the Creative Commons Attribution 4.0 License.
the Creative Commons Attribution 4.0 License.
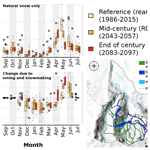
Simulated hydrological effects of grooming and snowmaking in a ski resort on the local water balance
Marion Réveillet
Eric Sauquet
Louise Crochemore
Flora Branger
Étienne Leblois
Marie Dumont
The presence of a ski resort modifies the snow cover at the local scale, due to snow management practices on ski pistes, especially grooming and snowmaking. Snow management exerts 2-fold effects on the local hydrological cycle, through (i) abstraction and transfer of water used for snowmaking, and (ii) changes in water runoff due to added snow mass through snowmaking and/or delayed melting of the snowpack due to snow grooming. This induces a local pressure on water resources, which has seldom been addressed in scientific studies hitherto.
Here we introduce a method to compute the hydrological effects of snow management on ski pistes and we apply and illustrate its results for the case study of the La Plagne ski resort in the Northern French Alps. The approach mainly relies on snow cover modelling using the Crocus snow cover driven by SAFRAN reanalysis and climate projections. Model results are evaluated against in-situ hydrological observations and show that the modelling approach, although very simplified for many hydrological processes, provides relevant information and insights in terms of the influence of snow-related processes on water resources.
Our study shows a visible impact of grooming, virtually eliminating snowmelt in winter, thus delaying the onset of snowmelt. This results is a lower snowmelt flux during the wintertime, low flow period, on the order of −10 % to −20 %, compensated by higher amounts when snow melts. While about 10 % of the water used for snowmaking is estimated to be lost by evaporation through the ice formation process from the liquid water droplets, we find that, in the case studied, the annual scale alteration of water resources is limited and estimated to be on the order of 1 % to 2 %. This is due to the fact that the amount of water used for snowmaking on ski pistes represents a fraction of 10 % to 20 % of total annual precipitation, that ski pistes cover typically 10 % of the surface area of catchments within which ski resorts are located, and that snowmaking equipment covers, in the case of La Plagne, 40 % of the surface area of ski pistes. Therefore, in this case, snowmaking mainly leads to a moderate shift in snow cover formation and snowmelt processes and plays, for example, a smaller role than the influence of future climate change on mountain hydrology.
This study provides an initial overview of the influence of grooming and snowmaking on river flows in a mountain catchment, which can inform future studies on water management and climate change adaptation in areas with ski tourism facilities. This study does not discuss long-term sustainability challenges of ski tourism and other aspects of the local environmental impacts (landscape, biodiversity) of snow management, such as the construction and use of mountain water reservoirs and other earthworks in ski resorts.
- Article
(9722 KB) - Full-text XML
- BibTeX
- EndNote
Ski resorts are a prominent component of mountain economies in many regions of the world (Europe, North America, China, Japan, New Zealand and South Eastern Australia) (Steiger et al., 2019; Hock et al., 2019; Vanat, 2021). Snow management, in particular grooming and snowmaking, i.e. artificial production of snow on ski pistes before and during the ski season, have become routine activities for ski resorts operations (Steiger et al., 2019). Among the various environmental concerns related to the operations of ski resorts, the hydrological impact of snowmaking is often mentioned as an argument against their development. In particular, the use of substantial amounts of water for snowmaking is considered as an unsustainable pressure exerted on the mountain environment. It is, however, often argued by ski tourism supporters that water is only “borrowed” for snowmaking and is returned to mountain creeks and rivers at the time of snowmelt. Despite this contentious situation, very few scientific studies have addressed the influence of snow management quantitatively, in particular snowmaking, on the water cycle at both local and regional scale. This is, however, required in order to contribute scientific information regarding the relationships between mountain water resources and ski resort operations, at a time of intense debates regarding the transition of mountain tourism into a more sustainable pathway, taking into account climate change impacts and related adaptation options (Hock et al., 2019; Tschanz et al., 2022; François et al., 2023). The goal of the present study is to explore this knowledge gap and to shed light on the influence of grooming and snowmaking on the local hydrological cycle, in particular the river runoff downstream of a watershed within which a ski resort is located.
Eisel et al. (1988, 1990) carried out pioneering studies on water losses due to snowmaking based on case studies in Colorado (United States of America), motivated primarily by water rights issues. They separated water losses into two categories: “initial loss”, corresponding to “consumptive water loss that occurs during the actual snowmaking process due to evaporation and sublimation” and “watershed loss”, corresponding to “consumptive water loss that occurs from the time the man-made snow or ice particles have fallen on the snowpack through spring melt. This loss is due to sublimation and evapotranspiration”. Eisel et al. (1988) focused on the “initial loss”, which was later complemented by more recent studies carried out in Europe (Spandre et al., 2017; Grünewald and Wolfsperger, 2019). The amount of “initial losses” are estimated to be on the order of 10 % of the water used for snowmaking, due to evaporation and atmospheric sublimation processes. Spandre et al. (2017) highlighted that some of the snow produced for snowmaking is not recovered on ski pistes (it simply falls besides the ski pistes), and is thus wasted from perspective of ski resort operations, although it is not lost in terms of the total amount of water in the catchment. They estimated that this concerns approximately 30 % of the water used for snowmaking, although it varies substantially depending on local context and meteorological conditions. Eisel et al. (1990) investigated the watershed losses for several ski resorts, by means of a comparison between observed runoff, water consumption for snowmaking and meteorological conditions, and simulated runoff. They concluded that, for the situations analysed, the watershed loss ranged between 7 % to 33 % of the water used for snowmaking, after the initial loss, leading to 13 % to 37 % total consumptive loss. This indicates that, in this case, abstracting water for snow production leads to substantial net water loss for the catchments due to sublimation and evaporation of the snow cover on ski pistes. However, they clearly stated that “these results should not be extrapolated directly to other specific ski area sites because actual consumptive loss at these sites is dependent on atmospheric temperature during snowmaking, temperature, and precipitation during the winter and watershed conditions at the site”. We are not aware of whether these studies were corroborated by further studies, or whether they were used operationally, in particular for water rights applications. In a more recent study, Wemple et al. (2007) reported on the fact that snowmaking corresponded to 3 % to 4 % of the total annual precipitation in a ski resort in the NE USA. Even more recently, Gerbaux et al. (2020) compared water demand for snowmaking with water resources availability in several ski resorts in the Northern French Alps, but did not address the downstream effects on the river runoff induced by snow grooming and snowmaking and potential water losses. Leroy (2015) developed an integrated modelling approach for the quantitative comparison of the amount of water withdrawals for various uses (hydropower, agriculture, domestic use, snowmaking) in a pilot ski resort in the Northern French Alps, but did not address downstream influence on river flows either.
The present study explores the impact of snow management (grooming and snowmaking) on the hydrological cycle and downstream water availability, in particular seasonal patterns, at the local scale. Mostly based on modelling tools, we implement this approach as a case study in the Northern French Alps (La Plagne ski resort). We provide and analyse results at various geographical scales: (i) at the point scale at 1800 m elevation, (ii) for a study domain encompassing the entire ski resort area, and (iii) for two interconnected catchments (Frasses and Bonnegarde catchments) for which simulation results and a diverse set of observations are combined and jointly analysed. Results are provided not only for past meteorological conditions but also under future climate conditions, for the Bonnegarde catchment.
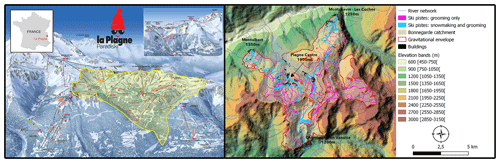
Figure 1Maps of the La Plagne ski resort, with the Bonnegarde catchment in yellow. On the left-hand side: location of the La Plagne ski resorts on a national map for France, landscape image provided by the La Plagne ski resort, overlay of the Bonnegarde catchment by the authors. On the right-hand side, detailed map of the ski resort, showing its gravitational envelope (solid red line), ski pistes with (blue) and without (pink) snowmaking, location of main villages. Right-hand side: Hillshaded background derived from EU-DEM v1.1 (Copernicus Land Monitoring Service), further geographical information from BD Alti provided by IGN at the 25 m resolution, geographical information on location and status of ski pistes provided by the La Plagne ski resort (generation of the map by the authors, see text for methodological information).
The La Plagne ski resort is the largest ski resort in France, one the of world largest ski resorts, with over 2 million daily skier visits every year (Vanat, 2021), located in the Northern French Alps. It spans an elevation range from 1250 to 3250 m above sea level and includes 5.28 km2 of ski pistes (Ebner et al., 2021). Figure 1 shows the geographical location of La Plagne and the geographical distribution of its pistes with and without snowmaking.
The main snowmaking technology used in La Plagne is lances. Lances are a type of snowmaking equipment that use pressurized water rather than a fan-like snowgun. French ski resorts are equipped to a great extent with such equipment. At the scale of the entire ski resort, 40.2 % of the surface area of ski pistes is equipped with snowmaking, which is higher than the average value for French ski resorts (Berard-Chenu et al., 2022b).
The wider ski resort area is defined by its gravitational envelope. This concept was introduced by François et al. (2014) and later refined and implemented by further studies (François et al., 2016, 2023; Spandre et al., 2019; Berard-Chenu et al., 2022a). Indeed, there is no standard delineation of the ski resort's geographical domain. The gravitational envelope corresponds to the geographical domain accessible downhill from the top of all the ski lifts and reaching the bottom of one of the ski lifts. Not all of the gravitational envelope consist of ski pistes (they cover, on average 10 % of the surface area according to Spandre et al., 2019) and is even accessible to skiing, but it provides a representative and usable boundary for the area characterizing the ski resort, based only on the catalogue of ski lifts and the local topography. In La Plagne, the surface area of ski pistes corresponds to 9.7 % of the surface area of the gravitational envelope, so that, ultimately, the gravitational envelope is covered at 3.9 % by ski pistes equipped with snowmaking. Figure 1 shows the La Plagne gravitational envelope used for this study.
Water used for snow production in La Plagne is stored in five reservoirs (Prajourdan, Lovatière, Forcle, Pierres Blanches and Montchavin; see Fig. 2) located in or near the ski resort. Water is taken from various sources (surface or underground) to fill these reservoirs. Some reservoirs are managed under a 2-fold purpose, i.e. meeting water demand for snow production and water supply for domestic uses.
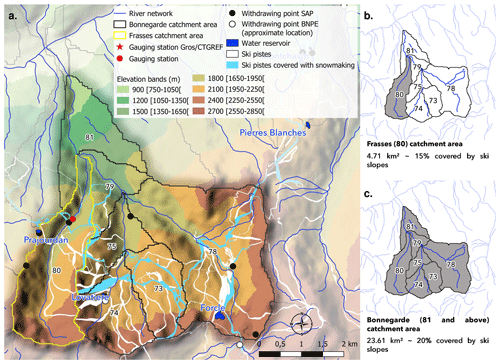
Figure 2Detailed map of the Bonnegarde catchment illustrating its various subcatchments, including the Frasses catchment. Hillshaded background derived from EU-DEM v1.1 (Copernicus Land Monitoring Service), further geographical information from BD Alti provided by IGN at the 25 m resolution, geographical information on location and status of ski pistes provided by the La Plagne ski resort (generation of the map by the authors, see text for methodological information).
Figure 2 shows the subcatchments including the ski pistes for the Bonnegarde catchment and one of its subcatchments (the Frasses catchment), which are analysed in more details in this study. These catchments are located at the heart of the La Plagne ski resort, including main villages and ski tourism hosting infrastructures. Figure 2 illustrates the complexity of the interaction between the spatial organization of the ski resort and the local hydrological context. The Frasses catchment has a surface area of 4.71 km2, and 15.2 % of its surface area is covered by ski pistes. The Bonnegarde catchment, which includes the Frasses catchment, has a surface area of 23.61 km2, and 19.4 % of its surface area is covered by ski pistes, 36.2 % of which is equipped with snowmaking equipment. Overall, 7.0 % of the Bonnegarde catchment is covered by snowmaking equipment. The outlet of the Bonnegarde catchment almost directly reaches the Isère river flowing through the Tarentaise valley.
3.1 Snow cover modelling
In this study, the simulation of the natural and managed snowpack is performed using the Crocus snow cover model, which represents natural snow processes, but also grooming and snowmaking (Spandre et al., 2016a, 2019). The model accounts for all the processes governing the internal evolution of the snowpack and its interfaces, including the surface mass balance: absorption and reflection of solar radiation, as a function of the surface albedo of the snow calculated by the model, absorption of atmospheric infrared radiation, emission of infrared thermal energy as a function of the surface temperature of the snow, latent and sensible heat fluxes at the surface of the snowpack, as a function of the surface temperature of the snow and atmospheric conditions (wind speed, temperature, relative humidity, etc.) (Vionnet et al., 2012). The implementation of snowmaking in the model (described in detail in Spandre et al., 2016a) is consistent with typical practices in ski resort operations (Spandre et al., 2016b), although local deviations (timing of snowmaking operations, configuration of the snowmaking units, etc.) can be observed (Abegg et al., 2020). We here use typical values rather than refining the model configuration in detail, so that the modelling system can be transferred to other contexts more easily. In the model, snow production is only possible for wind speed values below 4.2 m s−1 and for wet bulb temperature values (computed using temperature and relative humidity values, as described in Spandre et al., 2016b) below −6 ∘C for the lances' snowguns. The density of the snow produced is 600 kg m−3. The representation of the grooming process includes the compaction and the mixing of snow layers (effect of the tiller), so that the snowpack on ski pistes results from the gradual mixing of snow from natural snowfall and machine-made snow (Spandre et al., 2016b). Note that the modelling system does not account for the effect of skiers on the snowpack (compaction, snow displacement), and this is implicitly represented through the effect of grooming (Spandre et al., 2016b). In the early season, before the start of the main winter holiday period, production can take place between 1 November and 15 December, only if the wet bulb and wind speed conditions are met, until a maximum of 150 kg m−2 of water is converted into artificial snow, i.e. 25 cm of artificial snow at 600 kg m−3, taking into account 40 % water loss (combining evaporative losses (10 %) and the fact that not all of the machine-made snow falls on the ski pistes (30 %)). Between 15 December and 31 March the production can take place (again, if the wet bulb temperature and wind speed conditions allow) as soon as the snow depth falls below 60 cm. There is no further production after 31 March. The model calculates the water demand corresponding to snow production, without imposing limits to the availability of water used for snowmaking. For further details, see Spandre et al. (2016b, 2019).
For areas besides the ski pistes, only natural snow cover processes are considered. Note that we do not take into account interactions between forests and the snow cover in this study, because we focus on the differences between simulations with and without grooming and snowmaking on ski pistes. Also, note that the modelling framework used in this study does not take into account wind-induced snow transport. In the modelling system used, the snowpack interacts with the underlying soil, represented by the SURFEX/ISBA land surface model (Masson et al., 2013). Thermally, the underlying soil is coupled to the snowpack through a diffusion scheme. This makes it possible to represent the insulating effect of the snow, according to its physical properties, and the thermal interaction between the snow, the ground and the atmosphere. From a hydrological point of view, several water fluxes are calculated by the model (Fig. 3) and are available at daily time steps in the simulation results produced for this study. This study focuses on quantifying the impact of snow management on water availability in the watershed. The main water flux analysed and used is, therefore, the amount of water reaching the upper soil interface, which combines the water flux flowing at the base of the snowpack (due to snow melt and liquid water percolation through the snowpack) with the liquid precipitation (rain) on snow-free ground. This variable is referred to as the “total liquid water reaching soil” in Fig. 3.
3.2 Model simulations
In this work, consistent with many previous studies using snow cover modelling in French mountainous regions, the Crocus model is driven by meteorological conditions estimated by the SAFRAN reanalysis (temperature, specific humidity, precipitation, wind speed, incoming radiation) (Vernay et al., 2022). Consistent with the geometry of the SAFRAN modelling system leading to the SAFRAN meteorological reanalysis, the simulations – named hereafter SAFRAN-Crocus simulations – are performed for 300 m altitude bands within spatially homogeneous areas (massifs), on flat terrain and for slopes of 10, 20, 30, 40∘, for eight main aspects (N, NE, E, SE, S, SW, W, NW). We use SAFRAN data for the Vanoise massif, within which the La Plagne ski resort is located. The full duration of the SAFRAN-Crocus simulation used for this study spans from 1961 to 2020.
The use of SAFRAN reanalysis data feeding the Crocus model including snow grooming and snowmaking has been used as such for other studies (Spandre et al., 2019; Gerbaux et al., 2020; Morin et al., 2021; Ebner et al., 2021), which demonstrated its ability to reproduce observed snow conditions on ski pistes. In particular, Ebner et al. (2021) analysed the performance of SAFRAN-Crocus simulations in terms of snow conditions on ski pistes at the La Plagne ski resort.
3.3 Geographical representation of the ski resort and catchments
Our study uses the concept of ski resorts representative units (SRUs), introduced in Hanzer et al. (2020). This approach identifies unit elements characterizing the ski pistes (or their surrounding environment), taking into account their elevation and aspect and the presence or absence of snowmaking equipment. Here we use the topographical clustering of the SAFRAN reanalysis described above. A given SRU is either concerned with natural snow (outside ski pistes) or, on ski pistes, grooming only or grooming and snowmaking. Snow cover simulations are carried out for each SRU, and their results can be combined according to their surface area (Hanzer et al., 2020; Ebner et al., 2021) in order to provide integrated information such as the total amount of liquid water reaching soil for a given area (gravitational envelope, catchment, etc.).
Hydrological catchments located within or close to the ski resorts were derived from the high-resolution digital elevation model (DEM) BD-Alti from the French National Geographic Institute (IGN), using HydroDem, a set of computer routines for processing digital elevation models from a hydrological perspective. HydroDem was initially designed to support the scientific development of geostatistically based runoff mapping routines (e.g. Gottschalk et al., 2006). Over the years, HydroDem has been used as an aid for delineating drainage patterns in a variety of medium- and large-scale distributed hydrological model applications (e.g. Thierion et al., 2012, as in this study). HydroDem's computer code implements routines quite similar to a lot of other software. The drainage pattern is built as follows: local flow directions are first estimated from the direction of local maximum slope, using 3×3 kernels for a smooth estimation of partial derivatives; such procedures are known to generate a suitable drainage pattern from the slopes of the digital elevation model (DEM), but there are a number of local conflicts in the valleys, where watercourses are naturally not resolved by the raster grid cell of the DEM, and a heuristic algorithm is then used to remedy them, where the lowest elevation path and the most likely way out are identified in each case. This heuristic algorithm is applied gradually, first passes accepting only modest levels of both elevation increase and connection of pool areas. Another notable trick is that the accepted elevation increase is scaled by the standard deviation of local elevation, allowing the algorithm to self-adapt in the case of subareas of contrasting roughness.
3.4 Data used for the hydrological analysis of the Bonnegarde and Frasses catchments
In addition to the snow cover modelling approach described above, we perform an in-depth analysis of the components of the hydrological cycle of the Frasses and Bonnegarde catchments (see Fig. 2) based on a hydrological modelling approach and various observational data. In this area, information on hydrology is rather sparse:
-
River flow measurements were collected between June and mid-November at a single location (corresponding to a catchment surface area of 3.85 km2) by the Société d'Aménagement de la Plagne (SAP) along the Frasses River since 2013 (“Gauging station SAP” in Fig. 2; 4 km2).
-
A gauging station that is now closed (“Gauging station Gros/CTGREF” in Fig. 2; 4 km2) recorded discharges over two discontinuous periods (1948–1968 and in 1980). Only statistics derived from time series of daily discharge are currently available for this gauging station (average daily flows over the period 1948–1968 for Gros data and monthly flows for 1980 for CTGREF data). These discharge data were only corrected for upstream water diversion, i.e. 45 L s−1 each day from June to September (transfer out of the gauged catchment mainly in summer for irrigation purposes through an open channel).
In addition, we have considered time series of natural daily discharge simulated by the distributed hydrological model J2000 (Morel et al., 2023) over the period 1980–2016. The version of J2000 used here considers natural hydrological processes and was calibrated at the whole Rhone basin scale to simulate daily discharges at the outlet of medium-scale basins. The SAFRAN-France meteorological reanalysis (Vidal et al., 2010) was used as meteorological forcings for J2000. Note that, in contrast to the SAFRAN reanalysis used for running the Crocus snow cover model, which operates for mountain massifs and 300 m elevation bands, SAFRAN-France operates on a 8 km×8 km grid.
Water abstraction data were provided by the French national database BNPE (Banque Nationale des Prélèvements quantitatifs en Eau, https://bnpe.eaufrance.fr/, last access: 6 November 2023), including time series of annual withdrawals, location, use such as industry, energy production, and irrigation, and source (surface or groundwater) since 2008. Local databases provide additional information on water management such as the location of the main withdrawing point for snowmaking (“Withdrawing point SAP”), weekly water volumes stored in each reservoir for snow production between 2007 and 2020, contribution of each reservoir to snow production over each ski piste, synoptic diagram of the water supply networks, monthly volume abstracted for and released from reservoirs for domestic water supply between 2016 and 2020. For reasons of national security, the exact location of the pumping structures for domestic water use is not publicly available, and only an approximate location is indicated on Fig. 2 (see “Withdrawing point BNPE”).
3.5 Climate change simulations
In addition to simulations for past meteorological conditions based on the SAFRAN reanalysis from 1961–2020 (Vernay et al., 2022), we performed simulations under future climate change conditions. These simulations are based on one model run of the GCM/RCM pair CNRM-CM5/ALADINv6, part of the EURO-CORDEX ensemble (Jacob et al., 2014; Kotlarski et al., 2022) for the high-emission scenario RCP8.5 for a 15 year time period in the middle (2043–2057) and at the end (2083–2097) of the 21st century. These simulations are carried out using the Crocus snow cover model configuration introduced earlier, driven by the RCM model output adjusted with the quantile mapping adjustment method ADAMONT (Verfaillie et al., 2017) using the SAFRAN reanalysis as a reference atmospheric dataset (see Verfaillie et al., 2018 for more details). These simulations are processed similarly to the SAFRAN-driven simulations and are meant to illustrate how the results obtained in past and current climate conditions are potentially modified under a warmer climate – although this study does not address the diversity of the sources of uncertainty involved in climate change simulations. Note that the multi-annual temperature and precipitation values at the scale of the French Alps at 1800 m elevation, for the ADAMONT-adjusted results of the CNRM-CM5/ALADINv6 GCM/RCM pair, match the median of an ensemble of 19 adjusted GCM/RCM pairs, for the time period 2090–2099 under RCP8.5 (Monteiro et al., 2022). This choice of GCM/RCM pair can, therefore, be considered as representative of the mean climate change signal, pending further investigations using an ensemble of climate model simulations.
4.1 Hydrological effects at 1800 m elevation
Grooming and snowmaking influence the characteristics of the snowpack, and this translates into its hydrological behaviour. As an example, Fig. 4 displays, for the winter season 2019–2020, the time evolution of the snow depth for natural, grooming and grooming and snowmaking simulations at 1800 m elevation in the Vanoise massif, typical of the situation of the La Plagne ski resort. Several key features are highlighted in this figure. First of all, it shows that simulations with groomed snow result in lower snow depth, due to the compaction induced by the grooming process.
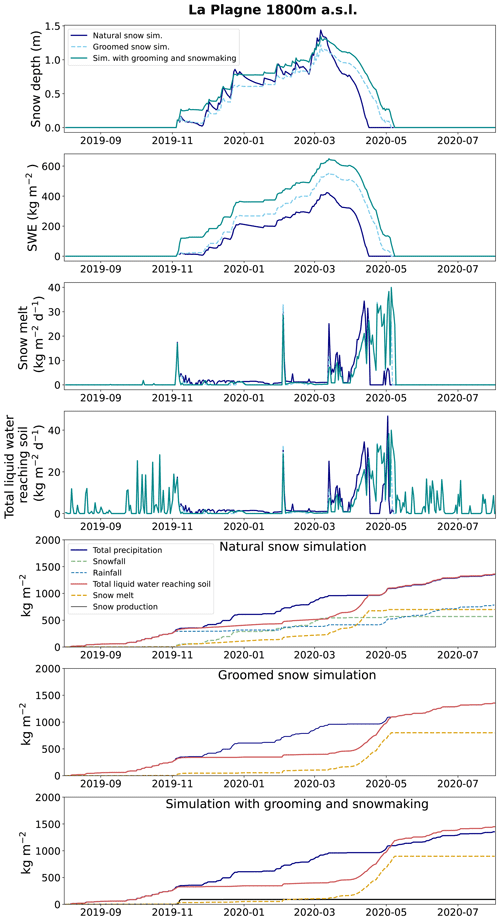
Figure 4Model simulations at 1800 m elevation, representative for the La Plagne ski resort, with natural snow simulation (solid blue line), groomed snow simulation (light blue dashed line) and the simulation with grooming and snowmaking (solid green line), for the hydrological year 2019–2020. From top to bottom, the panels display snow depth, snow water equivalent (SWE), snow melt, liquid water reaching soil (snow melt flux and rainfall on snow-free ground) and cumulative water fluxes for the three simulations. These show total precipitation (solid blue line), snowfall (dashed green line), rainfall (dashed blue line), liquid water reaching soil (solid red line), snow melt (dashed orange line). The last panel further shows water demand for snowmaking (solid black line).
At the end of the season, the snow melt-out date occurs later in groomed snow simulations compared to natural snow simulations. This effect was described and analysed in Spandre et al. (2016a). It is due to the generally higher snow density due to grooming, thus higher thermal conduction through a groomed snowpack, leading to more efficient loss of energy from the snowpack (and underlying ground), especially at night, progressively inducing colder conditions in the snowpack (and underlying ground) and delaying melt processes.
Simulations with snowmaking lead to higher snow depth values since the beginning of the season (due to additional snow mass added to the snowpack through snowmaking). The melt-out date is further delayed compared to grooming-only simulations (but comparatively less than between groomed and natural snow simulations), due to the additional mass that needs to be melt until complete melt-out. The cumulative total precipitation over the entire hydrological year matches exactly the cumulative total liquid water reaching soil for the natural snow and grooming snow simulations, which indicates that evaporation from the snowpack plays a negligible role in the simulation results. In contrast, when snowmaking is taken into account, the cumulative value of total liquid water reaching soil exceeds total annual precipitation, due to the addition of snow through snowmaking.
4.2 Hydrological effects over the gravitational envelope of the ski resort
The aggregated influence of grooming and snowmaking on liquid water reaching soil, at the scale of the entire La Plagne ski resort gravitational envelope (see Fig. 1) is examined in this section. Note that this does not bear direct hydrological relevance because the ski resort is located on independent catchments on different aspects of the mountain over which the ski resort develops. Here, we also assume in this illustrative case that 100 % of the ski pistes are covered with snowmaking.
Figure 5a shows the aggregated amount of liquid water reaching soil for the hydrological year 2019/2020, aggregated only over the ski pistes, i.e. neglecting all areas surrounding the pistes. The figure displays daily (instantaneous) and cumulative (from 1 August 2019 to 1 August 2020) values. In this case, the simulation shows that for most of the winter season, the simulations including grooming (with and without snowmaking) lead to lower values of the total liquid water reaching soil, especially during the winter, than natural snow simulations. In terms of cumulative amounts, not only the cumulative values for the natural snow cover simulation increases steadily during the winter season due to higher basal snowmelt than for groomed or grooming and snowmaking snow simulations, but the late season melt peak starts earlier.
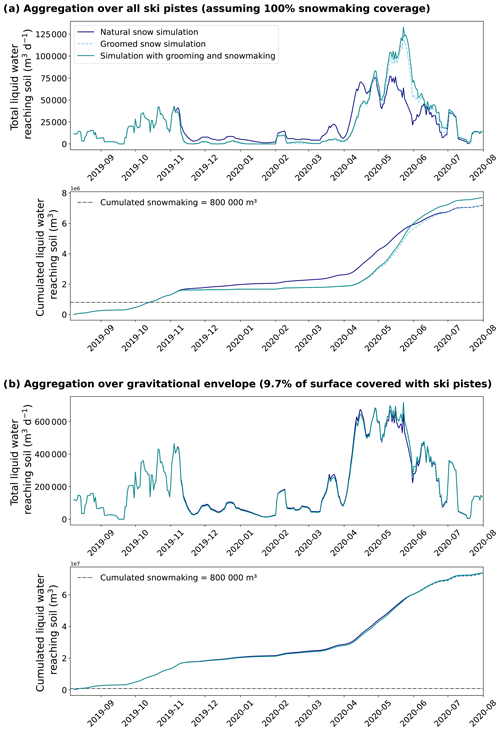
Figure 5Aggregation over all ski pistes (a) and for the entire ski resort gravitational envelope (b) of the daily liquid water reaching the soil and cumulative liquid water reaching soil, respectively, for the natural snow (solid blue line), grooming snow on ski pistes (dashed green line) and grooming and snowmaking on ski pistes (solid green line).
Natural and grooming snow cover simulations converge, in terms of cumulative total liquid water reaching soil, in early June, and the cumulative values remain very close until the end of the season. Simulations including snowmaking converge around the same date, but then keep increasing over the end of the season, exceeding the total amount of liquid water reaching soil by about 800 000 m3, corresponding to 11.1 % of the annual total liquid water reaching soil. Aggregated over the ski pistes only, the results reveal that grooming (with or without snowmaking) exerts a substantial influence on the water balance. This is mostly due to the fact that grooming leads to essentially suppressing winter snowmelt (leading to deficits, reaching more than −40 %, on cumulative total liquid water reaching soil in April), while snowmaking influences mostly the water balance towards the end of the melt season.
Figure 5b provides similar types of results to Fig. 5a, but the aggregation now extends to the entire gravitational envelope of the ski resort. The differences between the configurations is smaller, with a deficit of cumulative total liquid water reaching soil on the order of −5 % as of April for the grooming and grooming and snowmaking configurations on ski pistes, compared to a simulation when natural snow cover processes only are considered for the whole domain. In the case when snowmaking is taken into account (assuming full coverage of the ski pistes with snowmaking equipment), the excess in total annual liquid water reaching soil amounts to 1.1 % of the value for natural snow processes only. The difference between the results of the two scales of aggregation (Fig. 5a and b) is directly linked to the surface area covered with ski pistes, which is generally on the order of 10 % in French ski resorts (François et al., 2016), and specifically 9.7 % for the La Plagne ski resort.
4.3 Hydrological effects at the catchment scale
The goal of this section is to assess the water footprint of a specific use (snow management in ski resorts), using natural resources as a reference. Four sources of available river discharge data are used to characterize natural resources, as introduced in Sect. 3.4, focusing on the Frasses and Bonnegarde catchments. Figure 6 shows the river discharge of the Frasses River at the Gros/CTGREF gauging station (see Fig. 2) from various observational and modelling sources. Note that this gauging station corresponds almost to the outlet of the Frasses catchment.
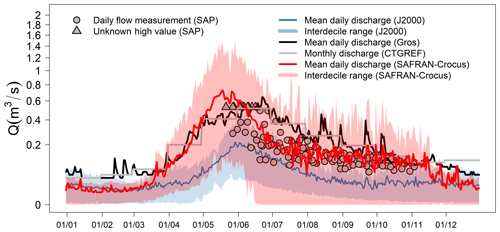
Figure 6Annual hydrographs derived from available discharge observation data and simulation results for the Frasses catchment. Data from the J2000 model are those simulated at the gauging station Gros/CTGREF: the shaded area indicates the interdecile range, and the mean hydrograph is in dark blue. Monthly discharge for 1980 from CTGREF are displayed in grey. The Crocus results correspond to the aggregation of the total water reaching soil for the Frasses catchment, spanning the time period from 1961 to 2020. The grey points are flow measurements made by SAP, identified on the x axis by the Julian day of the recordings. The y axis displays a square-root scale.
The SAP data are both incomplete (e.g. some hydraulic conditions did not allow current measurements; see the filled up-pointing triangle in Fig. 6) and influenced by upstream abstractions (e.g. the Prajourdan reservoir has been supplied by the water intake on the Frasses River since 2001). The SAP data were, thus, not considered as a reference.
As shown in Fig. 6, the other three data sources suggest a nival (snow-dominated) river flow regime with a peak in June due to snowmelt. They show similar hydrographs, and time series are highly correlated (for example, R2=0.83 between Gros and CTGREF data on a monthly scale and R2=0.73 between Gros and J2000 data on a daily scale). However, they are not perfectly consistent in terms of annual volume. The annual flow is equal to 49 L s−1 according to the J2000 model, whereas measurements at the Gros/CTGREF gauging station estimate an annual flow of around 200 L s−1. The outputs of the J2000 model do not appear to be usable because of the bias identified in the annual flow, perhaps due to the excessively coarse resolution of the meteorological forcings (64 km2) in relation to the size of the catchment. In contrast, the aggregation of the “total water reaching soil” from the SAFRAN-Crocus for the Frasses catchment (using simulations of natural snow around ski pistes and only taking grooming into account on ski pistes and spanning the time period 1961–2020), shows a better agreement with the CTGREF and Gros datasets given that the annual flow simulated by SAFRAN-Crocus is equal to 162 L s−1. However, Crocus-based results show too low discharge values (even unrealistic zero-flow conditions in some cases; see the red envelope in Fig. 6) in wintertime leading to R2=0.64 between SAFRAN-Crocus and CTGREF, which we interpret as the consequence of the lack of a genuine hydrological modelling framework in this case, leading to ignoring, e.g. groundwater processes sustaining winter baseflow. We also note that the variables used from the SAFRAN-Crocus simulations do not take into account evapotranspiration. However, this figure shows that the catchment-level aggregation SAFRAN-Crocus simulations of total water reaching soil bear hydrological relevance compared to observed datasets and can be used further, especially for comparing simulations performed using different configurations in terms of snow-related processes.
Finally, mean daily flows from Gros data are used later as reference values for natural resources rather than CTGREF data, which are only representative of a single year. It should be noted that the observation period linked to the Gros records is old (1948–1968) but nevertheless still seems representative of the recent period (based on a comparison, even though it is questionable, with the mean annual hydrograph provided by J2000 over the period 1980–2016 and with the measurements made by SAP in autumn when abstractions are lower).
We now introduce results focusing on the two catchments Frasses and Bonnegarde (Frasses is a subcatchment of Bonnegarde; see Fig. 2).
The approach considered here is purely empirical: it is based on a water balance model applied to observed data and results from snow cover simulations at different time scales (from week to year).
Only first-order estimates of the water footprints developed along the river system can be expected due to limited direct information on the snowmaking process, water abstraction and consumption. For example, the water balance is partly biased by non-concomitant data periods (dating back to the 1940s and 1960s for water resources and after 2007 for water abstraction), whereas the demand for water for snowmaking and reservoir management is directly linked to the meteorological and hydrological conditions of the year.
The Bonnegarde catchment is ungauged. There is only one gauged catchment in the neighbourhood (the Frasses catchment), and the hydrological distributed model J2000 fails to reproduce reliable discharges at the gauging station Gros/CTGREF. The drainage-area ratio method was thus applied considering that the runoff per unit drainage area is equal over the ski resort catchment.
The other working assumptions for the calculation of the water uses are the following:
-
In the following, values are negative when they relate to abstraction, loss or consumption of water.
-
Monthly volumes for water domestic use are evenly distributed within the month at weekly time step. When water used for domestic purposes is abstracted and consumed in the catchment, the water returns to the river system with an efficiency rate of 85 % (https://www.services.eaufrance.fr/commune/73150, last access: 6 November 2023). When the water is returned outside the catchment area, a total loss of 100 % is assumed.
-
Only the water for snow production (WR) is taken into account in the calculation of the water balance. The impact of the Prajourdan reservoir has been included in the calculations for both catchments. The other two reservoirs Forcle and Lovatière have an influence limited to the Bonnegarde catchment. Transfers between reservoirs do exist but are neglected.
-
Values for the volumes of snow produced (SP) are given by SAP for each equipped slopes and are aggregated at the catchment scale in proportion to the length of the equipped slope crossing the catchment.
-
The effect (SGE) of the snow grooming and snowmaking is provided by the simulated values of total liquid water reaching soil described above. This effect is given by the average difference between natural snow and grooming and snowmaking simulations of total liquid water reaching the soil at the weekly time scale over the period 1961–2020 and for the entire ski resort gravitational envelope. The values obtained are then divided by the total volume to provide a dimensionless pattern for each ski piste. The local impact of grooming and snowmaking is calculated for each ski piste as the sum of this pattern multiplied by 60 % of the total volume of water used for snowmaking, and the mean pattern of total liquid water reaching soil simulated considering natural snow, multiplied by 30 % of the total volume of water used for snowmaking. A total of 10 % is considered to be definitely lost by evaporation and sublimation of the water droplets during the snowmaking process.
-
The hydrological alteration due to grooming and snowmaking including reservoir management is the sum of WR and SGE.
The results are presented starting the series at week 31 (which includes 1 August) in Figs. 7 and 8. The colours on these two figures were chosen to better distinguish the different types of water use (green and blue for domestic use and for grooming and snowmaking, respectively) from natural surface water availability (black). The shades of blue distinguish between the solid (S, dark blue) and liquid (L, light blue) phases of water. Interannual averaged values for week 53 are added to those for week 52. Because of the skewed distribution of mean weekly discharges, the y axis is represented with a square root scale. Negative values represent water losses. The water balance is computed at the seasonal and yearly time scales (Table 1), from data with distinct periods of availability (e.g. 2007–2020 for reservoir management, 2014–2020 for observations of water used to produce snow, 2016–2020 for observations of domestic water use, and 1948–1968 for observations of water resources (river discharge), 1961–2020 for snow cover modelling including the effect of grooming and snowmaking). Hence, the results can be viewed as a “typical” water year, compositing information from different time periods, due to the heterogeneities of the data sources. The “volumes” of snow-related quantities in Figs. 7d and 8d correspond to snow water equivalent.
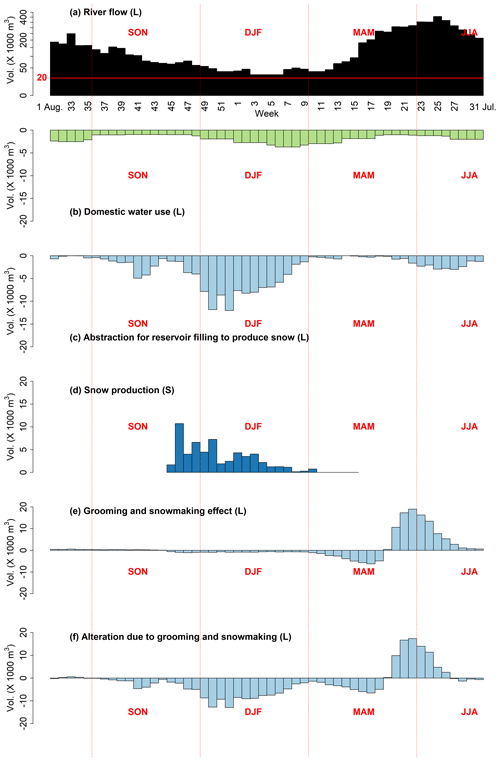
Figure 7Synthesis of water resources, abstraction and consumption for the Frasses catchment including water phases (S: solid; L: liquid). The red line in panel (a) represents the ecological flow for the corresponding catchment. SON: September–October–November; DJF: December–January–February; MAM: March–April–May; JJA: June–July–August.
Table 1Water balance at the catchment scale (× 1000 m3). DJF: December–January–February; MAM: March–April–May; JJA: June–July–August; SON: September–October–November.
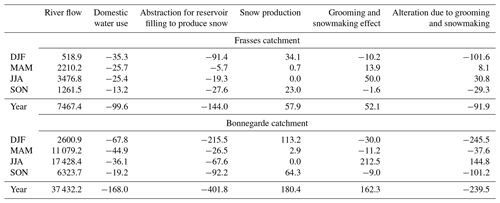
Figures 7a and 8a show that the seasonal pattern of river flows for both catchments is typical of snowmelt-dominated regimes with high flows in spring or early summer and winter minima caused by freezing. The different types of water use alter in different ways the river flow regime. The influence of water supply (Figs. 7b and 8b) is highly variable in both space and time even within a rather small ski area. According to the schematic diagrams of water supply, all of the water amounts abstracted for domestic uses within the Frasses catchment are consumed and returned to rivers outside the catchment. One part of the water supply is abstracted and consumed within the Bonnegarde catchment, and the other part is abstracted within and exported directly from the sources to downstream areas. Water abstraction for domestic use is closely linked to tourist activities; two peaks occur, one during the ski season (December to April) and the other during the summer (July and August) (Figs. 7b and 8b).
The patterns of weekly water abstraction for snowmaking (Figs. 7c and 8c) is quite similar for the two catchments. However, analysing abstracted volume at the catchment scale hides heterogeneities. The seasonal patterns differ from one reservoir to another and from one year to another, due in particular to technical constraints (availability of an overflow on intakes shared with the water supply) and regulatory constraints (compliance with ecological flows). Withdrawals to the Prajourdan reservoir (see Fig. 1) are concentrated in autumn and winter, while those to the Lovatière reservoir are evenly distributed throughout the year and those to the Forcle reservoir are concentrated in summer.
This analysis enables us to study the influence of grooming and snowmaking on river flow. The results show that they modify river flows throughout the year except in summer. Snow is first produced to provide a ground layer of snow at the beginning of the winter season (November–December) and afterwards (up to end-March) to maintain a minimum snow depth for skiing (Figs. 7d and 8d). Melting processes as described in the previous section are delayed, and the snowmelt peak is observed between April and May (Figs. 7e and 8e).
The effect of the grooming and snowmaking operations on river flow regime (Figs. 7f and 8f) is the sum of individual alterations. Alterations are mainly due to abstractions for filling reservoirs. Grooming and snowmaking modify the natural flow regime of rivers crossed by the ski area in different ways. At the catchment scale, the first modification is linked to the process of filling the reservoirs for snow production; the filling up is constrained by regulations on ecological flows and by the water availability in reservoirs shared with other uses (water supply for domestic use in the La Plagne ski resort). The filling starts in autumn, before the first phase of snow production. The second effect is related to the presence of groomed snow on the ski pistes and on the hillsides. In winter, grooming leads to more severe low flows while, later, river flows increase due to increased amount of snowmelt water in spring. When points and sources for water abstraction, and equipped ski pistes, are located within the same basin, the effects are only apparent at the seasonal scale (machine-made snow then plays the role of an additional buffer reservoir with storage in autumn and release in next spring) and rather neutral on the annual scale in terms of water resources.
In the two catchments, snowmaking causes water losses because water abstracted for production is transferred to other nearby areas; the water balance is overall negative for both catchments (see the last column in Table 1), but it is overall positive for the surrounding areas, which benefit from the water imported from the reservoirs (not shown here). Note also that the pressure on the resource is not evenly distributed within the year. The pressure is highest in winter, with a contribution of snowmaking use to the total withdrawals of around 20 % of the resource for the Frasses catchment and around 10 % for the Bonnegarde catchment. The balance for domestic water use (first column in Table 1) is also negative for the same reasons as for snowmaking use (transfer to neighbouring areas). The pressure on water resources is similar in terms of annual volume but less contrasted over the year. The pressure from domestic uses is at its highest during the touristic season, as it is for snowmaking. This is the low-water period in mountainous areas, and this use may compete with water supply for domestic use (Leroy, 2015).
4.4 Hydrological effects under past and future climate in the Bonnegarde catchment
Figure 9 illustrates the hydrological effects of grooming and snowmaking of the Bonnegarde catchment under past climate (1986–2015, based on the SAFRAN reanalysis) and for two future time periods in the middle (2050: 2043–2057) and at the end (2090: 2083–2097) of the 21st century using the adjusted GCM/RCM pair CNRM-CM5/ALADINv6 for the high-emission scenario RCP8.5. Note that the Bonnegarde catchment (see Fig. 1) has a surface area of 23.61 km2, and 19.4 % of its surface area is covered by ski pistes, 36.2 % of which is equipped with snowmaking equipment. Overall, 7.0 % of the Bonnegarde catchment is covered by snowmaking equipment.
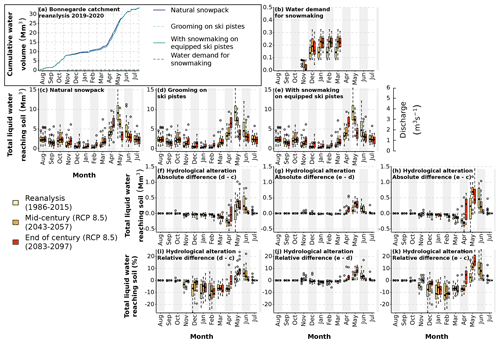
Figure 9Overview of the hydrological alteration due to grooming and snowmaking in the Bonnegarde catchment, based on the SAFRAN-Crocus simulations. Simulations take into account either natural snow only (c), grooming on ski pistes and natural snow around the pistes (d), and grooming on ski pistes and snowmaking on the parts of the ski pistes equipped with snowmaking equipment, and natural snow around the pistes (e). Here we do not take into account the water abstraction used for snowmaking (similar to Fig. 5). Panel (a) shows the aggregated total water reaching soil for the whole catchment for the hydrological year 2019–2020 (similar to Fig. 5). Panels (b) to (k) show various components and visualizations of the local hydrological cycle under past climate conditions (reanalysis, 1986–2015) and using climate projections (RCP8.5, centered on 2050 (2043–2057) and 2090 (2083–2097)). Panels (c) to (e) show the distribution of monthly aggregations of total water reaching soil under these three climate contexts. Panel (b) shows the cumulative water demand for snowmaking under these three climate contexts. Panels (f) to (h) show the hydrological alteration, here computed as the difference between monthly aggregated total water reaching soil between different configurations and with various references (natural snow only, or taking into account grooming on ski pistes). Panels (i) to (k) show the relative alteration, i.e. same as panels (f) to (h) but relative to the corresponding reference values.
Figure 9a shows the cumulative total water reaching soil over the Bonnegarde catchment for three different configurations (natural snow over the full catchment, grooming on ski pistes and natural snow around, and grooming on ski pistes, snowmaking on equipped ski pistes, and natural snow around), using a similar representation as in Fig. 5.
Figure 9c shows the evolution of the total liquid water reaching soil over the catchment, using only natural snow conditions, showing the typical trend towards an earlier snowmelt peak under a warming climate in mountainous catchments (Hock et al., 2019). Under past climate, the peak flow occurs in May, and this shifts to April in future climate projections. Figure 9d shows similar information, but accounting for grooming on ski pistes. A similar pattern is observed. The alteration, i.e. the monthly difference compared to the natural snow conditions, is shown in absolute terms on Fig. 9f and relative terms on Fig. 9i. The main effect, as shown in previous sections, is a lower snowmelt flux during the wintertime, low flow period, on the order of −10 % to −20 %, and a higher value later in the season, consistent with previously introduced results. Note that this alteration corresponds almost exactly to the fraction of the catchment covered by ski pistes and is directly due to the quasi-suppression of wintertime snowmelt on groomed ski pistes (with or without snowmaking).
Figure 9b shows the total water demand for snowmaking on ski pistes equipped with snowmaking in this catchment. It shows that, for the early-season period (especially November), the amount of snowmaking decreases in a warmer climate, consistent with the fact that conditions favourable for snowmaking become less frequent (Spandre et al., 2019; Morin et al., 2021; François et al., 2023). However, in a warmer climate, water demand for snowmaking increases in the second half of the season, due to increasingly frequent situations with low snow depth values (below 60 cm), triggering snowmaking in the model simulations as soon as wet bulb temperature values are below the threshold. This mostly occurs in January. Overall, in a warmer climate, total water demand tends to be larger than in the reference simulations 1986–2015, although the water demand is not larger for end of century than for mid-century, most certainly due to the fact that “cold-windows” appropriate for snowmaking become increasingly rare and inhibit the capacity to operate snowmaking facilities.
Figure 9e shows the aggregated total liquid water reaching soil, in the situation where snowmaking is implemented on equipped ski pistes of the Bonnegarde catchment. It does not show major differences to simulations ignoring snowmaking (Fig. 9c).
Figure 9g shows the difference, for each future time period, between the simulations with and without snowmaking (on ski pistes equipped with snowmaking), in absolute terms, and for Fig. 9j in relative terms. It shows that the impact of snowmaking on the monthly total liquid water reaching soil is maximum in June under past climate conditions, and progressively shifts towards earlier time period under a warmer climate, peaking to 10 % difference, on average, in May, at the end of the 21st century under RCP8.5.
Figures 9h and k show the absolute and relative alteration between the simulations accounting for grooming and snowmaking, and the fully natural snow conditions. There, the two effects of grooming and snowmaking combine. Grooming leads to lower low flows in wintertime, while snowmaking leads to increased snowmelt amount in April, May and June, depending on the climate context (earlier peak in alteration in a warmer climate).
This very limited change is mainly due to the fact that, while the annual amount of snowmaking shows a substantial increase in the simulations, it corresponds to an overall limited fraction of the local water resources at the scale of the catchment. The impact of snowmaking on monthly total liquid water reaching soil remains lower than shifts in (natural) snow melt seasonal patters due to climate change, both in terms of annual and monthly values, and does not substantially counteract the effect of climate change on the shift in snow melt pattern, even if snow management leads to a delayed snow melt on ski pistes.
The present study explores the influence of grooming and snowmaking on the hydrological regime of alpine catchments within which ski pistes are located. It introduces a method for such an investigation, based on numerical modelling, which is illustrated and demonstrated using the case study of the La Plagne ski resort, specifically. Prior to discussing the implications of the results, we first highlight some of the key limitations of this work.
5.1 Limitations
The current study introduces a method, based on existing snow cover modelling tools designed to carry out numerical simulation of the snow cover in ski resorts (Spandre et al., 2016a; Hanzer et al., 2020; Ebner et al., 2021), in order to assess the influence of snow management on the hydrology of associated catchments. Using such tools enables us to simulate the hydrological role of the snow cover, with and without grooming and snowmaking, yet this does not pretend to encompass the complexity of hydrological processes that a detailed hydrological model would allow us to account for. For example, our focus on the variable “total water reaching soil”, obtained as the sum of (i) liquid water flowing at the bottom of the snowpack and (ii) rainfall on snow-free ground, and aggregated over a given catchment, does not take into account other key hydrological processes such as subsurface flow and evapotranspiration. The absence of subsurface flow is most probably responsible for the fact that the wintertime river runoff in the Frasses river from this modelling approach, as shown in Fig. 6, is underestimated compared to available observations, in particular cases of discharge values equal to zero. However, the simulations compare rather favourably in terms of discharge at other time periods of the year, which indicates that the proxy variable “aggregated total water reaching soil” is relevant to assess the differences between two model simulations using various configurations. Despite these limitations, our modelling approach also brings new results, which could not be obtained from a classical hydrological model. For example, our study illustrates the substantial role of grooming on the physical behaviour of the snowpack and, in particular, basal melt, which is essentially suppressed due to grooming. Such results would not be revealed using snowpack models not accounting for snow physics, in a way sufficiently detailed so as to account for the influence of the grooming process. For example, snowpack components of hydrological models, which are formulated in terms of a single snow mass reservoir (snow water equivalent), with a melt rate formulation only dependent on air temperature and, potentially, radiation (temperature-index models), cannot account for the different physical behaviour of snow on ski pistes, compared to the surrounding natural snow cover. Likewise, our approach enables us to explicitly account for snowmaking on the snowpack properties. Overall, our approach provides a quantitative appraisal on the influence of grooming and snowmaking on the water budget of the related catchment.
The current study can be used to trigger the development of more complex, integrated modelling approaches, within which snow cover processes can be represented consistently with the approach described here, while hydrological processes can be represented with more details. This also applies to the representation of the balance between the water demand induced by snowmaking, and the water availability from various sources, including the fact that water can be withdrawn from one catchment, stored, and used in another catchment. However, developing models capable of representing this full complexity through a genuine, two-way coupling between snow cover simulation, including snowmaking, and the hydrological functioning of mountainous catchments at the local scale of a few hundreds of metres of horizontal resolution, represents a very challenging endeavour (Hanzer et al., 2014). Furthermore, this approach would be difficult to transfer to other locations. In contrast, we suggest that our approach can be further tested by other teams and used in combination with other data sources, in order to better assess the balance between water demand and water availability, and local and downstream impacts, and contribute to better informing discussions relevant to water management in mountain regions.
Hydrological model simulations derived from J2000 were assessed but not used due to strong biases. Nevertheless, future work could make use of such models using more adequate meteorological driving data in mountainous areas.
5.2 Implications
Notwithstanding the limitations highlighted above, this study brings novel insights into the influence of snow management on the hydrology of mountain catchments within which ski resorts are located. While the detailed results are provided and directly applicable to the case study of the ski resorts of La Plagne, in the Northern French Alps, this study bears implications for the related scientific domain and for water resources management and ski resorts in mountainous areas. First of all, our results highlight that the hydrological effect of grooming and snowmaking scales, to a large extent, with (i) the surface area covered with ski pistes, driving the deficit in winter snowmelt due to grooming, and (ii) the fractional snowmaking coverage, driving the amount of excess snowmelt due to snowmaking (and the corresponding amount of prior water abstraction). Further, the influence of snowmaking is directly related to the ratio between the amount of machine-made snow produced and total precipitation (aggregated over the surface equipped with snowmaking). In fact, our study supports the following equation describing the annual-scale alteration of water resources affected by snowmaking:
where A is the annual-scale alteration due to snowmaking (unitless, in relative terms), Psnowmaking is the annual amount of water used for snowmaking per unit surface area of ski pistes equipped with snowmaking, xsnowmaking is the efficiency of the liquid-water-to-snow mass conversion (90 % typically), Pannual is the annual precipitation amount falling per unit surface area, Spistes and Scatchment are the surface area of the ski pistes and catchment, respectively, and Rsnowmaking represents the fraction of the ski pistes surface area equipped with snowmaking.
In the case of the La Plagne ski resort studied here, the annual snow production corresponds to 10 % to 20 % of the annual precipitation on ski pistes. Ski pistes cover about 10 % of the surface area of the entire ski resort. Taking into account the snowmaking fractional coverage (on average 40 % in La Plagne), this leads to 0.4 % to 0.8 % of the total water discharge aggregated over an entire year. The upper value can be increased at the local scale, in cases where the proportion of ski pistes in the catchment is higher (up to 20 %) and the snowmaking fraction coverage is higher (up to 80 % typically), or if the amount of water used for snowmaking corresponds to a higher fraction of annual precipitation. In the case where the water is taken from a different catchment, this leads to a net increase in water discharge in the catchment where the ski resort is located, compared to an undisturbed case. In cases where at least part of the water abstraction takes place in the same catchment, then the net alteration at the annual scale is smaller than A, due to the compensation, to a large extent (except the 10 % evaporation, see below), between water uptaken for snowmaking and water resulting from the melt of the produced snow on ski pistes.
Our results enable us to discuss to what extent water is only “borrowed” to be used for snowmaking. This term is often mentioned by snowmaking promoters and condemned by opponents, who consider that water withdrawn for direct or indirect (through filling reservoirs) use for snowmaking is lost. Besides the 10 % evaporative water loss during the process of ice droplet solidification into ice spheres which forms the basis of snowmaking (Eisel et al., 1988, 1990; Spandre et al., 2016b; Grünewald and Wolfsperger, 2019), our results indicate that, in the situation prevailing in the Northern French Alps, there is little to no further loss of water through snowmaking. This situation is in contrast with the substantial water loss associated with irrigation, in agriculture (Boulet et al., 2020), to which snowmaking has been compared (Steiger et al., 2019). Water storage for agriculture is often questioned, in particular due to the fact that strong evaporation from the reservoirs can occur, leading to direct loss of stored water (Rodrigues et al., 2020), and that water used for irrigation is lost through the evapotranspiration of the agricultural plants and soils. Due to colder temperature prevailing at the location where water reservoirs used for snowmaking are built, it is likely that the evaporation rate from such reservoirs is much smaller than from lower elevation agricultural reservoirs. Nevertheless, this needs to be assessed in detail. Through these two aspects (lack of “evapotranspiration” from the snowpack, and different, probably lower evaporation rate from water storage reservoirs), it appears that snowmaking differs markedly from water use for agriculture irrigation in lowlands, and thus this comparison may be partially misleading from a water management perspective. To a large extent, it appears from our study that the effect of snowmaking on the hydrological regime of the catchments affected by the presence of ski resort is rather neutral at the annual scale and mostly operates at the seasonal scale by storing water masses temporarily, on the order of a small fraction of the water at the scale of the catchments (typically less than 1 % to 2 %), either in reservoirs prior to snowmaking or in the form of snow itself through snowmaking. In terms of water quantities, snow grooming and snowmaking mainly lead to shifting the water cycle in time (at the seasonal scale) and space (through water transfers in some cases). Such shifts can have consequences for water resources management at the local scale, especially in cases where (i) wintertime abstractions in river occur for other uses of water, because snow grooming tends to lower the river discharge in wintertime and (ii) water abstractions for snowmaking take place at times and locations where conflicts for water use can be encountered. Tools such as those developed in this study could be used in the future to better assess and manage such situations at the local scale.
This study is consistent with the conclusions of IPCC (2019): “Integrated water management approaches across multiple scales can be effective at addressing impacts and leveraging opportunities from cryosphere changes in high mountain areas. These approaches also support water resource management through the development and optimization of multi-purpose storage and release of water from reservoirs (medium confidence), with consideration of potentially negative impacts to ecosystems and communities.” However, we highlight here that this study does not address all the issues related to the impacts of ski resort operations and snowmaking on mountainous environments. The influence on the water cycle, quantified in this study, is only addressed in terms of the effects of snow grooming and snowmaking (hence, not accounting for other water uses related to tourism activities, including housing), and only in terms of water quantity but not water quality. Also, water storage for snowmaking is only considered here in terms of water amounts and the related alteration of the water cycle but does not address other environmental impacts on biodiversity and mountain landscape related to the construction and use of mountain water reservoirs and surrounding ski resorts. Implications relevant to the positioning of snowmaking and ski tourism within the future path development of mountain tourism and broader mountain economies (Steiger et al., 2022; Berard-Chenu et al., 2023; Scott et al., 2022; François et al., 2023) are beyond the scope of this particular study, which solely focuses on downstream hydrological impacts.
5.3 Future climate
Our analysis is also based on future climate change simulations. The results indicate that the impact of snowmaking on the hydrological cycle at the local scale will moderately increase in a warmer climate, although the impact of climate change on the hydrological balance of the related catchment (in particular, earlier and smaller snowmelt peak) will be of a larger magnitude. This method, pending the use of a larger set of climate change simulations to better quantify the uncertainties at play, can be used to provide quantitative information combining the influence of (i) grooming and snowmaking and (ii) climate change on the local water cycle in mountain catchments within which ski resorts are located. This will require the use of a full ensemble of climate change projections in order to provide quantitative assessments of the rate of change, along with the uncertainties at play (Verfaillie et al., 2018; Gerbaux et al., 2020). Furthermore, using ensembles of climate change simulations will enable to compute the time evolution of water resources in the ski resorts under consideration, for future climate conditions. Simulations carried out in this work were performed using current snow management rules and technical configurations, which may evolve in the future, due to potential changes in the technologies used for snow management (snowmaking equipment, grooming techniques, etc.) but also changes in the operational practices implemented in ski resorts, including those due to future changes in skier demand and other future changes in the local economies and the future role of ski tourism.
This study introduces a new framework for analysing the impact of snow management on the hydrological regime at the scale of individual ski resorts. The method was implemented and demonstrated results for a case study of the La Plagne ski resort. Its generic formulation makes it possible to be replicated for other ski resorts and related catchments. The results of the case study at La Plagne show a substantial influence of the ski resorts snow management operations at the local scale on the seasonality of runoff amounts, especially due to grooming in winter. The effects of snowmaking on runoff generation occurs mainly at the time of snowmelt, and its magnitude depends on the geographical scale of aggregation, up to about 1 % to 2 % under current climate for the catchments under consideration.
The study provides hints into the impact of future climate change on the hydrological alteration due to grooming and snowmaking. For the catchments under consideration, the amount of snowmaking, under current snowmaking practices and technology, increases in a warmer climate, which is consistent with previous studies (Spandre et al., 2019). However, at the catchment scale, the main influence of climate change on the hydrological regime proceeds through the well documented earlier and smaller (mostly natural) snowmelt peak (Hock et al., 2019), over which the influence of grooming and snowmaking overimposes a secondary modulation. Thus, under current and future climate conditions, grooming and snowmaking appear to have a rather limited impact on total runoff at the catchment scale. The main technical issue raised by the use of snowmaking in a warmer climate is the ability to have sufficient water available for snowmaking operations (e.g. Gerbaux et al., 2020).
Although our study provides quantitative estimates of the impact of grooming and snowmaking on the hydrological regime of mountain catchments influenced by the presence of ski resorts using a modelling framework, it also reveals the complexity of using in-situ hydrological observations in such contexts. Often, the data do not exist or are too incomplete to provide a consistent picture, based on observations, of the hydrological context. This is a clear area of potential improvement for better integrated water management in mountain areas, under current and future climate conditions (Hock et al., 2019), bringing together integrated modelling approaches with a broader range of in-situ observations. We hope and expect that forthcoming studies will provide additional insights on the complex social-ecological challenges faced by mountain areas under global change.
The Crocus snow cover model used for this work was developed inside the open-source SURFEX project (http://www.umr-cnrm.fr/surfex/, UMR-CNRM, 2023).
Data used in this article are available from the authors upon reasonable request. Data displayed on Fig. 9 are available from this address: https://doi.org/10.5281/zenodo.8408515 (Morin et al., 2023).
SM, HF and ES designed the research; MR, HF, ES, LC, FB and EL produced the data; MR, HF and ES produced the figures and the table with support from the co-authors; all authors contributed to the analysis and interpretation of the results; SM wrote the paper, using feedback from all the co-authors.
The contact author has declared that none of the authors has any competing interests.
Publisher's note: Copernicus Publications remains neutral with regard to jurisdictional claims made in the text, published maps, institutional affiliations, or any other geographical representation in this paper. While Copernicus Publications makes every effort to include appropriate place names, the final responsibility lies with the authors.
The project conducive to this article has received funding from Compagnie Des Alpes and the European Union's Horizon 2020 research and innovation programme (Grant agreement no. 730203). We thank the three anonymous reviewers and the topical Editor Elena Toth for insightful comments and suggestions, which led to improving this manuscript through the editorial process. CNRM/CEN, IGE and LESSEM belong to Labex OSUG@2020.
This research has been supported by Horizon 2020 (grant no. 730203) and Compagnie des Alpes.
This paper was edited by Elena Toth and reviewed by three anonymous referees.
Abegg, B., Morin, S., Demiroglu, O. C., François, H., Rothleitner, M., and Strasser, U.: Overloaded! Critical revision and a new conceptual approach for snow indicators in ski tourism, Int. J. Biometeorol., 65, 691–701, https://doi.org/10.1007/s00484-020-01867-3, 2020. a
Berard-Chenu, L., François, H., George, E., and Morin, S.: Past changes in natural and managed snow reliability of French Alps ski resorts from 1961 to 2019, The Cryosphere, 16, 863–881, https://doi.org/10.5194/tc-16-863-2022, 2022a. a
Berard-Chenu, L., François, H., George, E., and Morin, S.: Snowmaking Development Trajectories in French Alpine Ski Resorts: The Influence of Local Specificities and Regional Support Policies, Journal of Alpine Research, 110, 4, https://doi.org/10.4000/rga.10465, 2022b. a
Berard-Chenu, L., Fran cois, H., Morin, S., and George, E.: The deployment of snowmaking in the French ski tourism industry: a path development approach, Curr. Issues Tour., 26, 3853–3870, https://doi.org/10.1080/13683500.2022.2151876, 2023. a
Boulet, G., Jarlan, L., Olioso, A., and Nieto, H.: Chapter 2 – Evapotranspiration in the Mediterranean region, in: Water Resources in the Mediterranean Region, edited by: Zribi, M., Brocca, L., Tramblay, Y., and Molle, F., Elsevier, 23–49, https://doi.org/10.1016/B978-0-12-818086-0.00002-9, 2020. a
Ebner, P. P., Koch, F., Premier, V., Marin, C., Hanzer, F., Carmagnola, C. M., François, H., Günther, D., Monti, F., Hargoaa, O., Strasser, U., Morin, S., and Lehning, M.: Evaluating a prediction system for snow management, The Cryosphere, 15, 3949–3973, https://doi.org/10.5194/tc-15-3949-2021, 2021. a, b, c, d, e
Eisel, L. M., Mills, K. D., and Leaf, C.: Estimated consumptive loss from man made snow, J. Am. Water Resour. As., 24, 815–820, https://doi.org/10.1111/j.1752-1688.1988.tb00932.x, 1988. a, b, c
Eisel, L. M., Bradley, K. M., and Leaf, C. F.: Estimated runoff from man-made snow, J. Am. Water Resour. As., 26, 519–526, https://doi.org/10.1111/j.1752-1688.1990.tb01390.x, 1990. a, b, c
François, H., Morin, S., Lafaysse, M., and George-Marcelpoil, E.: Crossing numerical simulations of snow conditions with a spatially-resolved socio-economic database of ski resorts: A proof of concept in the French Alps, Cold Reg. Sci. Technol., 108, 98–112, https://doi.org/10.1016/j.coldregions.2014.08.005, 2014. a
François, H., Morin, S., Spandre, P., Lafaysse, M., and George-Marcelpoil, E.: Croisement de simulations numériques des conditions d'enneigement avec une base de données socioéconomiques spatialisée des stations de sports d'hiver : description de l'approche et application aux Alpes françaises, Houille Blanche, 4, 66–84, https://doi.org/10.1051/lhb/2016041, 2016. a, b
François, H., Samacoïts, R., Bird, D. N., Köberl, J., Prettenthaler, F., and Morin, S.: Climate change exacerbates snow-water-energy challenges for European ski tourism, Nat. Clim. Change, 13, 1–8, https://doi.org/10.1038/s41558-023-01759-5, 2023. a, b, c, d
Gerbaux, M., Spandre, P., François, H., George, E., and Morin, S.: Snow reliability and water availability for snowmaking in the ski resorts of the Isère département (French Alps), Under current and future climate conditions, Journal of Alpine Research, 108-1, 6742, https://doi.org/10.4000/rga.6742, 2020. a, b, c, d
Gottschalk, L., Krasovskaia, I., Leblois, E., and Sauquet, E.: Mapping mean and variance of runoff in a river basin, Hydrol. Earth Syst. Sci., 10, 469–484, https://doi.org/10.5194/hess-10-469-2006, 2006. a
Grünewald, T. and Wolfsperger, F.: Water Losses During Technical Snow Production: Results From Field Experiments, Front. Earth Sci., 7, 78, https://doi.org/10.3389/feart.2019.00078, 2019. a, b
Hanzer, F., Marke, T., and Strasser, U.: Distributed, explicit modeling of technical snow production for a ski area in the Schladming region (Austrian Alps), Cold Reg. Sci. Technol., 108, 113–124, https://doi.org/10.1016/j.coldregions.2014.08.003, 2014. a
Hanzer, F., Carmagnola, C. M., Ebner, P. P., Koch, F., Monti, F., Bavay, M., Bernhardt, M., Lafaysse, M., Lehning, M., Strasser, U., François, H., and Morin, S.: Simulation of snow management in Alpine ski resorts using three different snow models, Cold Reg. Sci. Technol., 172, 102995, https://doi.org/10.1016/j.coldregions.2020.102995, 2020. a, b, c
Hock, R., Rasul, R., Adler, C., Cáceres, B., Gruber, S., Hirabayashi, Y., Jackson, M., Kääb, A., Kang, S., Kutuzov, S., Milner, A., Molau, U., Morin, S., Orlove, B., and Steltzer, H.: High Mountain Areas, in: IPCC Special Report on the Ocean and Cryosphere in a Changing Climate, edited by: Pörtner, H.-O., Roberts, D., Masson-Delmotte, V., Zhai, P., Tignor, M., Poloczanska, E., Mintenbeck, K., Alegriáa, A., Nicolai, M., Okem, A., Petzold, J., Rama, B., and Weyer, N., Cambridge University Press, Cambridge, 131–202, https://doi.org/10.1017/9781009157964.004, 2019. a, b, c, d, e
IPCC: Summary for Policymakers, in: IPCC Special Report on the Ocean and Cryosphere in a Changing Climate, Cambridge University Press, 3–36, https://doi.org/10.1017/9781009157964.001, 2019. a
Jacob, D., Petersen, J., Eggert, B., Alias, A., Christensen, O. B., Bouwer, L. M., Braun, A., Colette, A., Deque, M., Georgievski, G., Georgopoulou, E., Gobiet, A., Menut, L., Nikulin, G., Haensler, A., Hempelmann, N., Jones, C., Keuler, K., Kovats, S., Kroner, N., Kotlarski, S., Kriegsmann, A., Martin, E., Meijgaard, E. V., Moseley, C., Pfeifer, S., Preuschmann, S., Radermacher, C., Radtke, K., Rechid, D., Rounsevell, M., Samuelsson, P., Somot, S., Soussana, J.-F., Teichmann, C., Valentini, R., Vautard, R., Weber, B., and Yiou, P.: EURO-CORDEX: new high-resolution climate change projections for European impact research, Reg. Environ. Change, 14, 563–578, https://doi.org/10.1007/s10113-013-0499-2, 2014. a
Kotlarski, S., Gobiet, A., Morin, S., Olefs, M., Rajczak, J., and Samacoïts, R.: 21st Century alpine climate change, Clim. Dynam., 60, 65–86, https://doi.org/10.1007/s00382-022-06303-3, 2022. a
Leroy, E.: Proposition d'interface Science-Société pour la gestion intégrée de la ressource en eau dans un contexte de changements climatiques, Theses, Université Grenoble Alpes, https://tel.archives-ouvertes.fr/tel-01241347 (last access: 7 November 2023), 2015. a, b
Masson, V., Le Moigne, P., Martin, E., Faroux, S., Alias, A., Alkama, R., Belamari, S., Barbu, A., Boone, A., Bouyssel, F., Brousseau, P., Brun, E., Calvet, J.-C., Carrer, D., Decharme, B., Delire, C., Donier, S., Essaouini, K., Gibelin, A.-L., Giordani, H., Habets, F., Jidane, M., Kerdraon, G., Kourzeneva, E., Lafaysse, M., Lafont, S., Lebeaupin Brossier, C., Lemonsu, A., Mahfouf, J.-F., Marguinaud, P., Mokhtari, M., Morin, S., Pigeon, G., Salgado, R., Seity, Y., Taillefer, F., Tanguy, G., Tulet, P., Vincendon, B., Vionnet, V., and Voldoire, A.: The SURFEXv7.2 land and ocean surface platform for coupled or offline simulation of earth surface variables and fluxes, Geosci. Model Dev., 6, 929–960, https://doi.org/10.5194/gmd-6-929-2013, 2013. a
Monteiro, D., Caillaud, C., Samacoïts, R., Lafaysse, M., and Morin, S.: Potential and limitations of convection-permitting CNRM-AROME climate modelling in the French Alps, Int. J. Climatol., 42, 7162–7185, https://doi.org/10.1002/joc.7637, 2022. a
Morel, M., Pella, H., Branger, F., Sauquet, E., Grenouillet, G., Côte, J., Braud, I., and Lamouroux, N.: Catchment-scale applications of hydraulic habitatmodels: Climate change effects on fish, Ecohydrology, 16, e2513, https://doi.org/10.1002/eco.2513, 2023. a
Morin, S., Samacoïts, R., François, H., Carmagnola, C. M., Abegg, B., Demiroglu, O. C., Pons, M., Soubeyroux, J.-M., Lafaysse, M., Franklin, S., Griffiths, G., Kite, D., Hoppler, A. A., George, E., Buontempo, C., Almond, S., Dubois, G., and Cauchy, A.: Pan-European meteorological and snow indicators of climate change impact on ski tourism, Climate Services, 22, 100215, https://doi.org/10.1016/j.cliser.2021.100215, 2021. a, b
Morin, S., François, H., Réveillet, M., Sauquet, E., Crochemore, L., Branger, F., Leblois, E., and Dumont, M.: Simulated hydrological effects of grooming and snowmaking in a ski resort on the local water balance [Data set], Zenodo [data set], https://doi.org/10.5281/zenodo.8408515, 2023. a
Rodrigues, C. M., Moreira, M., Guimarães, R. C., and Potes, M.: Reservoir evaporation in a Mediterranean climate: comparing direct methods in Alqueva Reservoir, Portugal, Hydrol. Earth Syst. Sci., 24, 5973–5984, https://doi.org/10.5194/hess-24-5973-2020, 2020. a
Scott, D., Knowles, N., and Steiger, R.: Is snowmaking climate change maladaptation?, J. Sustain. Tour., https://doi.org/10.1080/09669582.2022.2137729, in press, 2022. a
Spandre, P., Morin, S., Lafaysse, M., George-Marcelpoil, E., François, H., and Lejeune, Y.: Integration of snow management in a detailed snowpack model, Cold Reg. Sci. Technol., 125, 48–64, https://doi.org/10.1016/j.coldregions.2016.01.002, 2016a. a, b, c, d
Spandre, P., François, H., George-Marcelpoil, E., and Morin, S.: Panel based assessment of snow management operations in French ski resorts, Journal of Outdoor Recreation and Tourism, 16, 24–36, https://doi.org/10.1016/j.jort.2016.09.002, 2016b. a, b, c, d, e, f
Spandre, P., François, H., Thibert, E., Morin, S., and George-Marcelpoil, E.: Determination of snowmaking efficiency on a ski slope from observations and modelling of snowmaking events and seasonal snow accumulation, The Cryosphere, 11, 891–909, https://doi.org/10.5194/tc-11-891-2017, 2017. a, b
Spandre, P., François, H., Verfaillie, D., Lafaysse, M., Déqué, M., Eckert, N., George, E., and Morin, S.: Climate controls on snow reliability in French Alps ski resorts, Sci. Rep.-UK, 9, 8043, https://doi.org/10.1038/s41598-019-44068-8, 2019. a, b, c, d, e, f, g
Steiger, R., Scott, D., Abegg, B., Pons, M., and Aall, C.: A critical review of climate change risk for ski tourism, Curr. Issues Tour., 22, 1343–1379, https://doi.org/10.1080/13683500.2017.1410110, 2019. a, b, c
Steiger, R., Knowles, N., Pöll, K., and Rutty, M.: Impacts of climate change on mountain tourism: a review, J. Sustain. Tour., https://doi.org/10.1080/09669582.2022.2112204, in press, 2022. a
Thierion, C., Longuevergne, L., Habets, F., Ledoux, E., Ackerer, P., Majdalani, S., Leblois, E., Lecluse, S., Martin, E., Queguiner, S., and Viennot, P.: Assessing the water balance of the Upper Rhine Graben hydrosystem, J. Hydrol., 424–425, 68–83, https://doi.org/10.1016/j.jhydrol.2011.12.028, 2012. a
Tschanz, L., Arlot, M.-P., Philippe, F., Vidaud, L., Morin, S., Maldonado, E., George, E., and Spiegelberger, T.: A transdisciplinary method, knowledge model and management framework for climate change adaptation in mountain areas applied in the Vercors, France, Reg. Environ. Change, 22, 15, https://doi.org/10.1007/s10113-021-01862-3, 2022. a
UMR-CNRM: SURFEX Home Page, http://www.umr-cnrm.fr/surfex/ (last access: 25 September 2023), 2023. a
Vanat, L.: 2021 International Report on Snow and Mountain Tourism. Overview of the key industry figures for ski resorts, Tech. rep., http://www.vanat.ch/RM-world-report-2021.pdf (last access: 7 November 2023), 2021. a, b
Verfaillie, D., Déqué, M., Morin, S., and Lafaysse, M.: The method ADAMONT v1.0 for statistical adjustment of climate projections applicable to energy balance land surface models, Geosci. Model Dev., 10, 4257–4283, https://doi.org/10.5194/gmd-10-4257-2017, 2017. a
Verfaillie, D., Lafaysse, M., Déqué, M., Eckert, N., Lejeune, Y., and Morin, S.: Multi-component ensembles of future meteorological and natural snow conditions for 1500 m altitude in the Chartreuse mountain range, Northern French Alps, The Cryosphere, 12, 1249–1271, https://doi.org/10.5194/tc-12-1249-2018, 2018. a, b
Vernay, M., Lafaysse, M., Monteiro, D., Hagenmuller, P., Nheili, R., Samacoïts, R., Verfaillie, D., and Morin, S.: The S2M meteorological and snow cover reanalysis over the French mountainous areas: description and evaluation (1958–2021), Earth Syst. Sci. Data, 14, 1707–1733, https://doi.org/10.5194/essd-14-1707-2022, 2022. a, b
Vidal, J.-P., Martin, E., Franchistéguy, L., Baillon, M., and Soubeyroux, J.-M.: A 50 year high-resolution atmospheric reanalysis over France with the Safran system, Int. J. Climatol., 30, 1627–1644, https://doi.org/10.1002/joc.2003, 2010. a
Vionnet, V., Brun, E., Morin, S., Boone, A., Faroux, S., Le Moigne, P., Martin, E., and Willemet, J.-M.: The detailed snowpack scheme Crocus and its implementation in SURFEX v7.2, Geosci. Model Dev., 5, 773–791, https://doi.org/10.5194/gmd-5-773-2012, 2012. a
Wemple, B., Shanley, J., Denner, J., Ross, D., and Mills, K.: Hydrology and water quality in two mountain basins of the northeastern US: assessing baseline conditions and effects of ski area development, Hydrol. Process., 21, 1639–1650, https://doi.org/10.1002/hyp.6700, 2007. a