the Creative Commons Attribution 4.0 License.
the Creative Commons Attribution 4.0 License.
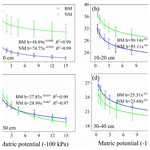
Biocrust-reduced soil water retention and soil infiltration in an alpine Kobresia meadow
Licong Dai
Ruiyu Fu
Xiaowei Guo
Yangong Du
Guangmin Cao
Huakun Zhou
Zhongmin Hu
Biocrust is a key component of ecosystems and plays a vital role in altering hydrological processes in terrestrial ecosystems. The impacts of biocrust on hydrological processes in arid and semi-arid ecosystems have been widely documented. However, the effects and mechanisms of biocrust on soil hydrological processes in alpine ecosystems are still poorly understood. In this study, we selected two meadow types from the northern Qinghai–Tibet Plateau: normal Kobresia meadow (NM) and biocrust meadow (BM). Both the soil hydrological and physicochemical properties were examined. We found that, in the 0–30 cm soil layer, soil water retention and soil water content in NM were higher than those in BM, whereas the 30–40 cm layer's soil water retention and soil water content in NM were lower than those in BM. The topsoil infiltration rate in BM was lower than that in NM. Furthermore, the physicochemical properties were different between NM and BM. The 0–10 cm soil layer's clay content in BM was 9 % higher than that in NM, whereas the 0–30 cm layer's soil capillary porosity in NM was higher than that in BM. In addition, the 0–20 cm layer's soil total nitrogen (TN) and soil organic matter (SOM) in NM were higher than those in BM, implying that the presence of biocrust may not favor the formation of soil nutrients owing to its lower soil microbial biomass carbon and microbial biomass nitrogen. Overall, soil water retention was determined by SOM by altering the soil capillary porosity and bulk density. Our findings suggest that the establishment of cyanobacteria crust biocrust may not improve soil water retention and infiltration, and the soil in cyanobacteria crust meadows could be more vulnerable to runoff generation and consequent soil erosion. These results provide a systematic and comprehensive understanding of the effects of biocrust on the soil hydrology of alpine ecosystems.
- Article
(3344 KB) - Full-text XML
- BibTeX
- EndNote
Biocrusts are composed of living non-vascular plants (mosses, lichen, and green algae) and microorganisms (such as cyanobacteria, fungi, and bacteria) associated with their bonding soil particles that occur in the uppermost few millimeters (Belnap et al., 2016; Sun et al., 2022). As a crucial part of the soil surface, biocrust plays a vital role in regulating biogeochemical processes, hydrology processes, and surface energy balances (H. Li et al., 2016), which can serve as “ecological engineers” in soil systems. However, to our knowledge, the controlling mechanism of biocrust on soil hydrological processes is still unclear. Most previous studies were conducted in arid and semi-arid ecosystems, such as the Tengger Desert, the Negev, and the Loess Plateau, with hydrological processes where plants are limited by soil moisture. Very few studies have focused on the role of biocrust in hydrological processes (i.e., soil water content, soil water retention, and soil infiltration) in alpine ecosystems where plants are limited by soil temperature. Thus, examining the impact of biocrust on hydrological processes could provide insight into water balances in alpine ecosystems and grassland management policies for maintaining the sustainability of meadow ecosystems.
The alpine meadow is an important ecosystem in the Qinghai–Tibet Plateau (QTP), which plays an important role in water retention (Dai et al., 2019), preventing soil erosion (Qian et al., 2021) and regulating energy exchange (Zhu et al., 2020) by altering soil surface features (i.e., roughness, soil texture, porosity, and aggregation) (H. Li et al., 2016; Xu et al., 2022). However, the formation of biocrust in alpine meadows is different from that in arid areas, where biocrust is formed from intensive land use such as overgrazing. Overgrazing could reduce vegetation coverage, thereby increasing the soil light condition, which favors the photosynthesis of cyanobacteria crust. A previous study found a good relationship between biocrust and vegetation coverage; i.e., the occurrence frequency of cyanobacteria crust increased with decreasing vegetation coverage owing to overgrazing (Y. Li et al., 2016). Moreover, the biocrust types vary with the succession stages of alpine meadows (Y. Li et al., 2016). For instance, as the degree of degradation increases, the moss-dominated crust is transformed into cyanobacteria-dominated crust, followed by lichen-dominated crust from Graminoid-dominated vegetation degradation to Kobresia humilis meadow (light degradation) and then to K. pygmaea meadow (moderate degradation) (H. Li et al., 2016). Thus, we suggest that the impact of biocrust on hydrologic processes in alpine meadows may differ from that in arid areas and vice versa.
To date, although numerous studies have pointed out that biocrust has substantial effects on soil water retention and soil moisture infiltration processes by altering soil microenvironments, such as soil roughness, soil porosity, and aggregation, no consensus has been reached. For instance, some studies have found that biocrust could increase soil water infiltration and reduce runoff by increasing soil porosity and aggregate stability compared with bare soil in cool desert ecosystems (Kidron and Benenson, 2014; Wei et al., 2015). In contrast, other studies reported that soil water infiltration was significantly reduced in crusted areas compared with non-crusted areas in arid ecosystems (Li et al., 2010). These discrepancies highlight the necessity to further explore the effects of biocrust on hydrological processes, such as exploring the specific hydrological processes by conducting soil infiltration experiments and soil water retention curve measurements. Furthermore, most previous studies were mainly conducted in arid and semi-arid ecosystems, and very few studies have focused on the effects of biocrust on the soil's hydrological processes in alpine ecosystems. Therefore, it is crucial to assess the role of biocrust in soil water retention and infiltration in alpine meadows.
To address these knowledge gaps, in this study, normal Kobresia meadow and biocrust meadow in the QTP were selected. Both soil and hydrological features were measured with the aim of exploring the role of biocrust in hydrological processes in alpine ecosystems. Specifically, the objectives of this study were to explore the effects of biocrust on soil–hydrological features in alpine ecosystems and to reveal how biocrust affects soil water retention by altering soil and vegetation properties. Our results could provide insights into the management of biocrust in alpine meadows.
2.1 Site description
The field test sites were located on the northeastern Qinghai–Tibet Plateau (37∘37′ N, 101∘19′ E) in Qinghai Province, China (Fig. 1a). The area has a continental plateau climate with a mean air temperature of −1.7 ∘C and a mean annual precipitation of approximately 562 mm (Dai et al., 2020). It should be noted that approximately 80 % of the precipitation occurs during the growing season (between May and September), and the other 20 % occurs during the non-growing season. The main vegetation type in this region is Kobresia meadow, which is dominated by Kobresia humilis (Fig. 1b). The soil type in the study area is silt loam according to the USDA soil taxonomy system of classification, with a soil thickness of approximately 60–80 cm. The pH and EC are 7.5 ms m−1 and 6.7, respectively, in the study area (Y. Li et al., 2016).
2.2 Experimental design and soil sampling
In August 2020, we chose two study sites on the northeastern Qinghai–Tibet Plateau to avoid pseudoreplication, and two types of soil surfaces were selected at each study site, i.e., normal Kobresia meadow (NM, Fig. 1b) and biocrust meadow (BM, Fig. 1c). To reduce the differences caused by spatial heterogeneity, BM was selected adjacent to NM to ensure the soil type and topographic condition were the same. The vegetation cover in BM is usually less than 20 %, with a thick turf but no litter layer in the topsoil, and the BM type is dominated by cyanobacteria crust (ca. 80 %) (Y. Li et al., 2016). In contrast, NM has a dense vegetation cover and is mainly dominated by Kobresia pygmaea, with average plant heights of 1–3 cm. Furthermore, a clear typical turf horizon and litter layer were observed within the topsoil in NM, i.e., the Afe horizon. BM had a higher root biomass than that of NM, owing to its thick turf (Table 1).
Table 1The soil saturated hydraulic conductivity (Ks), soil water content, and root density across the two surface soil types.
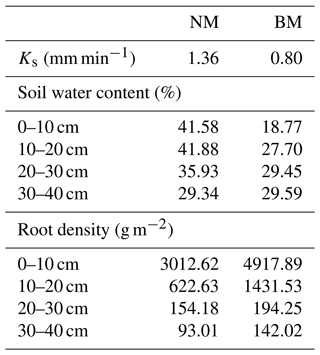
Note: NM is normal Kobresia meadow, and BM is biocrust meadow.
We obtained the disturbed soil samples (i.e., non-ring knife soil samples) in NM and BM. Four quadrats (1×1 m) were randomly selected for soil sampling with a depth of 10 cm in each treatment using an earth-boring auger and then brought back to the laboratory to measure and analyze soil organic matter (SOM), soil microbial biomass carbon (MBC), microbial biomass nitrogen (MBN), total carbon (TC), total nitrogen (TN), and soil texture (PSD). Undisturbed cylindrical ring samples (i.e., ring knife soil samples) were also obtained in each treatment to determine the soil bulk density (BD), soil porosity, and soil hydraulic properties (i.e., soil water retention and soil water supply capacity). The soil infiltration rates were measured using a double-ring infiltrometer for each treatment.
2.3 Laboratory measurements and analyses
First, the disturbed soil samples were sieved through 0.25 and 2 mm soil sieves to remove debris and roots for the analysis of soil properties. SOM was measured based on the Walkley–Black procedure (Nelson and Sommers, 1982), MBC and MBN were measured by the chloroform fumigation direct extraction method (Vance et al., 1987), and TC and TN were measured using an element analyzer (Elementar Vario EL III, Hanau, Germany). PSD was determined using a Mastersizer 2000 (Malvern Instruments, UK). BD was measured as the ratio of the oven-dried soil mass to the core volume (100 cm3). The soil total porosity, soil capillary porosity, and soil non-capillary porosity were measured using the following equations (Dai et al., 2020):
where TP, CP, and NCP represent the soil total porosity (%), soil capillary porosity (%), and soil non-capillary porosity (%), respectively; CWC represents the soil capillary water capacity; and ds is the soil particle density, which was assumed to be 2.65 g cm−3.
The soil water retention curves (SWRCs) were measured using a pressure plate apparatus (1500 F1, Soil Moisture Equipment Corp., SEC, USA), and the relationship between soil water content and matric potential was fitted by the Gardner model. The formula of the Gardner model is as follows (Gardner et al., 1970):
where h is the soil water content (%), θ is the matric potential (kPa), and A and B are the fitting parameters. Higher values of A⋅B and A indicate a higher soil water supply capacity and soil water retention capacity, respectively.
2.4 Statistical analysis
In this study, to compare the differences between BM and NM in soil water retention and soil properties, we conducted one-way analysis of variance (ANOVA) statistical tests to determine differences in plant and soil properties for the same soil layers between BM and NM, and a least-significant-difference test (P<0.05) was conducted when significant differences were detected by ANOVA. To explore the relationship between soil properties and soil water retention and for quantitative evaluation of the effects of soil properties on soil–soil water retention, Pearson's correlation and a variance partition in the analysis were used by R software version 3.4.3 with the hier.part and corrplot packages. Furthermore, structural equation modeling was used to examine the soil properties' direct and indirect effects on soil water retention.
3.1 Soil texture among two surface soil types
Sand content dominated the soil texture in the 0–40 cm soil layer across the two surface soil types (mean 61.69 %), followed by sand (mean 30.13 %) and clay (mean 8.18 %) (Fig. 2). Specifically, the 0–10 cm clay content in BM was 9 % higher than that in NM, whereas the 10–40 cm clay content in BM was 16 % lower than that in NM, especially for the 10–20 cm soil layer (P<0.001). In contrast, the 0–40 cm silt content in BM was higher than that in NM, especially for the 20–30 cm soil layer (P<0.05). However, no clear pattern was observed for the sand content between BM and NM. Overall, the 0–40 cm clay content (8.62 %) in NM was 11 % higher than that in BM (7.69 %), whereas in 0–40 cm, the silt content (61.24 %) in NM was nearly equal to that in BM (62.13 %).
3.2 Soil physicochemical properties among the two surface soil types
There were no significant differences for 0–40 cm BD, 0–40 cm TP, 0–40 cm CP, and 0–40 cm NCP (P>0.05) (Fig. 3), but the 0–20 cm BD in NM was 13 % lower than that of BM, and the TP and CP in NM were 7 % and 5 % higher than that of BM. No clear pattern was observed for NCP in NM and BM (Fig. 3). Furthermore, the 0–20 cm TN and SOM in NM were much higher than those in BM and reached a significant level at 0–10 cm (P>0.05), whereas the 30–40 cm TN and SOM in NM were lower than those in BM (Fig 3). Similarly, the 0–10 cm TC and the C:N ratio in NM were significantly higher than those in BM, whereas the 30–40 cm TC and the C:N ratio in NM were lower than those in BM (Fig. 3). Additionally, the 0–40 cm MBC and MBN in NM were higher than those in BM and reached a significant level at 0–10 cm (P<0.05) (Fig. 4).
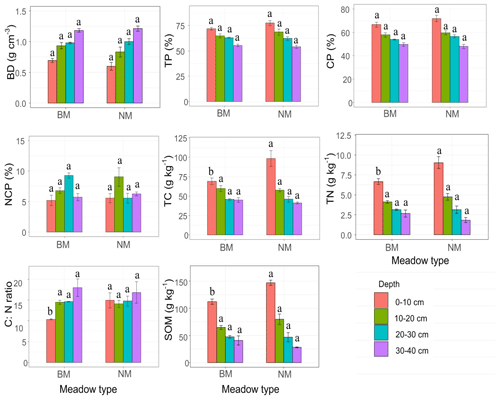
Figure 3The soil physicochemistry among two surface soil types. BD: soil bulk density, TP: soil total porosity, CP: soil capillary porosity, NCP: non-capillary porosity, TN: soil total nitrogen, TC: soil total carbon, C:N: soil C:N ratio, SOM: soil organic matter. The different letters mean significant differences (P<0.05) between normal Kobresia meadow and crust meadow in the same soil layer.
3.3 Soil hydrological processes among the two surface soil types
The soil hydrological processes varied between BM and NM (Fig. 5 and Table 1). Given that parameter A fitted by the Gardner model represents the soil water retention (a higher A value indicates higher soil water retention), the soil water content reduced with decreasing matric potential, reduced sharply at high matric potential, and remained stable at low matric potential (Fig. 5). The 0–30 cm layer's soil water content and soil water retention in NM were higher than those in BM, whereas the 30–40 cm layer's soil water content and soil water retention in NM were lower than those in BM (Table 1 and Fig. 6b). Similarly, the 0–10 and 20–30 cm soil water supply capacities (i.e., A⋅B fitted by the Gardner model) in NM were higher than those in BM, while the 10–20 and 30–40 cm soil water supply capacities in NM were lower than those in BM (Fig. 6a). Furthermore, the surface infiltration rate (Ks) in BM was significantly lower than that in NM (Table 1).
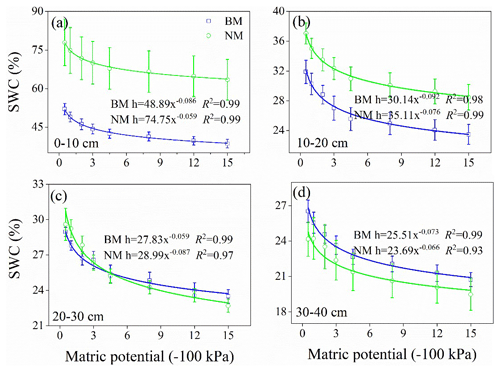
Figure 5Soil water retention curve of different soil layers – (a) 0–10 cm, (b) 10–20 cm, (c) 20–30 cm, and (d) 30–40 cm – among two surface soil types between soil water content (SWC) and matric potential. The soil water retention curve was fitted by the Gardner model (i.e., ), and A and B are the fitting parameters; a higher value of A indicated a higher soil-water-holding capacity.
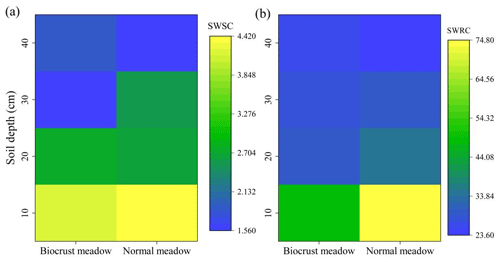
Figure 6Soil water supply capacity (SWSC) (a) and soil water retention capacity (SWRC) (b) of different soil layers across two surface soil types. The SWSC represents the A⋅B from the Gardner model. The SWRC represents the A from the Gardner model. Higher values of A⋅B and A indicated higher soil water supply capacity and soil water retention capacity, respectively.
3.4 Dominating factors affecting soil–soil water retention
Pearson correlation analysis showed that soil water retention was significantly negatively related to BD but significantly positively related to TP, CP, and SOM (Fig. 7a), whereas soil texture exerted weak soil water retention (Fig. 7a). Furthermore, the variance partition showed that SOM explained the greatest variability in soil–soil water retention (24.40 %), followed by CP (21.24 %), BD (18.22 %), and TP (18.22 %) (Fig. 8b), and structural equation modeling showed that the effect of SOM on soil water retention was achieved by altering CP and BD (Fig. 8).
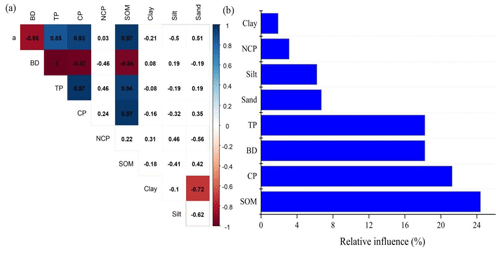
Figure 7Pearson correlation between soil water retention and soil properties (a) across two surface soil types and the relative influence of soil properties on soil water retention (b). Note: “*”, “”, and “” indicate significance at the 0.05, 0.01, and 0.001 levels, respectively. Note: a is the parameter fitted by the Gardner model, BD is the soil bulk density, TP is the soil total porosity, CP is the capillary porosity, NCP is the non-capillary porosity, and SOM is the soil organic matter.
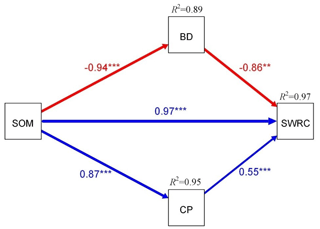
Figure 8Structural equation modeling of the direct and indirect effects of soil properties on the SWRC among two surface soil types. Standardized path coefficients, adjacent to arrows, are analogous to partial correlation coefficients and are indicative of the effect size of the relationship. Continuous blue and red lines represent positive and negative correlations, respectively. Model fit: Fisher. C=5.48, df=2, and P value = 0.065.
4.1 Effect of biocrust on soil properties
The effects of biocrust on soil properties have been widely explored in previous studies (Guo et al., 2008; Liu et al., 2019). Compared with non-biocrust and most studies conducted in arid regions, the presence of biocrust could improve soil aggregation and stability (Wu et al., 2020), increase soil fertility (Zhou et al., 2020), and reduce soil erosion (Chamizo et al., 2017). In this study, however, we found that the presence of cyanobacteria crust could improve topsoil texture compared with normal meadow but not that of deep soil. The 0–10 cm clay content in cyanobacteria crust meadow was higher than that in normal meadow, whereas the 10–40 cm clay content in cyanobacteria crust meadow was lower than that in normal meadow, which is in line with previous studies conducted in arid and semi-arid regions (H. Li et al., 2016; Wu et al., 2020). The higher clay content in cyanobacteria crust meadow was attributed to the exudation and cohesiveness of the biocrust, which promoted clay and silt formation and reduced sand content (Wang et al., 2021). Furthermore, we found that the 0–20 cm soil bulk density in normal meadow was lower than that in cyanobacteria crust meadow, thereby leading to a higher soil porosity and total capillary porosity in normal meadow. Such a higher soil capillary porosity in normal meadow was attributed to its higher soil organic matter content, which was also confirmed by the significant positive relationship between soil organic matter and soil capillary porosity (Fig. 7) because it has been well documented that higher soil organic matter could improve soil aggregation and stability and subsequently increase soil capillary porosity (Cui et al., 2021).
Moreover, most previous studies indicated that the presence of cyanobacteria crust can also improve soil nutrient conditions in the process of mobile sand fixation (Belnap et al., 2004; Guo et al., 2008; W. H. Li et al., 2005). However, we found that the presence of cyanobacteria crust reduces the 0–10 cm soil total carbon, total nitrogen, and C:N ratio compared with normal meadow, which is in contrast to most previous studies conducted in arid and semi-arid regions (Chamizo et al., 2012a; Zhao et al., 2010). A possible reason for these differences may be the environmental differences. It is well documented that the formation of biocrust is a changing process from simple to complex in its morphology, and the early cyanobacteria crust was formed only under favorable hydrothermal conditions such as temperature, soil water, solar radiation, and nutrient content (Belnap et al., 2004; X.-R. Li et al., 2005). For instance, biocrust is metabolically active when the external environment is wet, and its metabolically active environment is sensitive to temperature (Belnap et al., 2004; X.-R. Li et al., 2005); otherwise, the biocrust may choose to enter the dormant stage when the external environment is under unfavorable conditions. Therefore, compared to the higher soil temperatures in arid and semi-arid lands, the biocrust in alpine ecosystems may be in a dormant stage owing to its lower temperature and less available nutrients. Moreover, the biocrust in our study was mostly dominated by cyanobacteria crust, which was vulnerable to external disturbances such as grazing activity. Thus, the biocrust may choose dormancy when it is subjected to grazing pressure; this evidence was also confirmed by the significantly lower microbial soil carbon and microbial soil nitrogen content in cyanobacteria crust meadow compared with normal meadow (Fig. 4).
4.2 Effect of biocrust on soil hydrology and its underlying mechanisms
We found that soil water infiltration was greatly reduced in cyanobacteria crust meadow compared with that in normal meadow, which was consistent with the results of a previous study conducted in alpine meadows (Y. Li et al., 2016). However, this is in contrast to other studies conducted in cool desert ecosystems where biocrust increased soil water infiltration and reduced runoff by increasing soil porosity and aggregate stability compared with physical crusts and non-crusted bare soils (Kidron and Benenson, 2014; Wei et al., 2015). These discrepancies were associated with soil texture and biocrust developmental stage. In general, soil water infiltration in coarse-textured soils is higher than that in fine-textured soils owing to its large pores compared with the narrow pores in fine-textured soils, which reduces the movement of water into the soil (Belnap, 2006). However, we found that the establishment of cyanobacteria crust increased clay content and subsequently reduced soil macropores, which hindered soil water infiltration. Therefore, we conclude that the soil in cyanobacteria crust meadow may be more vulnerable to runoff generation and consequent soil erosion owing to its lower soil water infiltration and soil water retention capacity. On the other hand, biocrust can reduce available pore spaces for water to infiltrate by clogging the soil surface conductive pores owing to its higher water absorption and swelling of biocrust (Fischer et al., 2010), and it can consequently reduce soil infiltration. In addition, soil water infiltration was also affected by the developmental stage of the biocrust in homogeneous soil. A previous study found that soil hydraulic parameters differed significantly between cyanobacterial biocrust and moss biocrust (Wang et al., 2017). For instance, Chamizo et al. (2012b) reported that the incipient cyanobacterial crust had a lower soil infiltration rate than that of the cyanobacterial crust, whereas the dark-colored mosses' crust had a higher surface soil infiltration capacity by increasing macroporosity and unsaturated hydraulic conductivity in the grasslands (Jiang et al., 2018). In our study, the biocrust was dominated by incipient cyanobacterial crust, which had low biological activity and low porosity owing to the predominance of vesicle pores, thereby leading to a lower soil infiltration rate.
Furthermore, the soil water retention and soil water supply capacity varied significantly between the biocrust and normal meadows. We found that, in 0–10 cm, the soil water retention and soil water supply capacity in normal meadow were higher than those in cyanobacteria crust meadow, which was not in line with previous studies conducted in drylands in which biocrusts enhanced surface soil water retention capacity and water availability (Sun et al., 2022). We speculate that the lower soil water retention in cyanobacteria crust meadow was related to its lower soil organic matter; this evidence was also confirmed by the lower microbial biomass carbon (Fig. 4a). Furthermore, the structural equation model indicated that the effect of soil organic matter on water retention was mainly achieved by altering soil bulk density and soil porosity (Fig. 8) because higher soil organic matter could reduce soil bulk density and thereby increase soil porosity (Liu et al., 2019), leading to higher soil water retention. This result was also confirmed by the significant positive relationship between soil organic matter and soil water retention (Fig. 7), considering that soil organic matter was derived from vegetation litter and root biomass, whereas the vegetation litter in cyanobacteria crust meadow was lower than that in normal meadow owing to its lower aboveground biomass and vegetation coverage, ultimately resulting in lower soil organic matter in cyanobacteria crust meadow.
4.3 Implications for the effect of biocrust on alpine meadows
Grassland ecosystems cover more than 60 % of the QTP and provide important ecosystem services, such as biodiversity conservation, carbon storage, and water conservation (Qian et al., 2021). However, in recent decades, grasslands in the QTP have suffered from serious degradation due to increasing human activity (Dai et al., 2020). Biocrust is an important surface feature of degraded alpine meadows. It is acknowledged that biocrust has a positive effect on soil nutrient and soil water content retention in arid regions. In contrast, we found that the presence of cyanobacteria crust decreased soil water retention and soil infiltration rate, which did not improve water conservation in alpine meadows. Therefore, the soil in the cyanobacteria crust region may be more vulnerable to runoff generation and consequent soil erosion. Moreover, soil nutrients, such as SOM, TC, and TN, were reduced significantly in the cyanobacteria crust meadow, suggesting that the growth of vegetation in the cyanobacteria crust meadow may be limited by soil nutrients. Considering the negative effects of biocrust on alpine meadows, some steps should be taken to reduce the formation of cyanobacteria crust in degraded alpine meadows, such as reducing grazing intensity. Nevertheless, our study results were only obtained by conducting on a site scale, which may not sufficiently extrapolate the whole QTP owing to its high spatial heterogeneity. Thus, a larger scale or more study sites are necessary to draw a generalizability conclusion regarding the effects of biocrust on hydrological processes in alpine meadows of the QTP.
Soil hydrological processes were significantly affected by the establishment of cyanobacteria crust, and we found that cyanobacteria crust could reduce topsoil water and infiltrate topsoil, which suggested that the establishment of cyanobacteria crust may not favor soil hydrological processes in alpine meadows. Furthermore, the presence of cyanobacteria crust increased topsoil clay content, while the 0–30 cm layer's soil capillary porosity in NM was higher than that in BM, indicating that the presence of cyanobacteria crust reduced soil porosity and thereby reduced topsoil water infiltration. This suggested that the discrepancies in soil water retention and topsoil infiltration were close to physicochemical properties and that SOM plays a role in soil water retention by affecting CP and BD. Our study may be helpful for formulating reasonable management policies to maintain the sustainability of meadow ecosystems in the long run, especially under intense human activity and climate change in the QTP.
All the data needed to evaluate the conclusions in the paper are present in the paper and are contained in Figs. 2–6.
LD: investigation, data curation, writing – original draft, formal analysis. RF: investigation, data curation, writing – original draft, formal analysis, visualization. XG and ZH: investigation, data curation, project administration, supervision. YD: writing – original draft, review, editing. GC and HZ: conceptualization, methodology, funding acquisition, supervision.
The contact author has declared that none of the authors has any competing interests.
Publisher's note: Copernicus Publications remains neutral with regard to jurisdictional claims made in the text, published maps, institutional affiliations, or any other geographical representation in this paper. While Copernicus Publications makes every effort to include appropriate place names, the final responsibility lies with the authors.
We thank two anonymous reviewers for their constructive feedback, which helped improve the original paper.
This research has been supported by the Open Project of the Qinghai Provincial Key Laboratory of Restoration Ecology in Cold Regions (grant no. 2023-KF-04), the Natural Science Foundation of Qinghai (grant no. 2021-HZ-811), the Natural Science Foundation of Hainan (grant no. 422QN264), the Second Tibetan Plateau Scientific Expedition and Research Program (grant no. 2019QZKK0405), and start-up funding from Hainan University (grant no. KYQD(ZR)-22085).
This paper was edited by Patricia Saco and reviewed by two anonymous referees.
Belnap, J., Phillips, S. L., and Miller, M. E.: Response of desert biological soil crusts to alterations in precipitation frequency, Oecologia, 141, 306–316, https://doi.org/10.1007/s00442-003-1438-6, 2004.
Belnap, J.: The potential roles of biological soil crusts in dryland hydrologic cycles, Hydrol. Process., 20, 3159–3178, https://doi.org/10.1002/hyp.6325, 2006.
Belnap, J., Weber, B., and Büdel, B.: Biological Soil Crusts as an Organizing Principle in Drylands, in: Biological Soil Crusts: An Organizing Principle in Drylands, edited by: Weber, B., Büdel, B., and Belnap, J., Springer International Publishing, Cham, 3–13, https://doi.org/10.1007/978-3-319-30214-0_1, 2016.
Chamizo, S., Cantón, Y., Miralles, I., and Domingo, F.: Biological soil crust development affects physicochemical characteristics of soil surface in semiarid ecosystems, Soil Biol. Biochem., 49, 96–105, https://doi.org/10.1016/j.soilbio.2012.02.017, 2012a.
Chamizo, S., Cantón, Y., Lázaro, R., Solé-Benet, A., and Domingo, F.: Crust Composition and Disturbance Drive Infiltration Through Biological Soil Crusts in Semiarid Ecosystems, Ecosystems, 15, 148–161, https://doi.org/10.1007/s10021-011-9499-6, 2012b.
Chamizo, S., Rodríguez-Caballero, E., Román, J. R., and Cantón, Y.: Effects of biocrust on soil erosion and organic carbon losses under natural rainfall, Catena, 148, 117–125, https://doi.org/10.1016/j.catena.2016.06.017, 2017.
Cui, Z., Huang, Z., Luo, J., Qiu, K., López-Vicente, M., and Wu, G.-L.: Litter cover breaks soil water repellency of biocrusts, enhancing initial soil water infiltration and content in a semi-arid sandy land, Agr. Water Manage., 255, 107009, https://doi.org/10.1016/j.agwat.2021.107009, 2021.
Dai, L., Guo, X., Zhang, F., Du, Y., Ke, X., Li, Y., Cao, G., Li, Q., Lin, L., Shu, K., and Peng, C.: Seasonal dynamics and controls of deep soil water infiltration in the seasonally-frozen region of the Qinghai-Tibet plateau, J. Hydrol., 571, 740–748, https://doi.org/10.1016/j.jhydrol.2019.02.021, 2019.
Dai, L., Yuan, Y., Guo, X., Du, Y., Ke, X., Zhang, F., Li, Y., Li, Q., Lin, L., Zhou, H., and Cao, G.: Soil water retention in alpine meadows under different degradation stages on the northeastern Qinghai-Tibet Plateau, J. Hydrol., 590, 125397, https://doi.org/10.1016/j.jhydrol.2020.125397, 2020.
Fischer, T., Veste, M., Wiehe, W., and Lange, P.: Water repellency and pore clogging at early successional stages of microbiotic crusts on inland dunes, Brandenburg, NE Germany, Catena, 80, 47–52, https://doi.org/10.1016/j.catena.2009.08.009, 2010.
Gardner, W. R., Hillel, D., and Benyamini, Y.: Post-Irrigation Movement of Soil Water: 1. Redistribution, Water Resour. Res., 6, 851–861, https://doi.org/10.1029/WR006i003p00851, 1970.
Guo, Y., Zhao, H., Zuo, X., Drake, S., and Zhao, X.: Biological soil crust development and its topsoil properties in the process of dune stabilization, Inner Mongolia, China, Environ. Geol., 54, 653–662, https://doi.org/10.1007/s00254-007-1130-y, 2008.
Jiang, Z.-Y., Li, X.-Y., Wei, J.-Q., Chen, H.-Y., Li, Z.-C., Liu, L., and Hu, X.: Contrasting surface soil hydrology regulated by biological and physical soil crusts for patchy grass in the high-altitude alpine steppe ecosystem, Geoderma, 326, 201–209, https://doi.org/10.1016/j.geoderma.2018.04.009, 2018.
Kidron, G. J. and Benenson, I.: Biocrusts serve as biomarkers for the upper 30 cm soil water content, J. Hydrol., 509, 398–405, https://doi.org/10.1016/j.jhydrol.2013.11.041, 2014.
Li, H., Li, R., Rossi, F., Li, D., De Philippis, R., Hu, C., and Liu, Y.: Differentiation of microbial activity and functional diversity between various biocrust elements in a heterogeneous crustal community, Catena, 147, 138–145, https://doi.org/10.1016/j.catena.2016.07.008, 2016.
Li, W. H., Ren, T. R., Zhou, Z. B., and Liu, J. Z.: Study on the soil physicochemical characteristics of biological crust on sand dune surface in Gurbantünggtüt Desert, Xinjiang Region, J. Glaciol. Geocryol, 27, 619–627, https://doi.org/10.7522/j.issn.1000-0240.2005.0092, 2005.
Li, X.-R., Jia, X.-H., Long, L.-Q., and Zerbe, S.: Effects of Biological Soil Crusts on Seed Bank, Germination and Establishment of Two Annual Plant Species in the Tengger Desert (N China), Plant Soil, 277, 375–385, https://doi.org/10.1007/s11104-005-8162-4, 2005.
Li, X. R., Tian, F., Jia, R. L., Zhang, Z. S., and Liu, L. C.: Do biological soil crusts determine vegetation changes in sandy deserts? Implications for managing artificial vegetation, Hydrol. Process., 24, 3621–3630, https://doi.org/10.1002/hyp.7791, 2010.
Li, Y., Ouyang, J., Lin, L., Xu, X., Zhang, F., Du, Y., Liu, S., Cao, G., and Han, F.: Alterations to biological soil crusts with alpine meadow retrogressive succession affect seeds germination of three plant species, J. Mt. Sci., 13, 1995–2005, https://doi.org/10.1007/s11629-016-3917-3, 2016.
Liu, Y., Cui, Z., Huang, Z., Miao, H.-T., and Wu, G.-L.: The influence of litter crusts on soil properties and hydrological processes in a sandy ecosystem, Hydrol. Earth Syst. Sci., 23, 2481–2490, https://doi.org/10.5194/hess-23-2481-2019, 2019.
Nelson, D. W. and Sommers, L. E.: Total Carbon, Organic Carbon, and Organic Matter, in: Methods of Soil Analysis, John Wiley & Sons, Ltd, 539–579, https://doi.org/10.2134/agronmonogr9.2.2ed.c29, 1982.
Qian, D., Du, Y., Li, Q., Guo, X., and Cao, G.: Alpine grassland management based on ecosystem service relationships on the southern slopes of the Qilian Mountains, China, J. Environ. Manage., 288, 112447, https://doi.org/10.1016/j.jenvman.2021.112447, 2021.
Sun, F., Xiao, B., and Kidron, G. J.: Towards the influences of three types of biocrusts on soil water in drylands: Insights from horizontal infiltration and soil water retention, Geoderma, 428, 116136, https://doi.org/10.1016/j.geoderma.2022.116136, 2022.
Vance, E. D., Brookes, P. C., and Jenkinson, D. S.: An extraction method for measuring soil microbial biomass C, Soil Biol. Biochem., 19, 703–707, https://doi.org/10.1016/0038-0717(87)90052-6, 1987.
Wang, H., Zhang, G., Liu, F., Geng, R., and Wang, L.: Effects of biological crust coverage on soil hydraulic properties for the Loess Plateau of China, Hydrol. Process., 31, 3396–3406, https://doi.org/10.1002/hyp.11263, 2017.
Wang, J., Zhao, W., Wang, G., Yang, S., and Pereira, P.: Effects of long-term afforestationand natural grassland recovery on soil properties and quality in Loess Plateau (China), Sci. Total Environ., 770, 144833, https://doi.org/10.1016/j.scitotenv.2020.144833, 2021.
Wei, W., Yu, Y., and Chen, L.: Response of Surface Soil Hydrology to the Micro-Pattern of Bio-Crust in a Dry-Land Loess Environment, China, PLOS ONE, 10, e0133565, https://doi.org/10.1371/journal.pone.0133565, 2015.
Wu, G.-L., Zhang, M.-Q., Liu, Y., and López-Vicente, M.: Litter cover promotes biocrustdecomposition and surface soil functions in sandy ecosystem, Geoderma, 374, 114429, https://doi.org/10.1016/j.geoderma.2020.114429, 2020.
Xu, M., An, T., Zheng, Z., Zhang, T., Zhang, Y., and Yu, G.: Variability in evapotranspiration shifts from meteorological to biological control under wet versus drought conditions in an alpine meadow, J. Plant Ecol., 15, 921–932, https://doi.org/10.1093/jpe/rtac033, 2022.
Zhao, H.-L., Guo, Y.-R., Zhou, R.-L., and Drake, S.: Biological soil crust and surface soilproperties in different vegetation types of Horqin Sand Land, China, Catena, 82, 70–76, https://doi.org/10.1016/j.catena.2010.05.002, 2010.
Zhou, X., Ke, T., Li, S., Deng, S., An, X., Ma, X., De Philippis, R., and Chen, L.: Induced biological soil crusts and soil properties varied between slope aspect, slope gradient and plant canopy in the Hobq desert of China, Catena, 190, 104559, https://doi.org/10.1016/j.catena.2020.104559, 2020.
Zhu, J., Zhang, F., Li, H., He, H., Li, Y., Yang, Y., Zhang, G., Wang, C., and Luo, F.: Seasonal and Interannual Variations of CO2 Fluxes Over 10 Years in an Alpine Wetland on the Qinghai-Tibetan Plateau, J. Geophys. Res.-Biogeo., 125, e2020JG006011, https://doi.org/10.1029/2020JG006011, 2020.