific effects (Legout et al., 2016; De Schrijver et al., 2007).
4.2 Long-term changes in rainfall in forests
Rainfall regimes induce spatially divergent hydrological changes (Wu et al., 2024). Likewise, our study found that the throughfall of forests experienced a decrease followed by an increase from 2001 to 2022, similar to the trend in open rainfall, and stemflow showed characteristic trends in different forests, especially in the pine forest (Fig. 1). This suggested that the complexity of the forest structure and rainfall amount and their change exacerbated the spatiotemporal variability in throughfall and stemflow. Firstly, interannual variability in the forest structure (e.g. canopy coverage and leaf area index) and tree parameters (e.g. height, DBH, and CA) made throughfall and stemflow distribution uncertain (Yue et al., 2021). From 2001 to 2022, changes in the forest structure were confirmed in all three forests, such as changes in the plant density, canopy coverage, and LAI (Fig. 8). The throughfall ratio and stemflow ratios in the succession forest systems varied accordingly over time. Similarly, driven by forest structure (e.g. tree density and species dominance), a 6-year dataset from the Brazilian Atlantic Forest showed that the spatial variability in throughfall over time was less stable (Rodrigues et al., 2022). Furthermore, the variation in stemflow (CVSF) was larger than that in throughfall (CVTF) (Fig. 4); this was probably due to the differences in tree species with respect to stemflow (Fig. 3). For a forest succession, a 17-year study showed that the shift from monoculture Japanese red pine to a mixture of red pine, evergreen oak, and deciduous trees significantly increased stemflow (Iida et al., 2005). Likewise, for the forest succession in the Dinghushan area, the stemflow ratios in the broadleaf forest and mixed forest were both higher than that in the monospecific pine forest. A high plant density (tree and shrub) and LAI in the broadleaf forest and mixed forest are conducive to rainwater interception by multi-canopy trees; moreover, more leaves and angled branches potentially enhance stemflow (Fig. 8). Indeed, some differences in rainfall redistribution have been found in multi-layered vegetative structures. An experiment on vegetation communities with complex multi-layered structures found that (1) interception loss from shrubs was 2 times higher than that from trees and (2) smaller trees generated stemflow more efficiently than taller ones (Exler and Moore, 2022). Based on the 22 years of data from the forest community survey in our study site, forest canopy parameters (e.g. coverage and LAI) of trees and shrubs showed variation over time from 2001 to 2022 (Fig. 8). In the broadleaf forest, the plant density of trees and the canopy coverage of shrubs showed a slight increment compared to the other two forest types, although the LAI was lower. During this period, the interannual throughfall ratio and stemflow ratio showed significant change over time (Fig. 3), implying that the interannual variation in the forest structure plays a role in the rainfall redistribution process.
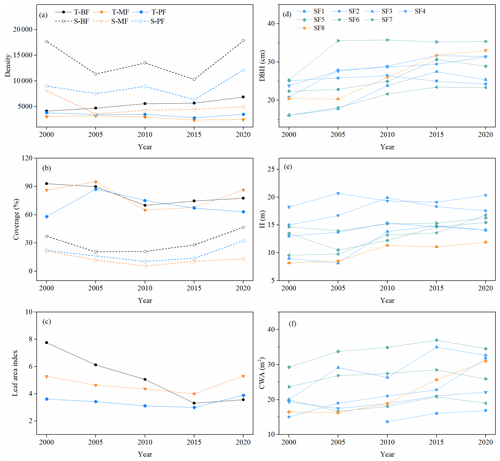
Figure 8The (a) plant density, (b) canopy coverage, and (c) leaf area index (LAI) of tree (T) and shrub (S) vegetation in the broadleaf forest (BF), mixed forest (MF), and pine forest (PF), respectively. The (d) diameter at breast height (DBH), (e) height (H), and (f) crown area (CA) are given for eight stemflow-sampled trees, respectively. Tree height was measured using a laser rangefinder. A tape measure was used to measure the diameter of trees at a height of 1.3 m, namely, the DBH. To establish the CA, a laser rangefinder was used to measure the maximum diameter at the edge of the canopy, with multiple measurements at different points to ensure accuracy. To survey the plant density, 25 plots of 20 m×20 m (A1–A25 plots) were built on a plot of 1 ha. Then, 25 plots of 5 m×5 m (B1–B25 plots) were randomly set on the A1–A25 plots to survey shrub density. Finally, 25 plots of 1 m×1 m (C1–C25 plots) were randomly set on the B1–B25 plots to survey herb density. To establish the canopy coverage, 25 observation plots (1 m×1 m) were selected in the 1 ha area of each forest type. The percentage of the surface area covered by plants with respect to the total plot area is termed canopy coverage (%). The LAI was measured using an LAI-2200 plant canopy analyser with 90° view caps (LI-COR Inc., USA). A total of 10 observation points (distance about 10 m) were selected in the 1 ha area of each forest type with five replications.
Secondly, ongoing rainfall changes with different magnitudes favour different levels of rainfall redistribution over time (Lian et al., 2022). At the event scale, the throughfall and stemflow proportions in the forests were both low for rainfall events <10 mm. The variations in throughfall and stemflow were both larger for gross rainfall <10 mm compared with that for events >10 mm. The rainfall threshold associated with the canopy interception capacity had an impact on throughfall and stemflow generation (Zabret et al., 2018; André et al., 2008; Durocher, 1990). Once canopy capacity reached its peak, throughfall and stemflow were documented to match the gross rainfall. Therefore, low proportions and high spatial variability in throughfall and stemflow appeared before the rainfall threshold; after the threshold was reached, relatively high proportions and low variability were observed in the three forests until a stable level. Moreover, at the interannual scale, the rainy days of different magnitudes presented fluctuations over the 22 years (Fig. 1). This fluctuation in rainy days and their distribution with respect to magnitude potentially regulated the long-term changes in open rainfall partitioned to interception loss, throughfall, and stemflow. Consequently, throughfall and stemflow, influenced by the comprehensive effect of rainfall regimes and forest structures, presented spatiotemporal variability at different levels (Figs. 3–6). From a long-term perspective, changes in rainfall redistribution potentially make forest water and biogeochemistry budgets more complex. Further knowledge of the long-term accumulative effect of rainfall redistribution on forest water and chemistry (e.g. soil and plant) is needed in the future.
Throughfall and stemflow are part of rainfall and are key players in the water cycle process. Based on the connection of the water cycle to precipitation and temperature and within the context of climate change, the frequency of extreme events (e.g. heavy rainfall and droughts) needs to be anticipated with respect to the associated effect on rainfall redistribution and solute transport within forests, which in turn may affect the water cycle and biogeochemical cycles (Blume et al., 2022). In this study, it should be noted that the 2008 rainfall data can be used as an example of an extreme event. In 2008, extreme weather events occurred in South China. Freezing events occurred in the dry season, whereas continuous heavy-rainfall and typhoon events occurred in the wet season. Gross rainfall was higher than in other years, with an annual rainfall of 2361.1 mm (22-year average annual rainfall of 1848.6 mm) (Fig. 1). At the same time, a total of 26 throughfall events were collected in 2008. The throughfall and stemflow trend in different forests presented different degrees of disturbance within the context of the mutation of open rainfall (Fig. 2). In this process, the driving effect of forest structure and rainfall on throughfall and stemflow mutation is synchronous. More data and modelling efforts are needed to support relevant work on the effect of climate change on rainfall redistribution in the future.
The current study investigated the long-term changes in the characteristics of rainfall redistribution along a subtropical forest succession sequence comprising pine forest (PF), mixed pine–broadleaf forest (MF), and monsoon evergreen broadleaf forest (BF). Firstly, in the 740 measured rainfall events, the throughfall ratio changed in the following order: BF < MF < PF. In contrast, the stemflow ratio changed in the following order: BF > MF > PF. The variation in stemflow was higher (CV>50 %) than that of throughfall (CV<25 %). Secondly, throughfall and stemflow of the investigated forests experienced a decrease followed by an increase from 2001 to 2022 (except for stemflow in the pine forest), similar to the trend in open rainfall. Driven by rainfall and forest factors, the interannual variability in both throughfall and stemflow in the broadleaf forest was higher than that in the mixed forest or pine forest, and this variability was different from annual open rainfall.
For rainwater chemistry, differences in the element fluxes in throughfall and stemflow among the three forest types were confirmed based on data from 2001, 2010, and 2022. On average, TN and TP fluxes of throughfall developed as follows: BF < MF < PF. In contrast, the K+ flux of throughfall changed as follows: BF > MF > PF. Over time, the rainwater chemical concentrations were lower in the wet season than in the dry season. Given the smaller proportion of open rainfall, stemflow chemical fluxes varied less among forest types and over time, although tree species in the forest types presented different stemflow chemistry (e.g. a high or low concentration). Nevertheless, the streamflow funnel effect on soil and plants over time still deserves more scientific attention in the future.
The above results indicate that (1) the water volume and chemistry of rainfall during redistribution processes under forests do not represent the same trend as open rainfall over time and (2) the throughfall and stemflow depend on the effect of rainfall and forest factors. Thus, this study provides insight into the rainfall redistribution process by linking the long-term change in rainfall patterns with a subtropical forest succession sequence.
The data supporting this study are freely available from Zenodo at https://doi.org/10.5281/zenodo.13329757 (Zhang, 2024).
The supplement related to this article is available online at: https://doi.org/10.5194/hess-28-3837-2024-supplement.
WZ: conceptualization, investigation, data analysis, writing, and visualization; TS and SS: review and supervision; QZ and GC: resources and data curation; LW: review; JL and XX: review, funding acquisition, and supervision.
The contact author has declared that none of the authors has any competing interests.
Publisher's note: Copernicus Publications remains neutral with regard to jurisdictional claims made in the text, published maps, institutional affiliations, or any other geographical representation in this paper. While Copernicus Publications makes every effort to include appropriate place names, the final responsibility lies with the authors.
Wanjun Zhang would like to acknowledge financial support from a CSC fellowship.
This research has been supported by the National Natural Science Foundation of China (grant nos. 42207158 and 32101342), the Special Project for Research and Development in Key areas of Guangdong Province (grant no. 2022B1111230001), and the China Postdoctoral Science Foundation (grant nos. 2021M703259, 2021M703260, and 2021M693220).
This paper was edited by Loes van Schaik and reviewed by John Van Stan and one anonymous referee.
André, F., Jonard, M., and Ponette, Q.: Influence of species and rain event characteristics on stemflow volume in a temperate mixed oak–beech stand, Hydrol. Process., 22, 4455–4466, https://doi.org/10.1002/hyp.7048, 2008.
André, F., Jonard, M., Jonard, F., and Ponette, Q.: Spatial and temporal patterns of throughfall volume in a deciduous mixed-species stand, J. Hydrol., 400, 244–254, https://doi.org/10.1016/j.jhydrol.2011.01.037, 2011.
Blume, T., Schneider, L., and Güntner, A.: Comparative analysis of throughfall observations in six different forest stands: Influence of seasons, rainfall-and stand characteristics, Hydrol. Process., 36, e14461, https://doi.org/10.1002/hyp.14461, 2022.
Borghetti, M., Gentilesca, T., Leonardi, S., Van Noije, T., and Rita, A.: Long-term temporal relationships between environmental conditions and xylem functional traits: a meta-analysis across a range of woody species along climatic and nitrogen deposition gradients, Tree Physiol., 37, 4–17, https://doi.org/10.1093/treephys/tpw087, 2017.
Brasil, J. B., Andrade, E. M. D., Palácio, H. A. D. Q., Medeiros, P. H. A., and Santos, J. C. N. D.: Characteristics of precipitation and the process of interception in a seasonally dry tropical forest, J. Hydrol.-Reg. Stud., 19, 307–317, https://doi.org/10.1016/j.ejrh.2018.10.006, 2018.
Brauman, K. A., Freyberg, D. L., and Daily, G. C.: Forest structure influences on rainfall partitioning and cloud interception: A comparison of native forest sites in Kona, Hawai'I, Agr. Forest Meteorol., 150, 265–275, https://doi.org/10.1016/j.agrformet.2009.11.011, 2010.
Bruijnzeel, L. A., Mulligan, M., and Scatena, F. N.: Hydrometeorology of tropical montane cloud forests: emerging patterns, Hydrol. Process., 25, 465–498, https://doi.org/10.1002/hyp.7974, 2011.
Carlyle-Moses, D. E., Laureano, J. F., and Price, A. G.: Throughfall and throughfall spatial variability in Madrean oak forest communities of northeastern Mexico, J. Hydrol., 297, 124–135, https://doi.org/10.1016/j.jhydrol.2004.04.007, 2004.
Chang, S. C. and Matzner, E.: The effect of beech stemflow on spatial patterns of soil solution chemistry and seepage fluxes in a mixed beech/oak stand, Hydrol. Process., 14, 135–144, https://doi.org/10.1002/(SICI)1099-1085(200001)14:1<135::AID-HYP915>3.0.CO;2-R, 2000.
Chen, S., Cao, R., Yoshitake, S., and Ohtsuka, T.: Stemflow hydrology and DOM flux in relation to tree size and rainfall event characteristics, Agr. Forest Meteorol., 279, 107753, https://doi.org/10.1016/j.agrformet.2019.107753, 2019.
Crockford, R. H. and Richardson, D. P.: Partitioning of rainfall in a eucalypt forest and pine plantation in southeastern Australia: II Stemflow and factors affecting stemflow in a dry sclerophyll eucalypt forest and a Pinus radiata plantation, Hydrol. Process., 4, 145–155, https://doi.org/10.1002/hyp.3360040205, 1990.
De Schrijver, A., Geudens, G., Augusto, L., Staelens, J., Mertens, J., Wuyts, K., Gielis, L., and Verheyen, K.: The effect of forest type on throughfall deposition and seepage flux: a review, Oecologia, 153, 663–674, https://doi.org/10.1007/s00442-007-0776-1, 2007.
Dunkerley, D.: Stemflow on the woody parts of plants: dependence on rainfall intensity and event profile from laboratory simulations, Hydrol. Process., 28, 5469–5482, https://doi.org/10.1002/hyp.10050, 2014.
Durocher, M. G.: Monitoring spatial variability of forest interception, Hydrol. Process., 4, 215–229, https://doi.org/10.1002/hyp.3360040303, 1990.
Exler, J. L. and Moore, R. D.: Quantifying throughfall, stemflow and interception loss in five vegetation communities in a maritime raised bog, Agr. Forest Meteorol., 327, 109202, https://doi.org/10.1016/j.agrformet.2022.109202, 2022.
Fan, Y. X., Lu, S. X., He, M., Yang, L. M., Hu, W. F., Yang, Z. J., Liu, X. F., Hui, D. F., Guo, J. F., and Yang, Y. S.: Long-term throughfall exclusion decreases soil organic phosphorus associated with reduced plant roots and soil microbial biomass in a subtropical forest, Geoderma, 404, 115309, https://doi.org/10.1016/j.geoderma.2021.115309, 2021.
Germer, S., Elsenbeer, H., and Moraes, J. M.: Throughfall and temporal trends of rainfall redistribution in an open tropical rainforest, south-western Amazonia (Rondônia, Brazil), Hydrol. Earth Syst. Sci., 10, 383–393, https://doi.org/10.5194/hess-10-383-2006, 2006.
Germer, S., Neill, C., Krusche, A. V., Neto, S. C. G., and Elsenbeer, H.: Seasonal and within-event dynamics of rainfall and throughfall chemistry in an open tropical rainforest in Rondônia, Brazil, Biogeochemistry, 86, 155–174, https://doi.org/10.1007/s10533-007-9152-9, 2007.
Germer, S., Werther, L., and Elsenbeer, H.: Have we underestimated stemflow? Lessons from an open tropical rainforest, J. Hydrol., 395, 169–179, https://doi.org/10.1016/j.jhydrol.2010.10.022, 2010.
Goebes, P., Bruelheide, H., Härdtle, W., Kröber, W., Kühn, P., Li, Y., Seitz, S., von Oheimb, G., and Scholten, T.: Species-specific effects on throughfall kinetic energy in subtropical forest plantations are related to leaf traits and tree architecture, PLoS one, 10, e0128084, https://doi.org/10.1371/journal.pone.0128084, 2015.
Grossiord, C., Sevanto, S., Dawson, T. E., Adams, H. D., Collins, A. D., Dickman, L. T., Newman B. D., Stockton, E. A., and McDowell, N. G.: Warming combined with more extreme precipitation regimes modifies the water sources used by trees, New Phytol., 213, 584–596, https://doi.org/10.1111/nph.14192, 2017.
Grunicke, S., Queck, R., and Bernhofer, C.: Long-term investigation of forest canopy rainfall interception for a spruce stand, Agr. Forest Meteorol., 292, 108125, https://doi.org/10.1016/j.agrformet.2020.108125, 2020.
Huang, W. J., Zhou, G. Y., and Liu, J. X.: Nitrogen and phosphorus status and their influence on aboveground production under increasing nitrogen deposition in three successional forests, Acta Oecol., 44, 20–27, https://doi.org/10.1016/j.actao.2011.06.005, 2012.
Iida, S. I., Tanaka, T., and Sugita, M.: Change of interception process due to the succession from Japanese red pine to evergreen oak, J. Hydrol., 315, 154–166, https://doi.org/10.1016/j.jhydrol.2005.03.024, 2005.
Jackson, N. A.: Measured and modelled rainfall interception loss from an agroforestry system in Kenya, Agr. Forest Meteorol., 100, 323–336, https://doi.org/10.1016/S0168-1923(99)00145-8, 2000.
Ji, S. Y., Omar, S. I., Zhang, S. B., Wang, T. F., Chen, C. F., and Zhang, W. J.: Comprehensive evaluation of throughfall erosion in the banana plantation, Earth Surf. Proc. Land., 47, 2941–2949, https://doi.org/10.1002/esp.5435, 2022.
Jiang, Z. Y., Zhi, Q. Y., Van Stan, J. T., Zhang, S. Y., Xiao, Y. H., Chen, X. Y., and Wu, H. W.: Rainfall partitioning and associated chemical alteration in three subtropical urban tree species, J. Hydrol., 603, 127109, https://doi.org/10.1016/j.jhydrol.2021.127109, 2021.
Junior, J. J., Mello, C. R., Owens, P. R., Mello, J. M., Curi, N., and Alves, G. J.: Time-stability of soil water content (SWC) in an Atlantic Forest-Latosol site, Geoderma, 288, 64–78, https://doi.org/10.1016/j.geoderma.2016.10.034, 2017.
Keim, R. F., Tromp-van Meerveld, H. J., and McDonnell, J. J.: A virtual experiment on the effects of evaporation and intensity smoothing by canopy interception on subsurface stormflow generation, J. Hydrol., 327, 352–364, https://doi.org/10.1016/j.jhydrol.2005.11.024, 2006.
Klos, P. Z., Chain-Guadarrama, A., Link, T. E., Finegan, B., Vierling, L. A., and Chazdon, R.: Throughfall heterogeneity in tropical forested landscapes as a focal mechanism for deep percolation, J. Hydrol., 519, 2180–2188, https://doi.org/10.1016/j.jhydrol.2014.10.004, 2014.
Kottek, M., Grieser, J., Beck, C., Rudolf, B., and Rubel, F.: World map of the Köppen-Geiger climate classification updated, Meteorol. Z., 15, 259–263, https://doi.org/10.1127/0941-2948/2006/0130, 2006.
Lacombe, G., Valentin, C., Sounyafong, P., De Rouw, A., Soulileuth, B., Silvera, N., Pierret, A., Sengtaheuanghoung, O., and Ribolzi, O.: Linking crop structure, throughfall, soil surface conditions, runoff and soil detachment: 10 land uses analyzed in Northern Laos, Sci. Total Environ., 616, 1330–1338, https://doi.org/10.1016/j.scitotenv.2017.10.185, 2018.
Legout, A., van Der Heijden, G., Jaffrain, J., Boudot, J. P., and Ranger, J.: Tree species effects on solution chemistry and major element fluxes: A case study in the Morvan (Breuil, France), Forest Ecol. Manag., 378, 244–258, https://doi.org/10.1016/j.foreco.2016.07.003, 2016.
Leuzinger, S. and Körner, C.: Rainfall distribution is the main driver of runoff under future CO2-concentration in a temperate deciduous forest, Global Change Biol., 16, 246–254, https://doi.org/10.1111/j.1365-2486.2009.01937.x, 2010.
Levia, D. F. and Germer, S.: A review of stemflow generation dynamics and stemflow-environment interactions in forests and shrublands, Rev. Geophys., 53, 673–714, https://doi.org/10.1002/2015RG000479, 2015.
Levia, D. F., Nanko, K., Amasaki, H., Giambelluca, T. W., Hotta, N., Iida, S. I., Mudd, R. G., Nullet, M. A., Sakai, N., Shinori, Y., Sun X. C., Suzuki, M., Tanaka, N., Tantasirin, C., and Yamada, K.: Throughfall partitioning by trees, Hydrol. Process., 33, 1698–1708, https://doi.org/10.1002/hyp.13432, 2019.
Levia Jr., D. F. and Frost, E. E.: Variability of throughfall volume and solute inputs in wooded ecosystems, Prog. Phys. Geog., 30, 605–632, https://doi.org/10.1177/0309133306071145, 2006.
Li, Y., Niu, S. L., and Yu, G. R.: Aggravated phosphorus limitation on biomass production under increasing nitrogen loading: a meta-analysis, Global Change Biol., 22, 934–943, https://doi.org/10.1111/gcb.13125, 2016.
Lian, X., Zhao, W. L., and Gentine, P.: Recent global decline in rainfall interception loss due to altered rainfall regimes. Nat. Commun., 13, 7642, https://doi.org/10.1038/s41467-022-35414-y, 2022.
Liu, J. Q., Liu, W. J., Li, W. X., and Zeng, H. H.: How does a rubber plantation affect the spatial variability and temporal stability of throughfall?, Hydrol. Res., 50, 60–74, https://doi.org/10.2166/nh.2018.028, 2019.
Livesley, S. J., Baudinette, B., and Glover, D.: Rainfall interception and stem flow by eucalypt street trees – The impacts of canopy density and bark type, Urban For. Urban Gree., 13, 192–197, https://doi.org/10.1016/j.ufug.2013.09.001, 2014.
Llorens, P. and Domingo, F.: Rainfall partitioning by vegetation under Mediterranean conditions. A review of studies in Europe, J. Hydrol., 335, 37–54, https://doi.org/10.1016/j.jhydrol.2006.10.032, 2007.
Llorens, P., Latron, J., Carlyle-Moses, D. E., Näthe, K., Chang, J. L., Nanko, K., Lida, S., and Levia, D. F.: Stemflow infiltration areas into forest soils around American beech (Fagus grandifolia Ehrh.) trees, Ecohydrology, 15, e2369, https://doi.org/10.1002/eco.2369, 2022.
Loustau, D., Berbigier, P., and Granier, A.: Interception loss, throughfall and stemflow in a maritime pine stand. II. An application of Gash's analytical model of interception, J. Hydrol., 138, 469–485, https://doi.org/10.1016/0022-1694(92)90131-E, 1992.
Marin, C. T., Bouten, W., and Sevink, J.: Gross rainfall and its partitioning into throughfall, stemflow and evaporation of intercepted water in four forest ecosystems in western Amazonia, J. Hydrol., 237, 40–57, https://doi.org/10.1016/S0022-1694(00)00301-2, 2000.
Moslehi, M., Habashi, H., Khormali, F., Ahmadi, A., Brunner, I., and Zimmermann, S.: Base cation dynamics in rainfall, throughfall, litterflow and soil solution under Oriental beech (Fagus orientalis Lipsky) trees in northern Iran, Ann. Forest Sci., 76, 1–12, https://doi.org/10.1007/s13595-019-0837-8, 2019.
Mużyło, A., Llorens, P., and Domingo, F.: Rainfall partitioning in a deciduous forest plot in leafed and leafless periods, Ecohydrology, 5, 759–767, https://doi.org/10.1002/eco.266, 2012.
Nanko, K., Hotta, N., and Suzuki, M.: Evaluating the influence of canopy species and meteorological factors on throughfall drop size distribution, J. Hydrol., 329, 422–431, https://doi.org/10.1016/j.jhydrol.2006.02.036, 2006.
Niu, X. T., Fan, J., Du, M. G., Dai, Z. J., Luo, R. H., Yuan, H. Y., and Zhang, S. G.: Changes of Rainfall Partitioning and canopy interception modeling after progressive thinning in two shrub plantations on the Chinese Loess Plateau, J. Hydrol., 619, 129299, https://doi.org/10.1016/j.jhydrol.2023.129299, 2023.
Pinos, J., Latron, J., Levia, D. F., and Llorens, P.: Drivers of the circumferential variation of stemflow inputs on the boles of Pinus sylvestris L. (Scots pine), Ecohydrology, 14, e2348, https://doi.org/10.1002/eco.2348, 2021.
Ponette-González, A. G., Weathers, K. C., and Curran, L. M.: Water inputs across a tropical montane landscape in Veracruz, Mexico: synergistic effects of land cover, rain and fog seasonality, and interannual precipitation variability, Global Change Biol., 16, 946–963, https://doi.org/10.1111/j.1365-2486.2009.01985.x, 2010.
Price, A. G., Dunham, K., Carleton, T., and Band, L.: Variability of water fluxes through the black spruce (Picea mariana) canopy and feather moss (Pleurozium schreberi) carpet in the boreal forest of Northern Manitoba, J. Hydrol., 196, 310–323, https://doi.org/10.1016/S0022-1694(96)03233-7, 1997.
Reynaert, S., De Boeck, H. J., Verbruggen, E., Verlinden, M., Flowers, N., and Nijs, I.: Risk of short-term biodiversity loss under more persistent precipitation regimes, Global Change Biol., 27, 1614–1626, https://doi.org/10.1111/gcb.15501, 2021.
Rodrigues, A. F., Terra, M. C., Mantovani, V. A., Cordeiro, N. G., Ribeiro, J. P., Guo, L., Nehren, U., Mello, M. J., and Mello, C. R.: Throughfall spatial variability in a neotropical forest: Have we correctly accounted for time stability?, J. Hydrol., 608, 127632, https://doi.org/10.1016/j.jhydrol.2022.127632, 2022.
Seiler, J. and Matzner, E.: Spatial variability of throughfall chemistry and selected soil properties as influenced by stem distance in a mature Norway spruce (Picea abies, Karst.) stand, Plant Soil, 176, 139–147, https://doi.org/10.1007/BF00017684, 1995.
Shen, W. J., Ren, H. L., Jenerette, G. D., Hui, D. F., and Ren, H.: Atmospheric deposition and canopy exchange of anions and cations in two plantation forests under acid rain influence, Atmos. Environ., 64, 242–250, https://doi.org/10.1016/j.atmosenv.2012.10.015, 2013.
Shinohara, Y., Levia, D. F., Komatsu, H., Nogata, M., and Otsuki, K.: Comparative modeling of the effects of intensive thinning on canopy interception loss in a Japanese cedar (Cryptomeria japonica D. Don) forest of western Japan, Agr. Forest Meteorol., 214–215, 148–156, https://doi.org/10.1016/j.agrformet.2015.08.257, 2015.
Siegert, C. M. and Levia, D. F.: Seasonal and meteorological effects on differential stemflow funneling ratios for two deciduous tree species, J. Hydrol., 519, 446–454, https://doi.org/10.1016/j.jhydrol.2014.07.038, 2014.
Staelens, J., De Schrijver, A., Verheyen, K., and Verhoest, N. E.: Spatial variability and temporal stability of throughfall deposition under beech (Fagus sylvatica L.) in relationship to canopy structure, Environ. Pollut., 142, 254–263, https://doi.org/10.1016/j.envpol.2005.10.002, 2006.
Staelens, J., De Schrijver, A., and Verheyen, K.: Seasonal variation in throughfall and stemflow chemistry beneath a European beech (Fagus sylvatica) tree in relation to canopy phenology, Can. J. Forest Res., 37, 1359–1372, https://doi.org/10.1139/X07-003, 2007.
Su, L., Zhao, C. M., Xu, W. T., and Xie, Z. Q.: Hydrochemical fluxes in bulk precipitation, throughfall, and stemflow in a mixed evergreen and deciduous broadleaved forest, Forests, 10, 507, https://doi.org/10.3390/f10060507, 2019.
Sun, J. M., Yu, X. X., Wang, H. N., Jia, G. D., Zhao, Y., Tu, Z. H., Deng, W. P., Jia, J. B., and Chen, J. G.: Effects of forest structure on hydrological processes in China, J. Hydrol., 561, 187–199, https://doi.org/10.1016/j.jhydrol.2018.04.003, 2018.
Sun, S. Y., Liu, X. F., Lu, S. X., Cao, P. L., Hui, D. F., Chen, J., Guo, J. F., and Yang, Y. S.: Depth-dependent response of particulate and mineral-associated organic carbon to long-term throughfall reduction in a subtropical natural forest, Catena, 223, 106904, https://doi.org/10.1016/j.catena.2022.106904, 2023.
Tonello, K. C., Rosa, A. G., Pereira, L. C., Matus, G. N., Guandique, M. E. G., and Navarrete, A. A.: Rainfall partitioning in the Cerrado and its influence on net rainfall nutrient fluxes, Agr. Forest Meteorol., 303, 108372, https://doi.org/10.1016/j.agrformet.2021.108372, 2021a.
Tonello, K. C., Van Stan II, J. T., Rosa, A. G., Balbinot, L., Pereira, L. C., and Bramorski, J.: Stemflow variability across tree stem and canopy traits in the Brazilian Cerrado, Agr. Forest Meteorol., 308, 108551, https://doi.org/10.1016/j.agrformet.2021.108551, 2021b.
Turpault, M. P., Kirchen, G., Calvaruso, C., Redon, P. O., and Dincher, M.: Exchanges of major elements in a deciduous forest canopy, Biogeochemistry, 152, 51–71, https://doi.org/10.1007/s10533-020-00732-0, 2021.
Van Stan II, J. T. and Pypker, T. G.: A review and evaluation of forest canopy epiphyte roles in the partitioning and chemical alteration of precipitation, Sci. Total Environ., 536, 813–824, https://doi.org/10.1016/j.scitotenv.2015.07.134, 2015.
Van Stan, J. T., Wagner, S., Guillemette, F., Whitetree, A., Lewis, J., Silva, L., and Stubbins, A.: Temporal dynamics in the concentration, flux, and optical properties of tree-derived dissolved organic matter in an epiphyte-laden oak-cedar forest, J. Geophys. Res.-Biogeo., 122, 2982–2997. https://doi.org/10.1002/2017JG004111, 2017.
Wang, C. Y., Sun, X. C., Fan, C. B., Wei, Y. X., Jia, G. K., and Cao, Y. H.: Spatio-temporal variability and intra-event variation of throughfall ammonium and nitrate in a pine plantation, Hydrol. Process., 37, e14872, https://doi.org/10.1002/hyp.14872, 2023.
Wu, T., Song, Y. T, Tissue, D., Su, W., Luo, H. Y, Li, X., Yang, S. M., Liu, X. J., Yan J. H., Huang, J., and Liu, J. X.: Photosynthetic and biochemical responses of four subtropical tree seedlings to reduced dry season and increased wet season precipitation and variable N deposition, Tree Physiol., 44, tpad114, https://doi.org/10.1093/treephys/tpad114, 2023.
Wu, Y. P., Yin, X. W., Zhou, G. Y., Bruijnzeel, L. A., Dai, A. G., Wang, F., Gentine, P., Zhang, G. C., Song, Y. N., and Zhou, D. C.: Rising rainfall intensity induces spatially divergent hydrological changes within a large river basin, Nat. Commun., 15, 823, https://doi.org/10.1038/s41467-023-44562-8, 2024.
Yan, J. H., Zhou, G. Y., Zhang, D. Q., and Wang, X.: Spatial and temporal variations of some hydrological factors in a climax forest ecosystem in the Dinghushan region, Acta Ecol. Sin., 23, 2359–2366, 2003.
Yue, K., De Frenne, P., Fornara, D. A., Van Meerbeek, K., Li, W., Peng, X., Ni, X. Y., Peng, Y., Wu, F. Z., Yang Y. S., and Peñuelas, J.: Global patterns and drivers of rainfall partitioning by trees and shrubs, Global Change Biol., 27, 3350–3357, https://doi.org/10.1111/gcb.15644, 2021.
Zabret, K., Rakovec, J., and Šraj, M.: Influence of meteorological variables on rainfall partitioning for deciduous and coniferous tree species in urban area, J. Hydrol., 558, 29–41, https://doi.org/10.1016/j.jhydrol.2018.01.025, 2018.
Zhang, W.: Experimental rainfall dataset in subtropical Chinese forests, Zenodo [data set], https://doi.org/10.5281/zenodo.13329757, 2024.
Zhang, W. J., Zhu, X. A., Chen, C. F., Zeng, H. H., Jiang, X. J., Wu, J. E., Zou, X., Yang, B., and Liu, W. J.: Large broad-leaved canopy of banana (Musa nana Lour.) induces dramatically high spatial–temporal variability of throughfall, Hydrol. Res., 52, 1223–1238, https://doi.org/10.2166/nh.2021.023, 2021.
Zhang, Y. F., Wang, X. P., Hu, R., Pan, Y. X., and Paradeloc, M.: Rainfall partitioning into throughfall, stemflow and interception loss by two xerophytic shrubs within a rain-fed re-vegetated desert ecosystem, northwestern China, J. Hydrol., 527, 1084–1095, https://doi.org/10.1016/j.jhydrol.2015.05.060, 2015.
Zhang, Y. F., Yuan, C., Chen, N., and Levia, D. F.: Rainfall partitioning by vegetation in China: A quantitative synthesis, J. Hydrol., 617, 128946, https://doi.org/10.1016/j.jhydrol.2022.128946, 2023.
Zhao, W. Y., Ji, X. B., Jin, B. W., Du, Z. Y., Zhang, J. L., Jiao, D. D., and Zhao, L. W.: Experimental partitioning of rainfall into throughfall, stemflow and interception loss by Haloxylon ammodendron, a dominant sand-stabilizing shrub in northwestern China, Sci. Total Environ., 858, 159928, https://doi.org/10.1016/j.scitotenv.2022.159928, 2023.
Zheng, M. H., Zhang, T., Luo, Y. Q., Liu, J. X., Lu, X. K., Ye, Q., Wang, S. H., Huang, J., Mao, Q. G., Mo, J. M., and Zhang, W.: Temporal patterns of soil carbon emission in tropical forests under long-term nitrogen deposition, Nat. Geosci., 15, 1002–1010, https://doi.org/10.1038/s41561-022-01080-4, 2022.
Zhou, G. Y., Wei, X. H., Wu, Y. P., Liu, S. G., Huang, Y. H., Yan, J. H., Zhang, D. Q., Zhang, Q. M., Liu, J. X., Meng, Z., Wang, C. L., Chu, G. W., Liu, S. Z., Tang, X. L., and Liu, X. D.: Quantifying the hydrological responses to climate change in an intact forested small watershed in Southern China, Global Change Biol., 17, 3736–3746, https://doi.org/10.1111/j.1365-2486.2011.02499.x, 2011.
Ziegler, A. D., Giambelluca, T. W., Nullet, M. A., Sutherland, R. A., Tantasarin, C., Vogler, J. B., and Negishi, J. N.: Throughfall in an evergreen-dominated forest stand in Northern Thailand: comparison of mobile and stationary methods, Agr. Forest Meteorol., 149, 373–384, https://doi.org/10.1016/j.agrformet.2008.09.002, 2009.
Zimmermann, A., Wilcke, W., and Elsenbeer, H.: Spatial and temporal patterns of throughfall quantity and quality in a tropical montane forest in Ecuador, J. Hydrol., 343, 80–96, https://doi.org/10.1016/j.jhydrol.2007.06.012, 2007.